There is a wide variety of substances that pollute the environment, including metals that have achieved high levels of concentration in many areas, creating a toxic effect on living organisms [1]. In recent years, a new scientific field was developed to reduce the cost of removal treatments of metals in solution: biosorption [2]. Early works showed that some biological materials can extract metal contaminants from water at very low cost, taking advantage of their selective physicochemical properties. Additional benefits of these treatments are its public acceptance and the possibility to be used with high performance under optimal and controlled conditions. New technologies for the recovery of these metals are in development [3].
Among the biopolymers with capture capability that can be used as biomass, the Exo-Polysaccharides (EPS) are biodegradable and with an interesting low-cost production [4]. There are a great number of microbial EPS that can interact with metal ions [5], [6]. These substances have a complex superficial chemistry with various functional groups, which together show different trends to join with other substances or ions [7], [8].
The classic characterization techniques for these systems are complex, laborious, with very high detection limits and low sensitivity. In addition, a considerable amount of sample to be analyzed is required. This has been a limitation to realize fast and precise experiences of transport in both, static and dynamic conditions. X-ray Micro-Fluorescence (XRµF) is an energy dispersive spectrometric technique with high resolution properties that allows studying dynamic systems. “In situ” XRµF was selected as the no-invasive technique to determine local concentrations in this study [9], [10].
Usually, to the design of a Plug Flow Reactor (PFR), the reaction rate is determined by tests on a Continuous Stirred-Tank Reactor (CSTR), these generate toxic effluents and also increase the cost of the design. In this work, an empirical expression (called “slip function”) in terms of the average concentration of the contaminant, was developed through the study of the transport behavior of CrVI into solutions of xanthan gum, as a model system. Chromium, one of the most common heavy metals contaminants [11] and, xanthan, a biodegradable and low-cost EPS, were chosen for this study [12].
The presentation of a more ecological way to design PFR for treatment of aqueous industrial effluents, and the proposal of the use of biodegradable and recyclable polymers of low cost, capable of retaining contaminants is the purpose of this work.
Experimental slip functions were used with laboratory PFR experiments planned in similar conditions, to obtain useful dimensionless parameters for the industrial design.
At the present time, the study of green chemical solutions for turnover times and subsequent recycling or reuse of both the polymeric material and the recovered metal are undertaken.
Standard Tritrisol Merck (1,000 mgL-1) solutions of Cr, and the same of Co (as internal standard) were used for the chromium quantification in SRμXRF. K2CrO4 was provided by Mallinckrodt.
Xanthan gum, provided by Sanofi Bio Industries (France), is produced by Xanthomonas campestris NRRL B-1459. It’s primary structure consists of regular repeating units; each unit contains five sugars: two glucose units, two mannose units, and one glucuronic acid unit. The main chain of xanthan gum is built up of β-D-glucose units linked through the 1- and 4-positions. A three-sugar side chain is linked to the 3-position of every other glucose residue in the main chain (Figure 1).
Xanthan gum monomer unit
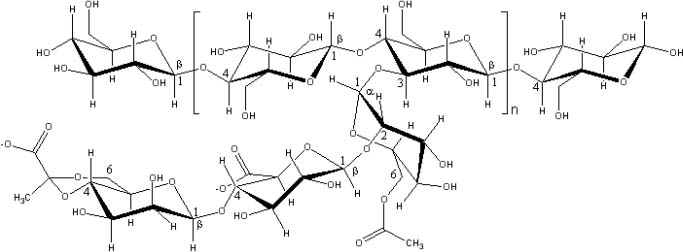
About half of the terminal D-mannose residues contain a pyruvic acid residue linked to the 4- and 6-positions. The non-terminal D-mannose unit in the side chain contains an acetyl group at position 6. Xanthan gum is a powder that dissolves in either hot or cold water to produce solutions with high viscosity at low concentration [13], [14].
To obtain the homogeneous solutions of this polymer, a measured mass of the solid was vigorously shaken during 24 h in high-purity water (18 MΩcm electrical resistivity). The polymeric system was purified by micro filtration and dialysis following a protocol especially tested and described in previous works [15]. The membranes used for dialysis were supplied by AH Thomas Co., (No. 4465-A2).
The intensity Ii, in arbitrary units (a.u.), detected by the device is directly proportional to the concentration C (molL-1) of the Cr (undifferentiating species) by a factor called sensitivity S of the measurement (Lmol-1). It was verified that the presence of any xanthan concentration does not influence the sensitivity (matrix effect) of the measurements. The utilization of the internal standard calibration technique removes any instrumental effect on the measurements. From the calibration curve using cobalt as an internal standard, the relative (to cobalt) sensitivity was obtained (Srel = 2,760 Lmol-1). In all determinations the intensity ratio ICr/ICo was calculated and the chromium concentration was obtained.
The microprobe installed at the LNLS X-Ray Fluorescence beam line station uses two types of set-ups depending on the required spatial resolution of the experiment. The set-up used in this work consists of a set of precision crossed slits giving the possibility of generating micro-beams sizes between 80 μm to 200 μm in both vertically and horizontally directions. The fluorescence photons are collected with the detector placed at 90º to the incident beam. The SRμXRF setup was designed for repeatability and high angular resolution as well as complete translational capacity. Translation stages were mechanically conducted to provide translations to the longitudinal axis. The originality of the measurements required the design of a special sample cell to measure transport properties into this type of systems. An improved design allows the study of two systems simultaneously (Figure 2).
Special sample cells for the scanning of polymeric systems, a design improved to study simultaneously two different systems
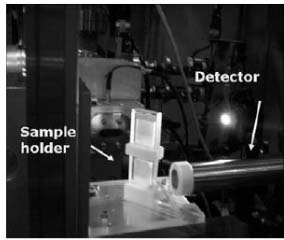
The experimental sample cells were designed to apply the semi-infinite free diffusion method for the determination of transport mass coefficients, similar to diffusion coefficients. In this method, the volume of solution begins at an interface and extends a length that can be considered infinite in terms of the experience: the thickness of the zone where diffusion occurs is much smaller than the total thickness of the sample. The transport of mass is a result of a pulse of concentration at the interface [16].
The main purpose of the experiences was to obtain the concentration profiles for the ions diffusing through the polymeric systems as a function of time t and as a function of distance x from the start point (x = 0).
For each experiment, 10 μL of a heavy metal salt solution were deposited in the bottom container of the acrylic cell. The upper container was filled with the polymer solution. After assembling the cell (t = 0), it was placed in a support of the XRF line. The cell moved through the x axis, perpendicular to the beam, by means of a motorized stage, so that specific regions on the surface were irradiated. All measurements were performed in air-conditioned rooms (25 ± 2 ºC).
For a complete study, samples were scanned six times requiring approximately fifteen minutes each and a set of XRF spectra with an acquisition time of 40 s each was obtained. Figure 3 shows a typical spectrum from xanthan solution with chromium. The total time for the diffusion into the cell was four hours and each experiment gave a collection of hundreds of spectra. The spectra were analyzed with the software package QXAS-AXIL2005, from IAEA.
SRµXRF spectrum obtained from one point in the cell with xanthan solution with chromium
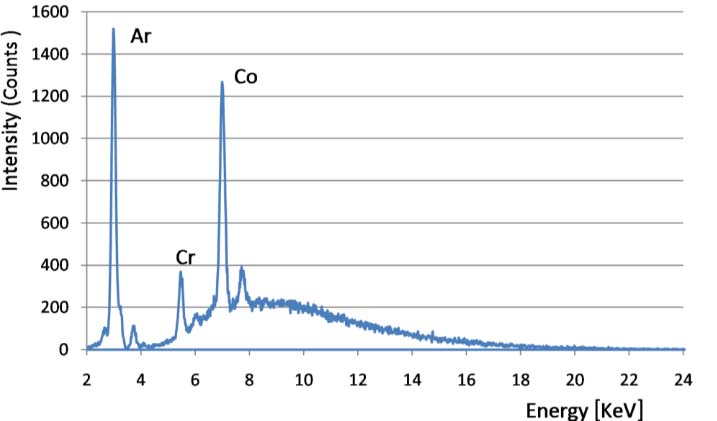
CrVI concentration at different times and positions, obtained during a SRµXRF scanner of the sample cell with 7,000 mgL-1 xanthan solution
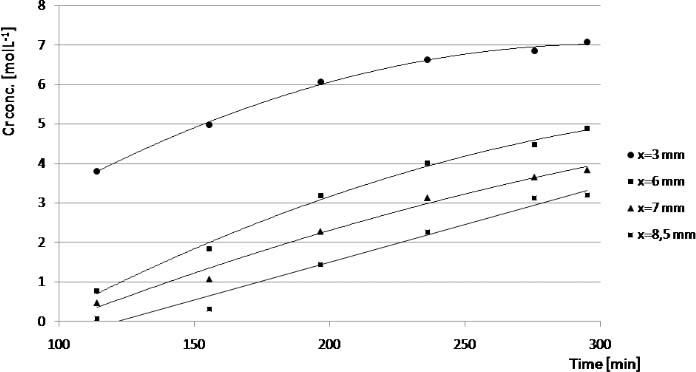
where αs and βs are empirical parameters depending on the ion range of concentration and polymer concentration. The values for CrVI in 7,000 mgL-1 xantan obtained by fitting (R2 = 0.976) are shown in Table 1.
Experimental slip function coefficients and design dimensionless parameters obtained for the retention of CrVI in 7,000 mgL-1 xanthan solution
Filler solution | Slip function coefficients | Dimensionless parameters | ||
---|---|---|---|---|
Xanthan [mgL-1] | αs [Lmol-1min-1] | βs [min-1] | ϴs | ks |
7,000 | -2.82 × 10-3 | 1.08 × 10-2 | 9.20 × 10-1 | 2.79 × 10-1 |
Obtained by this way, the called slip function fs is related with the accumulation rate of the ion in this system (Figure 5). fs necessarily vary with the nature of the ion, the type and concentration of the polymer and the temperature. Conceptually, fs is a measure of the ability of the ion to stay or be retained in that system.
Slip functions obtained from chromium moving in xanthan solutions calculated from experimental data
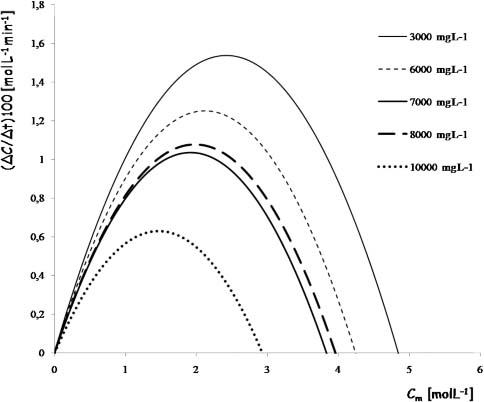
Higher ion rates are in dilute systems and lower in concentrated systems. However an anomaly was observed: The fs for xanthan 7,000 mgL-1 falls below that of 8,000 mgL-1. This can be explained in terms of the number of available active sites, due to easier access within a less concentrated solution. That is, despite there being a greater number of active sites as there are more macromolecules, not all of these sites are available because this high concentration impedes access. And then at 10,000 mgL-1, a gel is formed that retains ions also by steric effects. The objective of this work is to use solutions rather than gels, by the advantages offered as its recyclability and the lower operating costs of the equipment involved (eg. lower pressure of work). The optimal range for our design will then be about 7,000 mgL-1 of xanthan, that retains the advantages of being a solution and presents a maximum of active sites.
In this work the experimental slip functions are employed as the retention rate functions as is shown in (eq. 3):
The ion residence time τs using the slip functions, in a reactor of volume V was calculated as in shown in (eq. 4):
Using the ratio of the experimental replacement time tre and the residence time, fixed in this experience at 60 min (Figure 6), a dimensionless parameter, θs, is generated (eq. 5). Experimental value is shown in Table 1.
Output Cr concentration from the laboratory PFR reactor as a function of the time
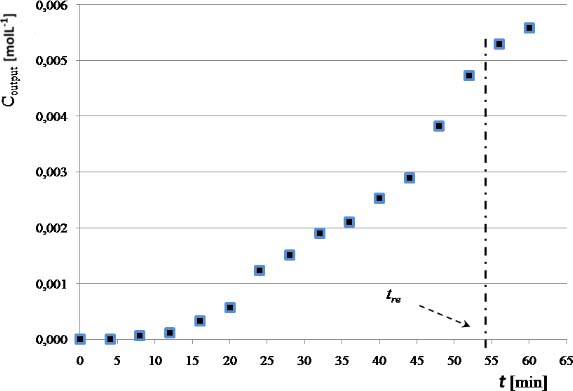
To confirm the independence of θs of the conditions of this particular experience, several similar experiments were conducted with different residence times. It was noted that θs value remains constant by doubling or by taking half the value of the volumetric flow through the reactor. Thus, a design parameter was obtained that indicates the number of residence times that any reactor may comply before that its filler should be replaced, for the given operating conditions (range of CrVI concentrations, xanthan concentration and temperature).
To make the change of scale to the industrial reactor, other dimensionless parameter was generated as the ratio of the replacement time value and the value of the residence time calculated (eq. 6). Experimental value is shown in Table 1.
The values found for this dimensionless number in experiences with different systems indicate that this is characteristic of the nature of the polymer, but does not depend on its concentration neither of the nature or concentration of the ion.
This parameter is used in the design as follows: τs is calculated by using the slip function integrated between the concentrations of interest and with ks, the replacement time is obtained. With this value, the θs parameter is then used to obtain the residence time of the industrial reactor and to calculate its volume for a known flow. The volume thus determined maximizes the design with regard to the efficiency of the filler that was tested in a microcell, with only a few milliliters of contaminant solution.
It has been shown that the slip functions can be used as retention rate equation in reactor design. The defined dimensionless parameters (ϴs, ks) link between experimental values obtained in a laboratory reactor tre, and the values calculated using the slip functions τs. It has been demonstrated from these experiments that the obtained values of θs can be used to estimate the time of replacement of the industrial prototype.
The presented methodology involves fewer quantities of drugs, and is faster than classical. Experiments with the laboratory PFR were essential to obtain the parameters required for the design, but, as with any engineering problem, the application will encounter compromise situations that should be resolved, involving costs and operation.
Heavy metals and the biopolymer can be recycled. When the polymer is out of stock, it can be easily cleaned and eliminated by biodegradation in digesters, thus closing the cycle of providing energy and fertilizer use.
This design method, which was described for the retention of contaminants by polymer solutions, could be applied to any other type of retention media.
In the near future when the constraints imposed by the environment were intensified and enforced, surely there will be more than one application for this kind of environmentally friendly industrial processes [18]-[20].
C | Concentration | [molL-1] |
C0 | Input concentration | [molL-1] |
Cv | Output concentration | [molL-1] |
Cm | Average concentration | [molL-1] |
F | Flow | [Ls-1] |
T | Time | [min] |
V | Volume | [L] |
αs | coefficient of the quadratic term of the slip function | [Lmol-1min-1] |
βs | coefficient of the lineal term of the slip function | [min-1] |
fs | slip function | [mols-1] |
ks | dimensionless design parameter | [-] |
θs | dimensionless design parameter | [-] |
τ | residence time | [s] |
tre | filler replacement time | [min] |
νR | retention rate | [mols-1] |
Heavy Metals: Toxicity and Removal by Biosorption ,Environmental Chemistry for a Sustainable World , Vol. Vol 2 , 2012, https://doi.org/10.1007/978-94-007-2439-6_10
, Biosorption of Heavy Metals - An Overview ,Indian Journal of Biotechnology , Vol. Vol. 7 , :159-1692008
, Biosorption Metals ,Encyclopedia of Industrial Biotechnology , 2010, https://doi.org/10.1002/9780470054581.eib166
, Chelating Properties of Extracellular Polysaccharides from Chlorella spp ,Appl. Environ. Microb , Vol. Vol. 53 , :2953-29561987
, Applications of Extracellular Microbial Polysaccharide-polyelectrolytes: Review of Literature, Including Patents ,J. Polym. Sci. Polym. Symp , Vol. Vol. 45 , :209-2271974, https://doi.org/10.1002/polc.5070450118
, Estudio del transporte de masa en sistemas poliméricos blandos (Mass Transport Studies in Soft Polymeric Systems) ,PhD Thesis , 2003
, Interactions between Metal Ions and Capsular Polymers ,Metal Ions and Bacteria , 325-3571989
, Trace Metal in Soil by Bacterial Polymers ,Environ. Health Perp , Vol. Vol. 103 , :53-581995, https://doi.org/10.1289/ehp.95103s153
, Ionic Transport into Soft Polymeric Systems by Micro X Ray Fluorescence ,LNLS Activity Report 2007 , 2008
, Preliminary Classification of Polymers by using Total-Reflection X-ray Fluorescence Spectra ,Spectrochim. Acta B , Vol. Vol. 59 (No. 8), :1189-11922004, https://doi.org/10.1016/j.sab.2004.04.012
, Mechanisms of Chromium Toxicity, Carcinogenicity and Allergenicity: Review of the Literature from 1985 to 2000 ,Human & Experimental Toxicology , Vol. Vol. 20 (No. 9), :439-512001, https://doi.org/10.1191/096032701682693062
, Biodegradation of Xanthan Gum by Bacillus sp ,Appl. Environ. Microbiol , Vol. Vol. 44 (No. 1), :5-111982
, Structure of the Extracellular Polysaccharide from Xanthomonas Campestris ,Carbohydr. Res , Vol. Vol. 45 , :275-2821975, https://doi.org/10.1016/S0008-6215(00)85885-1
, Polysaccharide (Xanthan) of Xanthomonas Campestris NRRL B-1459: Procedures for Culture Maintenance and Polysaccharide Production, Purification, and Analysis, ARS-NC-51 , 1-141976
, Low Impurities in Scleroglucan Systems: Removal Processes Control by Total Reflection X-ray Fluorescence ,J. Trace Microprobe Techn , Vol. Vol. 20 , :283-2922002, https://doi.org/10.1081/TMA-120003730
, , Diffusion: Mass Transfer in Fluid Systems , 2009, https://doi.org/10.1017/CBO9780511805134
, , Standard Methods for the Examination of Water & Wastewater , 2012
, Decreto 831/93, Residuos peligrosos, Reglamentación de la Ley 24051 (Hazardous waste, Regulation of Law 24051) ,Boletín Oficial de la República Argentina , 4-221993
, Treatment of Chromium (VI) Containing Aqueous Effluent of Tanneries and Electroplating Units by Membrane Process, 227-1 ,Proceedings of the International Conference on Environmental Pollution and Remediatio , 227-1-227-820112011
, , Producción limpia en la industria de la curtiembre (Cleaner production in tanning industry) , 2009
,