Numerous Architecture, Engineering, and Construction (AEC) industry-related environmental problems like scarcity of resources due to shrinking or even exhausted sources of raw materials and improper waste management lead to the avoidable need for waste deposits (landfill). The AEC industry is responsible for 60% of global raw material extractions [1] – other sources say 40–50% [2] – and the demand has tripled in the period 1970–2017 [3]. A significant proportion of waste and 40–50% CO2 emissions are AEC-related [4], [5]. In Austria, construction and demolition waste (CDW) accounted for about 17% of total waste generation in 2015 (60% if excavation material is added – in general, it is attributable to construction activities). Still, there is a local divergence here [6]. EU-wide, the share of CDW in total waste generation is about 33% [7]. However, it is difficult to compare the data of the individual member states. Often the ratio of CDW and required building material is 1:10, but in some regions (primarily cities), the ratio is significantly lower [8]. That allows the substitution of a higher proportion of primary resources. Comparison of the waste masses and the consumption of building materials show that the ratio of 1:10 is a realistic reference value for Austria Klicken oder tippen Sie hier, um Text einzugeben.Klicken oder tippen Sie hier, um Text einzugeben.. Secondary raw materials obtained through recycling can only cover part of the total demand. Although secondary raw materials do not utterly solve the resource problem, they can contribute significantly [10]. The sheer mass of mineral CDW has excellent potential for reducing both raw material extraction and the demand for waste deposit sites [11]. Even though by 2020, 70% of the building waste must be recycled or reused [12], resource efficiency and recyclability are often no design criteria in planning. They are usually not focused on during the deconstruction process [13].
Another AEC-related problem is high energy consumption. Buildings are responsible for 40% of the total energy consumption EU-wide [5], 5–10% of which is spent on the production of building materials, and the rest on operation and construction [14]. So, the importance of responsible material consumption in the AEC industry is apparent, especially concerning its impact on the environment and sustainability in a broader context.
Geissdoerfer et al. give an excellent overview of different definitions and the heritage of sustainability and Circular Economy (CE) [15]. In the context of the present study, the term sustainability is interpreted as a development that meets the needs of the present without compromising the ability of future generations to meet their own needs [16]. And that of CE as a regenerative system in which resource input and waste, emission, and energy leakage are minimised by slowing, closing, and narrowing material and energy loops [15]. It signifies that the implementation of CE measures must also consider environmental impacts due to recycling and reuse processes. Thus, sustainable CE and material efficiency should always be accompanied by a life cycle assessment (LCA), incorporating energy demand and environmental impact. One should also consider costs in a holistic approach because recycling needs to be economically beneficial. Otherwise, it will not be practiced [13].
The United Nations (UN) tries to target these problems, among others, with the UN Sustainable Development Goals (SDG) [17]. These consider sustainability in a holistic context in the three pillars of sustainability: ecological, economic, and social [18]. Van Soest et al. analysed the interaction and effects of all seventeen SDGs on one another, using expert interviews and Integrated Assessment Models (a method to analyse human-environment interaction) [19]. The results show middle to the strong interaction of the SDGs 9,11,12, and 13. A similar connection is observed by Bleischwitz et al., who present a proposal for a resource nexus focusing on SDG 2, 6, 7, 11, and 12, including energy, water, food, land, and material and their interconnection [20]. They analyse the affected SDGs by these resources and their interconnections and show which other SDGs are affected. For example, not only SDGs 2 and 11 are affected by the use of land for material production, but also SDGs 13 and 15. The importance of materials in relation to cities and industry is emphasised. The main difference is that one study focuses on the interaction of different SDGs to one another, and the other one on the influence of the use of different resources on SDGs. Another study conducted to connect different material types (e.g., green building material, local building material, low embodied energy building material, etc.) to the influence of the SDGs is seen in [21]. Most influences on the Goals identified were 3, 7, 9, 11, 12, 13, and 15. The influence on the SDGs 9, 11,12, and 13 was positive, except for the impact of local building material on the industry and the indicator 11.1 (access to safe and affordable housing and basic services) by some material categories.
However, one should understand sustainability in this paper as focusing on specific aspects of environmental sustainability and the associated costs (but no focus on economic sustainability). For example, elements such as biodiversity, water consumption, and land consumption are not considered, but the scope of consideration could subsequently be extended to include these aspects. Therefore, the focus is on sustainable material use with some environmental consequences of material consumption, aiming for CE. The SDGs 9 (Industry, Innovation, and Infrastructure), 11 (Sustainable Cities and Communities), 12 (Responsible Consumption and Production), and 13 (Climate Action) are minorly or significantly influenced by typical building behaviour or influence the building sector. If one wants SDG 11 and SDG 13, one needs SDG 12, and for successful implementation, SDG 9 is necessary.
Knowledge of the material composition is needed to target sustainable material use. Kleemann et al. developed a methodology to explore the material composition [22]. The building stock is determined by collecting and analysing existing documents, investigating pollutants, and determining the built-in materials through on-site inspection. Building Information Modelling (BIM) is a suitable tool for building data storage. One can see BIM as a method to generate a digital model of buildings, including relevant data throughout the life cycle [23]. A distinction must be made between geometric and non-geometric (alphanumeric) parameters. Geometric parameters control the dimensions of the elements, and alphanumeric parameters assign cost parameters, LCA data, building physics information, etc., to them. It is already possible to generate semi-automated Material Passports (MPs) for material documentation and improve the buildings’ recyclability through material documentation using BIM and linking environmental data with the materials [24].
Further tools like LCA or recyclability assessment can be implemented in the process. The MP used by Honic et al. for example, calculates the Eco indicators: Global Warming Potential (GWP), Acidification Potential (AP), and Primary Energy Input (PEI) as well as potential recycling material, which can be used as secondary raw material and therefore, reduce the raw material extraction [24]. This assessment can be semi-automated, linking an architectural BIM model with (material) data. There are many other approaches to use BIM for sustainable reasons.
However, the required flexibility between disciplines in AEC is not supported in the current most widely used open exchange schema Industry Foundation Classes (IFC). This taxonomy model used to define exchange requirements has an excellent comprehensive set of terms covering building elements and their attributes. This schema does not cover all requirements of all participants in AEC, especially life cycle experts. For example, ecological parameters are not considered or only considered to a small extent [25]. Akbarieh et al. thus also conclude that the End-of-Life phase is not sufficiently considered in any BIM software [26].
Further, Akinade et al. conclude from the analysis of different CDW management tools and stakeholder interviews that they are not BIM-compatible, and data for LCA on CDW is not available [27]. One solution could be BIMRel, which provides a Framework for embedding product information in BIM [28]. Producers provide a declaration of performance in a cloud-based Structured Query Language (SQL) database, which enables a standardised, phase-dependent output of product information. Implementing such a scheme to data exchange formats offers a solution for the above problem. Akanbi et al. estimate the amount of recyclable and reusable material with the BIM-based whole-life Performance Estimator by using a Weibull reliability distribution for manufactured products to consider the probability of failure over the life cycle [29]. Essentials into promoting CE in the AEC industry are knowledge on buildings' material compositions, what to do with the building waste, and how to communicate these materials for further use. Copeland and Bilec suggest a solution using BIM and RFID (radio frequency identification) [30]. By linking the RFID chips to BIM elements, knowledge about the material composition is created, analysed before deconstruction, and tracked with the help of the RFID chips after deconstruction and used for mediation.
For example, other existing mediation platforms include Recycling-Börse Bau, which allows placing advertisements in an online platform as offers and inquiries [31]. Predominantly mediated are mineral construction waste and already processed mineral recycling building materials. Harvest Map Austria [32] and Harvest Map Netherlands [33] provide materials suitable for reuse, ranging from washbasins to flooring to worn bricks.
Different research projects established the basis for the knowledge of the material stock of cities. For example, SCI-BIM aims to increase resource and energy efficiency by coupling different digital technologies and methods for data acquisition and as-built BIM using a gamification approach. The suitability of ground radar for material data acquisition is associated with laser scanning technology for geometry acquisition [34]. Madaster is a Swiss building raw material register, in which the MPs of the registered objects serve as a basis for urban mining processes, showing circular and financial potential and the possibility of component reuse [35]. Growing in Circles: the Global Initiative for Resource-Efficient Cities (GI-REC) program launched by the UN seeks to apply integrated approaches and analyses such as urban metabolism to urban planning and management. And it aims to engage industry, policy, and research representatives. Growing in Circles summarises the GI-REC experience [36].
Another important and extensively studied topic is waste management and built environment, which focuses on different fields, such as technical, management, or environmental impact. Even though holistic approaches, looking at the whole supply chain and including cost and environmental impact aspects are available, there is a lack of implementation in practice. Furthermore, there is a lack of data for the LCA of CDW [13]. This problem is addressed by Mortaheb and Mahpour, for example, who developed an Integrated Construction Waste Management in their work, which considers all phases of a building and shows recommendations for the individual measures to avoid waste [37]. Yeheyis et al. address the issue of linking all three pillars of sustainability in the phases of planning, construction, and deconstruction. The study presents a Framework for promoting reduction, reuse, and recycling related to CDW and the construction and demolition waste LCA-based sustainability index [38].
In a study that examined different practices in different EU member states to identify best practices in waste management, a consideration if the entire value chain of the construction sector is recommended [39]. The link between energy use, environmental impacts, and costs is poorly understood. Tomic and Schneider examine different waste recovery routes, with the associated costs (investment, operation, revenues, and taxes), the associated energy recovery, and material conservation [40]. Although the study deals with municipal waste, work with a similar thrust is also needed for the AEC industry. A study from Australia examines, among other things, the economic differences of selected materials in different material flows and shows that the economic viability of recycling vs. landfilling also depends on spatial factors. Furthermore, the energy demand for collection and treatment is investigated, but no emissions are included [41].
This paper aims to develop a Framework to assess the CE potential of the AEC industry. The focus here is on demolition buildings and construction waste (as part of AEC), comparing the ecological impact with the economic and technical effort required of recycling and reusing processes (see section Literature Review). It is an integrated approach linking the legal basis with environmental impacts and costs due to material use and waste management (collection, treatment, and processing for reuse). The Framework is developed by reviewing assessment methods and comparing the essential parameters of material sustainability, including material efficiency, environmental impact, and End-of-Life performance, as the basis for the recycling process and creation of closed material loops. The procedures should be as simple as possible and as complex as necessary to make them applicable in practice on a broad scale.
The conceptual development for evaluating the CE-potential of materials embedded in buildings is based on an extensive literature review, upon which relevant parameters and required evaluation methods were identified and compiled into an extensive conceptual Framework. Figure 1 presents the overall research methodology, including the literature review and scope of the paper.
Overview of the research methodology and scope of the paper
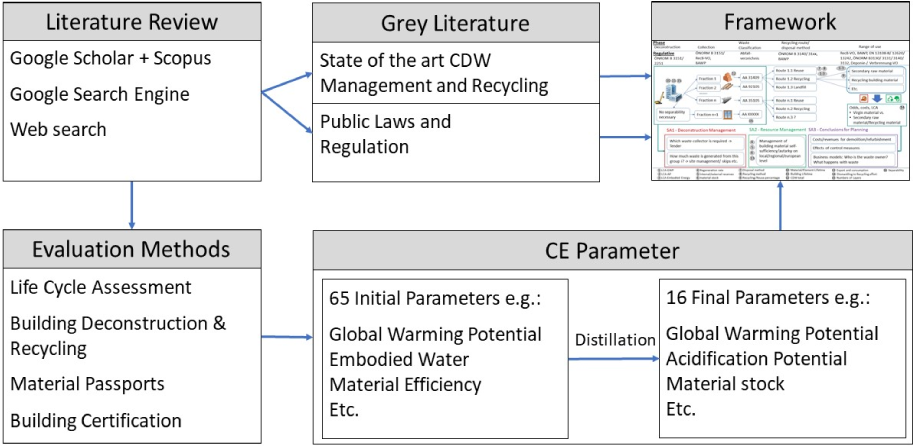
The review was conducted extensively because sustainable CE in the AEC industry is influenced by different fields, such as waste management, architecture, engineering, and environmental assessment. Two search engines Google Scholar and Scopus, were used to obtain significant literature. The search was further complemented by grey literature and web research, particularly useful for regulations and building certification. To get a general overview of CDW management and a more detailed one of existing assessments, the literature review was conducted for the following fields:
LCA for Buildings for environmental effects according to material use,
Deconstruction and recycling for estimation of waste and recycling material as well as disassembly methods,
Building certification systems,
MPs for material documentation,
Review of Regulations and Public Policies.
BIM-based approaches were observed in all of the literature. The literature found was then analysed and divided into three categories, a) important insights into relevant topics, b) calculation and evaluation approaches for assessing the sustainability of building materials (especially in the End-of-Life phase), c) laws, and law-like texts.
Following this, a parameter study of the literature of category b was conducted. Input variables required for the assessments were extracted, listed, analysed, and compared. The list of parameters obtained was subjected to a distillation process to eliminate irrelevant parameters or combine similar parameters. This process reduced the parameters obtained to about a quarter. In the next step, a conceptual Framework proposal (based on the findings from the literature research and the final parameter setting) was developed to evaluate the sustainable CE potential (ecological-economic-technical proportionality), as presented in the section Literature Review. In a final step, possible fields of application were linked to the evaluation Framework, and research areas were sought which are necessary for implementation and realisation.
The EU and Austria are making great efforts to tackle the resource problem. The most important regulations relevant to waste management are listed here in excerpts. The basis for this is the waste Framework directive (WFD) 2008, which defines the term waste and regulates its handling and treatment [12]. Since 2014, the Resource Efficiency in the Building Sector Report provides measures to increase material efficiency [14], and CE-Principles, which focuses on non-destructive disassembly [42]. The national implementation of the WFD is the waste management law 2002 (AWG2002) [43]. The waste loads to be separated, the handling of these, and the processing and possible fields of application are regulated in the Recycling Building Materials Directive (RecBVO) [44]. The directive refers to the standard ÖNORM B3151, which thus also has the character of law [45]. Therefore, selective deconstruction is the standard demolition method in Austria.
Depending on the existing masses and volumes of the demolition objects, the selective demolition method and a pollutant and contaminant investigation are carried out following ÖNORM B 3151 (for 3500 m³ built volume) and ÖNORM EN ISO 16000-32 [46]. Based on the pollutant and contaminant investigation, a demolition concept is drawn up, which, however, is not updated during the demolition process and has no binding character.
According to AWG2002: Recyclable materials need to be recovered if this is ecologically expedient and technically possible and if it does not involve disproportionate costs (in German). This requires data and information on demolition, processing and recycling costs, and the resulting environmental impacts. These are also reflected in several assessment methods, such as ecological impact in LCA; thereby, phases C (disposal and crediting) and D (off-system loads) of Environmental Product Declarations (EPD) are of relevance or the material-recycling assessment in MPs [24]. To carry out a holistic assessment, one must bring together available assessments that take sub-areas of the technical-economical-ecological proportionality into account.
The deconstruction of the Coca-Cola plant in Vienna is considered the first Austrian deconstruction project in the large-volume construction sector [47]. Through environmentally friendly and non-destructive dismantling, a turnover of 100,000 € was generated on behalf of a consortium of building owners consisting of eight property developers. More than 5,000 roof panels were provided as thermal insulation for reuse on-site. Likewise, 3000 m² of an extensive green roof could be removed and reused. With a land area of 240 ha, the Urban Lakeside Aspern is one of the most significant international urban development projects [10]. The project development company has been supporting Urban Mining since the beginning of the project with a specially founded logistics subsidiary. This case study shows how more than 1 million t of material could be processed and used locally in constructing the first 3,000 flats by balancing the masses of excavated material from different building sites. A performance specification to determine additional and reduced costs showed that economic advantages (varying from location to location) are achievable despite the control and quality assurance efforts. These economic advantages result from transport savings, availability, and presence of raw materials on-site, and the saving of the purchase of these.
In addition to using less material or using materials for longer, another possibility to make material use and the circular economy more sustainable is a change in the producing/processing technology. Shen et al., for example, identify the technology- and production-driven mitigation potential of up to 50% in CO2 emissions from cement production in China, in addition to the reduction of energy demand [48]. These include, for example, chlorine substitution, fossil fuel substitution, waste heat recovery, etc. Carbon capture and storage is another example. The networking of different industries and the direct use and processing of carbon, as attempted in the C2PAT (Carbon 2 Product Austria) pilot project, could contribute to climate neutrality and CE of the carbon cycle. The project involves the construction of a full-scale plant that collects CO2 from cement production, converts renewable-generated hydrogen into hydrocarbons, which are then fed into a petroleum refinery and finally processed into renewable fuels and renewable-based plastics [49], [50]. However, too much focus on cement (part of concrete production) can lead to neglect of other (possibly critical) materials and related factors (health, availability, etc.) [13].
To assess sustainable aspects of buildings, methods considering a broad scope of criteria and parameters are available. These include assessing the End-of-Life Performance and ease of disassembly [51]–[53], LCA with different methods [54], [55], and material documentation in the form of MPs considering different additional aspects [9], [24], [56], or attempts to link LCA with recycling processes [2]. Furthermore, there are building certification systems aiming to consider these aspects. Building certification systems (DGNB/ÖGNI 2018 [57], TQB/ÖGNB [58], SBTool [59], BREEAM [60], LEED [61], klimaaktiv [62]) consider different aspects for material sustainability. German Sustainable Building Council (DGNB) Certifications System 2018 and Building Research Establishment Environmental Assessment Method (BREEAM) even indicate the targeted SDGs in the individual categories. Some of these assessments and methods are discussed, and parameters required for different aspects are listed in Table 1.
LCA is defined by the International Organization for Standardization (ISO), Standard 14040:2006 [63] and Standard 14044:2006 [64]. It must be carried out according to the following steps: definition of the system boundaries, preparation of the life cycle inventory, impact assessment, externalization, and interpretation of the results. Furthermore, LCA for buildings is regulated in EN 15978:2011 [65]. Of the evaluations identified, LCA is the most widespread method used in the AEC industry. Nevertheless, it is seldom used in practice due to high data requirements and high implementation effort [55]. BIM-based assessment is also possible here, and numerous software tools are already on the market. However, those tools still have burdens, such as the difficulty of collecting and handling the considerable amount of data required, mainly in the early planning stage [54].
Furthermore, the degree of implementation in practice is still seldom compared to other sectors. A simplified approach has been developed as a countermeasure during the ENSLIC project [54], [66]. Peuportier et al. give a good overview of available LCA tools optimised for the construction industry [66]. Another study by Saghafi and Teshnizi attempts to connect LCA with recycling [2]. The method assesses energy savings by recycling building materials. The goal is to have an indicator of how worthy recycling materials and components are. Information about material selection, construction and deconstruction technologies, and recycling frequencies are required. The result of this study can be used in assessment tools as a factor separate from the embodied energy. The Austrian Institute for Building and Ecology (IBO) offers a method to reduce the environmental impact through summing up eco-indicators: Global Warming Potential (GWP), Acidification Potential, and Primary Energy Input-non-renewable (PEI) within one building evaluation [55]. The institute responsible for developing the method above also provides a database that offers a wide range of parameters based on EPDs [67]. It is available on the level of material (sectors EPD) and product (specific product of one company EPD).
The least researched area concerning the increase of material sustainability is the End-of-Life performance of buildings, which includes both deconstruction and subsequent material recycling. Some approaches have a research character or are not established in practice. The disposal indicator prepared by the IBO for the uniform assessment of the disposal properties of construction and materials at the building level has existed since 2012 [53]. The assigns a rating depending on the recycling potential and the disposal classification. A fictitious waste volume is calculated with those two parameters, and a building evaluation is issued concerning the areas of building components. Similar considerations are made in the case presented in [51]. This study is based on a building assessment from the recycling route, recycling technology, dismantling, transport, and processing (for preparation and recycling) costs.
The development of a BIM-based methodology for dismantling planning to promote reuse and high-quality recycling is presented in [2]. It requires information on the possible direction of movement of individual components, connections to neighbours, and deconstruction costs. DGNB provides a method regarding recycling (information about the recovery and disposal route) and disassembly friendliness of materials and consideration of these aspects in planning.
Material Passports (MPs) are a major instrument for enhancing CE in the AEC industry. The MP serves as a document of material characterization/composition of a building and simultaneously offers the potential for building up of digital material cadastres [38]. For example, this concept has been implemented in the form of the Swiss material cadastre (madaster), in which building owners can register and enter their objects [35]. The MPs generated in this way serve as a basis for urban mining processes, show circular and financial potential, and the possibility of component reuse. Very similar concepts to estimate the material stock of buildings in Switzerland and the city of Vienna are presented in [11], [68]. Due to age and topology, objects were categorized and linked with characteristic material intensities. The building volume can estimate the total mass. In the sense of policymaking, MP can be seen as the pendant to energy certificate. The idea of the MP is not new. A BIM-based implementation is already possible, as shown in [24]. The approach is based on a study that presents the systematics of an MP, the information to include, and the importance of material knowledge to implement urban mining in practice [9]. In addition, an evaluation index that is already used in practice to evaluate the waste and recycling volume of buildings is used [53]. The valuation index approach already used in practice is applied for LCA (without reducing to a building parameter) [55]. The result is the determination of material used over the life cycle of the building, produced recoverable mass (recycled material, how much non-recoverable waste), and the reprocessing-induced emissions amount of the ecological indicators GWP, AP, and PENRT.
A concept providing comprehensive documentation of various parameters is more far-reaching is described in [56]. Starting with LCA, Life Cycle Costing – LCC, and Social Life Cycle Assessment – SLCA (three pillars of sustainability), including service life and recyclability, through building physics properties such as fire resistance, chemical composition, mechanical properties, and appearance. However, implementation in practice seems questionable shortly due to the large number of parameters required. Some parameters have little to do with CE but serve as complete documentation, such as colour or transparency. Besides, any form of MPs needs geometrical sizes (lengths, areas, and volumes) and material densities form the basis of calculation.
Different building certification systems target different kinds of buildings as systemised by [9], [56]. Table 1 shows the most relevant certifications systems, relevant sustainability criteria, and possible certification objects.
The EU taxonomy directive (EU-tax) on establishing a Framework to facilitate sustainable investment will take effect from 2023 [69]. Depending on the scope, different evaluation categories have to be assessed. In the case of new buildings, all evaluation fields must be taken into account (climate protection, adaptation to climate change, biodiversity & ecosystems, water, circular economy, environmental pollution). In the case of existing buildings, one must assess the only evaluation fields: climate protection and adaptation to climate change. In the case of refurbishment, one must evaluate all categories of new buildings except for biodiversity. The interest rate varies depending on the real estate valuation.
Scope and fields of relevant building certification systems
Certification System |
Sustainability Scope |
Fields |
BREEAM |
Energy, water, material/recycling, waste, land use |
Office buildings, sales facilities, industrial buildings, schools, health care facilities, courthouses, prisons, hotels, entire settlements |
LEED |
Water efficiency, energy and resource consumption, recycling |
Mostly: administrative buildings. Also: residential buildings, schools, retail outlets, healthcare facilities, urban development, etc. |
DGNB/ÖGNI |
Ecological, economic, socio-cultural quality |
New buildings: office, education, health, production, research, residential, etc. Existing buildings: office and administration |
TQB/ÖGNB |
Ecological quality, energy, resource efficiency |
residential, office, logistic center, education |
klimaaktiv |
energy, building material |
Office buildings, industrial buildings, schools, courthouses, research, entire settlements |
EU-tax |
Climate, adaptation to climate change, biodiversity & ecosystems, water, CE, environmental pollution |
New constructions, existing buildings, renovation, individual measure |
The scope of the presented study focuses primarily on buildings (as a crucial element of the AEC industry), including the phases of demolition and refurbishment, thereby not limited to certain types of buildings. Furthermore, sustainability assessment focuses on ecological aspects (LCA, sustainable use of materials by reuse or recycling, prolongation of service life) and the costs incurred for reuse, recycling, and disposal. However, economic sustainability is not considered. Furthermore, only selected LCA indicators are used (GWP, PEI, and AP). Eutrophication potential, for example, is not considered but can be integrated if necessary. The various evaluation methods require different parameters to perform. The necessary parameters for the evaluation methods applied in LCA, End-of-Life, MP, and Building Certification have been through the literature review presented in the previous section. Then the most relevant ones have been identifed.
This distillation process was conducted according to the following principles: a) Number of requirements (NR) – eliminate seldom occurring parameters, b) Nomenclature – merge the parameters if they have different names but similar meanings, c) Quantifiable – eliminate unquantifiable parameters unless it is possible to attach a quantifiable unit. The process reduced the initial 65 parameters to the final 16 parameters. Common and well-established parameters in practice, such as the geometry of components and density of materials, were excluded, and operation energy, which is already very well established in the form of Energy Certificate.
Table 2 shows the most relevant parameters needed to assess the sustainable use of material resources. The table summarizes the various methods for evaluating environmental impacts and resources management in terms of CE. LCA and End-of-Life evaluation methods are based on numerous eco-indicators and other material parameters. Geometric quantities, parameters that can be assigned to materials, and material parameters, which are very strongly dependent on technologies, such as recycling quotes, were identified. The terms in brackets indicate the area they originate: MP stands for Material Passport, Dec for deconstruction, Cert for building certification, Rec for recycling. Some examples for the distillation principles: Principle a – LCA was reduced to the most common evaluation parameters with the note that much more indicators such as ozone depletion, ozone creation, eutrophication, water scarcity, abiotic lack of fossil resources, abiotic resource use, biotic resource use, human toxication, and land use considered in [24], [55]. Some methods required an extended PEI to include an exact breakdown of different energy contributions for material extraction, transport, erection, deconstruction, recycling, etc. Factors like recycling circles and recycling probability were eliminated. If recycling is possible, it should be recycled, and they were seldom required.
Relevant Parameters for integrated sustainability Assessment and frequency of nomination
Parameter |
Type of Assessment |
NR |
SDGs |
|
Material |
1: LCA-GWP |
MP [9], [24], [56]; Dec [52]; LCA [55], [56]; Cert [57], [59] |
7 |
9, 11, 12, 13 |
2: LCA-AP |
6 |
(9), 11, 12, |
||
3: Extended/broken down LCA-PEI/ Embodied Energy |
MP [9], [24], [56]; Dec [2], [52]; LCA [2], [52], [55]; Cert [57], [59] |
10 |
(9), 11, 12 |
|
4: Renewable/non-Renewable material |
2 |
9, (11), 12 |
||
5: Internal/external reserves |
2 |
(9), 11, 12 |
||
6: Material stock |
2 |
(9), 12 |
||
Others |
7: Disposal method |
4 |
(9), 11, 12 |
|
8: Recycling method |
3 |
(9), 11, 12 |
||
9: Recycling/Reuse potential/percentage |
LCA [2]; Dec [2]; MP [9], [56]; Rec [53], [51]; Cert [58], [59] |
8 |
(9), 11, 12 |
|
10: Material/Element-Lifetime |
6 |
(9), (11), 12 |
||
11: Building Lifetime |
5 |
(9), (11), 12 |
||
12: CDW total |
LCA [2]; Dec [2]; MP [9], [24], [56]; Rec [53]; Cert [58], [59] |
7 |
(9), 11, 12 |
|
13: Export and consumption |
MP [9] |
1 |
(9), 11, 12 |
|
14: Dismantling to Recycling effort |
4 |
(9), 11, 12 |
||
15: Numbers of Layers |
2 |
(9), (11) |
||
16: Separability |
3 |
(9), (11), 12 |
The cycles (more precisely, the amount of original material per cycle) are defined by recycling percentage/potential, a more commonly used parameter. Principle b – Quality of recycling material was eliminated since quality is a variable that is difficult to measure. It is nevertheless a significant parameter, as the technical properties, such as mechanical behaviour and pollutant loads, determine possible uses of the recycled material. The RecBVO regulates, for example, the use of processed building rubble, concrete, and asphalt demolition, depending on the eluate content [44]. According to the waste hierarchy, reuse has a higher priority than recycling but a similar goal; therefore, these two are merged.
Principle c – Dismantling, transportation, processing, and recycling effort/ease is reduced to a comprehensive dismantling to recycling effort, like the extended/broken down PEI. It is now valued with monetary expenditure. With the tonne-kilometres for transportation (Common criteria in logistics/freight transport) and information on the required energy input, an energy efficiency indicator could be calculated (energy per mass and distance) [70]. Measuring the distance can be easily done using Google Maps or other Geoinformation System (GIS)-Services. This assessment is possible by linking further data such as energy rates and costs. If material is renewable or non-renewable is not a quantifiable unit. However, it is possible to quantify the mass of materials, and in the case of renewable materials, it is possible to attach a regeneration rate. For non-renewable materials, the regeneration rate is zero or negligibly small in the example of sand (weathering of rock) or fossil fuels.
Parameters were also found that are input in one evaluation methodology and output in another. A good example is material efficiency which needs information on 1: material availability, 2: material recyclability, and 3: self-sufficiency [9]. These three needs information of 1: internal/external reserves, stock-secondary raw material and production, recycling potential, consumption of region, recycling material in a system, and stock change, 2: CDW total, factor for selective disassembly, and factor for separability to calculate CDW and recycling material flow, as well as the factor for technology and 3: internal reserves, stock-secondary raw material and production, recycling potential, regional material consumption, recycling material in the system, stock change and export data. Therefore, the output data of material availability, recyclability, and self-sufficiency are inputs to assess material efficiency, but without further specifications of how the assessment should be done [56]. Another example is the recycling and reuse rate, which is input in other assessments, but the output in [29]. The focus in this paper lies on the basic parameters that make computational operations feasible, where possible. As a result, the following steps have been conducted. Material efficiency (Material criticality/availability, recyclability, and self-sufficiency) was split up into internal/external reserves, material stock (secondary raw material, production, total and changes), recycling potential, consumption of region, CDW total, export. In recyclability, the factors for selective disassembly, separability, and technology were merged.
Based on extensive literature review and parameter analysis, a Framework for evaluating CE-potentials of building materials was developed, as presented in Figure 2. Possible secondary applications (SAs) for the fields of deconstruction management (SA1), resource management (SA2), and conclusions for planning (SA3) were embedded into the Framework.
The Framework presented in Figure 2 focuses on the Austrian market and considers Austrian standards and regulations. However, it is extendable for other markets for which other international or specific regional policies would have to be incorporated. The Framework is based on life cycle stages occurring in disassembly to disposal and recycling/reuse processes of building materials and assigned to each stage a regulation field. Further End-of-Life scenarios are given for usual and possible disposal and recycling routes. In the next step, areas of application for the reuse of these reprocessed/recycled or reusable materials are defined, and economic and ecological parameters are determined for them, which subsequently serve as a data basis for the assessment of material flows. The intent is to serve as a decision-making aid to which reuse of the materials has the best economic/ecological proportionality.
Framework for CE evaluation of buildings and possible secondary application areas
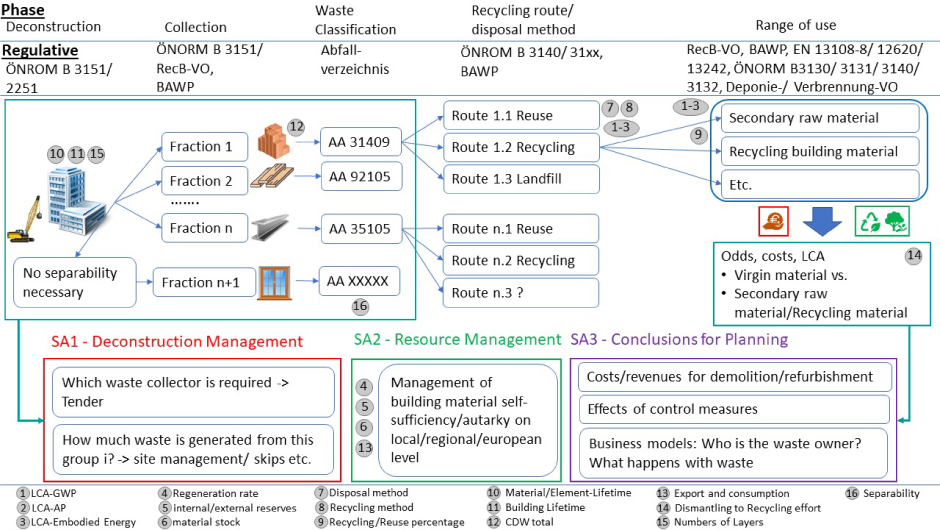
For example, a concrete column can be reused, recycled, or sent to a landfill. The next step is to know how recycling happens and what one can do with the recycled material. It is possible to take the concrete waste and feed it into cement production. Another possibility is the production of recycling concrete. But more often, it is used as a filling material. All these possible uses must be attributed to the above parameters (listed in Figure 2), especially 1–3, 7–9, and 14 (environmental impact and costs). If these are compared with the primary material counterparts, one can see how valuable and feasible the material path is.
For this purpose, one also needs data on LCA of recycled material/secondary raw material and primary raw material and the production costs incurred. Based on these processes, the SAs can be derived. Knowledge about the accruing masses and volumes of the waste loads to be separated and collected flows into SA1 enables on-site deconstruction management. Demolition companies primarily use it for planning and handling deconstruction projects. SA2 is especially interesting for governmental structures to allow stable and regional material management, thus decreasing the dependency on imports, strengthening self-sufficiency, keeping the value chain as much as possible in the country, and identifying fields that need regulatory measures (e.g., taxes). SA3 serves as a planning basis for dismantling projects and the whereabouts of waste masses by comparing different business models with the waste loads and estimating the following consequences of regulatory measures. The three SAs used in the presented study are shown in Figure 3. Using BIM and GIS as a building database and linking to other databases, the SAs can be served and the areas listed can be supported by digital tools.
On the one hand, it is essential to depict the waste and material flows under the applicable regulations and the possible recycling processes. Using BIM, waste directory numbers can be assigned to individual materials (possibly some material packages that do not have to be separated), which are assigned to disposal and processing routes. Waste director numbers are shown in [71]. Depending on potential areas of use, the circularity is now divided up. In these steps, one must consider the waste hierarchy and other regulations, thus describing the path of technical feasibility of recycling and reuse. To evaluate building value at End-of-Life and manage on-site deconstruction activities, knowledge of the material composition is essential before the demolition.
Secondary Applications, with building Information linked to other databases
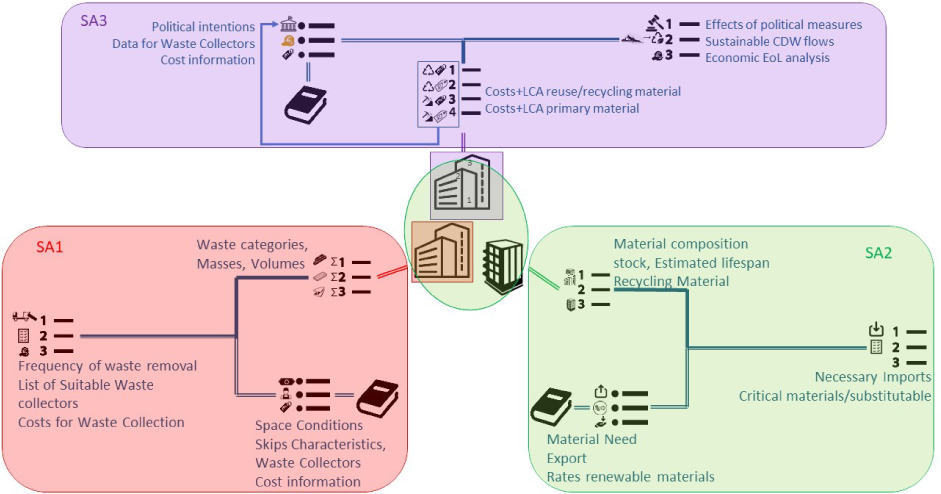
To assess the economic/environmental proportionality, one must compare the different costs and environmental impacts of recycled materials with the costs and environmental impacts of primary materials. For this purpose, parameters 1, 2, 3, 9, 14, and information for primary and secondary materials (EPD and cost information) are required. It must be legally regulated at what point the effort/benefit is justified in a further step. The by-products arising in this consideration, and through the integration of the remaining parameters 4, 5, 6, and 13, concern firstly the tendering of demolition projects and on-site management. Secondly, the planning of demolitions and the associated business models (what happens to the waste) and how properties are to be valued, and thirdly local, regional and state and European resource management of the construction sector (whereby the importance decreases with spatial expansion, presumably due to the high masses). To rate the evaluation parameters, it seems to make sense to give ecological parameters a higher weighting than, for example, costs. Ultimately Parameter 9 Recycling/Reuse percentage is the most crucial for CE and therefore is highest ranked, followed by Parameters 1–3. It also makes sense to consider these factors per parameter 14 Dismantling to recycling effort (which represents the costs). Since the Framework ultimately aims to provide a clear decision-making aid for the criterion formulated in the AWG for the required material recovery of CDW, a weighting is ultimately also incumbent on the legislator.
In the research, several obstacles were faced that can be summarised as data inheterogeneities. These included, for example, inconsistent nomenclature in different assessment methods, a broad scale of national, EU-wide, and international regulations, directives, and laws that one must consider. The focus is on the national aspects of waste management and the International Standards of LCA and EPDs, which are necessary to support the open market and transport of goods and create an available uniform regulated database.
An extensive literature review was conducted to overview the legal Framework, assessment methods, and already considered aspects in building certification. Due to the large number of publications in the individual sub-areas, relevant literature had to be selected and then analysed. Furthermore, a parameter study of the individual assessment methods was carried out and examined for similarities. The parameters found were reduced as far as possible. A Framework for the holistic consideration of the CE of a building was built up, and potential additional fields of application were defined. These SAs can take over the role of governmental control and measure application (e.g., what effects does a control measure have), for building owners and project developers decision support, what should be done with the resulting waste, and for construction companies on site, how to manage demolition projects.
But some advantages derive from the establishment of CE in the AEC industry. For example, gaining economic potentials, such as creating new economic sectors and occupational fields, which entails increased employment, and a regional value chain with the direct and indirect effects, is very welcome from a financial perspective [14], [72]. For example, the development and establishment of new business models. Waste management follows two approaches, the elimination of contaminated sites and the creation of functioning waste systems. In the municipal waste sector, creating deposit-refund systems is a common solution. A container with a product is purchased for a purchase price plus a refund. If the container is returned, the refund is returned [74]. This concept is not identically realisable, but it is conceivable in an adaptive way. For example, the Lukas Lang construction system has developed a system where the connecting nodes are modular, and the connections are detachable [75]. Container and product coincide, and a part of the purchase price can be refunded when returning functional products.
In a further step to this work, one must examine whether and in what form the necessary data are available and what influences on these effects and changes are possible, e.g., temporary impact on recycling rates, costs for disassembly, and material processing. However, collecting data from externals generates further problems: data must be brought to a uniform format, kept up to date, and verified for reliable use (data must be accessible and interoperable [5]). Therefore, an open, regulated database should be created, which is embedded in the legal Framework and allows sustainable material management in the AEC industry in the sense of a CE. One possible solution is the approach by Pauliuk et al., presenting a general open-source data model for socioeconomic metabolism [73]. This creation of data and networking is considered SDG 9 – Infrastructure in the form of Integrated Material data processing and construction waste management.
The parameters of this study presented in Table 1 were assigned to SDGs they influence. One should clearly distinguish that parameters (P) represent sub-goals or indicators. For example, P1 LCA-GWP explicitly represents goal 9.4.1 CO2 emissions. Other examples are P6 material stock representing indicator 12.2.1 material footprint and P9 recycling/reuse potential/percentage – indicator 12.5.1 national recycling rate. Some parameters can only be assigned to the SDGs in a broader sense. Examples of these are P1–3 and P7–9 representing Goal 11.6 reduce environmental impact, and P7–9 directly influencing indicator 11.6.1 proportion of municipal solid waste of cities, as treatment and recycling reduce the disposal of CDW. The consideration of P5, in turn, can be assigned to, for example, target 11.a strengthen national development; P5,6,13 influencing indicator 12.2.2 domestic material consumption. P1 affects 13.2 integrate climate change measures (specifically 13.2.1c reduce greenhouse gas). Lastly, some parameters are not assignable, such as P15. In the case of SDG 9 industry, innovation, and infrastructure, the parameters (except P1) cannot be assigned to it, but rather that the parameters must be available to achieve the SDG 9 targets in the building sector. For example, the application of the Framework represents Goal 9.4 resource-efficient industry, and BIM and GIS-based implementation in the broadest sense represents Goal 9.c increase information and communication technology access (no expansion of access, but an expansion of application areas). Similarly, Objectives 11.b implement policies for resource efficiency, 12.2 management and efficient use of natural resources, and 12.4 environmentally sound waste management throughout the life cycle, are strengthened by implementing the Framework (especially resource efficiency).
The Framework and SAs linked to it cover well the research topics identified in [13]. Most-influencing practice instruments identified on waste management costs by Galvez-Martos et al. (and linked to the SAs of the proposed Framework in this paper) are following:
SA1 – Deconstruction management supports: waste treatment and recovery – separate and process waste streams to maximise the production of high-quality recycled aggregates (impact high);
SA2 – Resource Management supports: reuse deconstruction – evaluate and maximize the recovery of materials with the principles of transparency, i.e., all elements are visible, regularity, i.e., identical materials are used for the same applications, and simplicity, i.e., a limited number of materials and components easy-to separate materials (impact high);
SA3 – Conclusion for Planning supports: Economic Instruments – economic instruments to encourage and maximise the environmental performance like landfill tax, aggregates levy, or Business-to-Business refund systems (impact high) [39].
The users of SAs are for SA1 – contractors and demolition contractors, SA2 – state bodies, SA3 – state bodies, planners such as architects, and developers. The support of crucial stakeholders is visible when comparing this with stakeholders listed in [39] with influence medium-high to high: Contractors (4), demolition contractors (3), Developers (3), standardisation bodies (1), designers (1) waste management organisations (4), and clients (3), Suppliers (1), site managers (1). (The number in brackets stands for how many fields with importance medium-high to high are mentioned).
Despite numerous attempts to increase CE in AEC, the potential that needs to be tapped is still enormous. Due to the complexity and interaction of different fields, such as planning, construction, legislation, waste management (deconstruction, demolition, and recycling), and facility management, a joint effort is required to collect and process data made available in a sound manner. This paper presents a conceptual Framework for assessing the sustainable CE potential of buildings to reduce resources consumption and related environmental impacts to tackle this problem. The Framewework focuses primarily on enhancing environmental sustainability and resources efficiency in AEC and only selected relevant costs to achieve this demolition followed by reuse and recycling (and if unavoidable disposal), rather than on assessment and optimisation of economic sustainability. A holistic environmental and economic assessment should be investigated further, identifying trade-offs within a quantitative multiple-objective approach. The presented Framework is conceptual; in the next future step, a proof of concept and validation is planned. For this purpose, on the one hand, the plan is to hold expert discussions which will assess the completeness of the legal requirements, discuss possible extensions, and define material-specific utilisation options and areas of application.
On the other hand, one should assess the concept for applicability and monitor material flows within use cases of deconstruction projects. In this way, the proof of concept can be delivered by identifying the missing data, considering additional information, and testing applicability. Furthermore, it is necessary to involve relevant institutions regarding assessment outputs, when a recycling route is to be carried out when it is recommended, and under which conditions it is not considered feasible.
Suppose the presented Framework and the linking of SAs, are challenging to implement, a suitable digital environment needs to be created, and a significant amount of data is required. Therefore, measures that are easier to implement and correspond to recommendations for action have been considered. Table 3 presents an interim compilation of the most important measures and evaluation criteria for a quick and straightforward assessment of the usefulness of materials identified.
Measures and assessment criteria for increasing the sustainable use of materials
Field |
Description |
|
Measures guideline |
Producer responsibility |
Obligation to take back materials that companies also produce |
New business models |
Establishing the turntoo model [76] for comparatively short-lived elements such as floor coverings, wall coverings, etc. |
|
Control measures |
Levies for landfilling penalties for non-compliance with the dismantling concept |
|
Waste concept |
Actual implementation of the demolition concept from the deconstruction planning in the deconstruction implementation and the recommendations drawn up by the deconstruction expert |
|
Planning |
Office buildings, industrial buildings, schools, courthouses, research, entire settlements |
|
Functional tendering |
New constructions, existing buildings, renovation, individual measure |
|
Purity grade |
Consideration of purity grade of the waste fractions |
|
Assess guideline |
Recycling rates |
Consideration of recycling rates |
Costs |
Consideration of dismantling and disposal costs |
|
Reuse |
Consideration of whether standardised structures are suitable for reuse |
|
Longevity |
Consideration of the service life of materials and components |
|
Raw material substitution |
Consideration of the substitution potential of primary material |
|
Energy |
Consideration of tonne-kilometres and type of transport up to treatment/reprocessing |
After validating the framework, a final package of measures will be developed. The material-independent Framework itself is to be classified in developing advanced performance evaluation criteria for wasted materials and recycled products and extending the research boundary of CDW, embedding in BIM in the field exploring the more efficient use of information technologies and SA3 in Reducing CDW from early project stages.
It is important to recall that time is pressing and rapid, but no hasty action is required to provide enough time to adapt and overcome the unsustainable use of resources. Even though recycling processes of (mineral) CDW are similar across Europe and well established, the final use of secondary material differs. The comparison to primary materials is missing entirely but essential for CE evaluation and should be carried out. Setting a sustainable and efficient future in building demolition in a constant and prosperous manner supporting material and component reuse is one of its essential aspects. It requires investment in terms of time, money, skills, tools, and technologies. Changes are urgent because planning and deconstruction are often apart 50 years or more. Therefore, a paradigm change in the planning of new buildings and deconstruction projects is necessary.
Abbreviations |
|
AEC |
Architecture, Engineering, and Construction |
AP |
Acidification Potential |
AWG2002 |
Waste Management Law 2002 |
BIM |
Building Information Modelling |
CDW |
Construction and Demolition Waste |
CE |
Circular Economy |
Cert |
Building Certification |
CO2 |
Carbon Dioxide |
Dec |
Deconstruction |
EPD |
Environmental Product Declaration |
EU |
European Union |
GIS |
Geoinformation System |
GWP |
Global Warming Potential |
IBO |
Austrian Institute for Building and Ecology |
IFC |
Industry Foundation Classes |
LCA |
Life Cycle Assessment |
MP |
Material Passport |
NR |
Number of requirements |
P |
Parameter |
PEI |
Primary Energy Input |
Rec |
Recycling |
RecBVO |
Recycling Building Materials Directive |
RFID |
Radio Frequency Identification |
SA |
Secondary Application |
SDG |
Sustainable Development Goal |
SQL |
Structured Query Language |
UN |
United Nations |
WFD |
Waste Framework Directive |
Life cycle assessment of Building materials: comparative analysis of energy and environmental impacts and evaluation of the co-efficiency improvement potential ,Building and Environment , Vol. 46 (5),pp 1133-1140 , 2011, https://doi.org/https://doi.org/10.1016/j.buildenv.2010.12.002
, Recycling value of building materials in building assessment systems ,Energy and Buildings , Vol. 43 (11),pp 3181-3188 , 2011, https://doi.org/https://doi.org/10.1016/j.enbuild.2011.08.016
, - , United Nations – International Resource Panel, Global Resource Outlook 2019, Natural Resources for the Future we want, A Report of the International Resource Panel., United Nations Environment Programme., 2019
- , World Green Building Council, Towards zero-emission efficient and resilient buildings - Global Status Report, 2016
- , European Commission, Communication from the Commission to the European Parliament, the European Council, The Council, the European Economic and Social Committee and the Committee of the Regions, 2019
- , Austrian Federal Ministry for Sustainability and Tourism, Waste Management Plan 2017 - Part 1 (in German), 2017
- , In association with European Commission and bio Intelligence Service, Service Contract on Management of Construction and Demolition Waste – SR1, Final Report Task 2, 2011
Urban Mining - A Contribution to Reindustrializing the City ,Journal of Industrial Ecology , Vol. 15 (3),pp 339-341 , 2011, https://doi.org/https://doi.org/10.1111/j.1530-9290.2011.00345.x
, - , Entwicklung eines Konzepts zur Förderung der Kreislaufwirtschaft im Bauwesen: Materieller Gebäudepass und Design for Recycling für das Bauwesen – Endbericht, 2011
- , Eco-efficient building with on-site resources., In Atlas Recycling, 2018
Potentials for a circular economy of mineral construction materials and demolition waste in urban areas: a case study from Vienna, Resources ,Conservation and Recycling , Vol. 161 , 2020, https://doi.org/https://doi.org/10.1016/j.resconrec.2020.104942
, - , European Parliament, Directive 2008/98/EC of the European Parliament and of the Council, of 19 November 2008, on waste and repealing certain Directives, 2008
Status quo and future directions of construction and demolition waste research: A critical review. ,Journal of Cleaner Production , Vol. 240 , 2019, https://doi.org/https://doi.org/10.1016/j.jclepro.2019.118163
, - , European Commission, Communication from the Commission to the European Parliament, the European Council, The Council, the European Economic and Social Committee and the Committee of the Regions, on Resource Efficiency Opportunities in the Building Sector, 2014
The Circular Economy–A new sustainability paradigm? ,Journal of cleaner production , Vol. 143 ,pp 757-768 , 2017, https://doi.org/https://doi.org/10.1016/j.jclepro.2016.12.048
, - , Report of the World Commission on Environment and Development: Our common future., 1987
- United Nations, Sustainable Development Goals (SDGs), https://sdgs.un.org/goals, [Accessed: 24-November-2020]
- , Die drei Dimensionen einer Nachhaltigen Entwicklung. Operationalisierung einer Nachhaltigkeitsstrategie: Ökologie, Ökonomie und Soziales integrieren, Gabler Verlag, 2009
Analysing interactions among sustainable development goals with integrated assessment models. ,Global Transitions , Vol. 1 ,pp 210-225 , 2019, https://doi.org/https://doi.org/10.1016/j.glt.2019.10.004
, Resource nexus perspectives towards the United Nations sustainable development goals. ,Nature Sustainability , Vol. 1 (12),pp 737-743 , 2018, https://doi.org/https://doi.org/10.1038/s41893-018-0173-2
, A conceptual Framework for understanding the contribution of building materials in the achievement of Sustainable Development Goals (SDGs). ,Sustainable Cities and Society , Vol. 52 , 2020, https://doi.org/https://doi.org/10.1016/j.scs.2019.101869
, A method for determining buildings' material composition prior to demolition ,Build. Res. Inf. , Vol. 44 (1),pp 51-6 , 2016, https://doi.org/https://doi.org/10.1080/09613218.2014.979029
, - , Describing the changes in architectural information technology to understand design complexity and free-form architectural expression, ITCON 11 (Special Issue The Effects of CAD on Building Form and Design Quality), 2006
Improving the recycling potential of buildings through Material Passports (MP): An Austrian case study, journal of Cleaner Production , Vol. 217 ,pp 787-797 , 2019, https://doi.org/https://doi.org/10.1016/j.jclepro.2019.01.212
, Integration of LCA and LCC analysis within a BIM-based environment ,Automation in Construction , Vol. 103 ,pp 127-149 , 2019, https://doi.org/https://doi.org/10.1016/j.autcon.2019.02.011
, BIM-Based End-of-Lifecycle Decision Making and Digital Deconstruction: Literature Review. ,Sustainability , Vol. 12 (7), 2020, https://doi.org/https://doi.org/10.3390/su12072670
, Designing out construction waste using BIM technology: Stakeholders' expectations for industry deployment ,Journal of Cleaner Production , Vol. 180 ,pp 375-385 , 2018, https://doi.org/https://doi.org/10.1016/j.jclepro.2018.01.022
, - , BIMReL: The Interoperable BIM Library for Construction Products Data Sharing., In Digital Transformation of the Design, Construction and Management Processes of the Built Environment, 2020
Salvaging building materials in a circular economy: A BIM-based whole-life performance estimator. ,Resources, Conservation and Recycling , Vol. 129 ,pp 175-186 , 2018, https://doi.org/https://doi.org/10.1016/j.resconrec.2017.10.026
, Buildings as material banks using RFID and building information modeling in a circular economy. ,Procedia CIRP , Vol. 90 ,pp 143-147 , 2020, https://doi.org/https://doi.org/10.1016/j.procir.2020.02.122
, - Österreichische Recyclingbörse Bau, RBB–Recycling Börse Bau, http://www.recycling.or.at/rbb/cake_rbb/, [Accessed: 17-November-2021]
- Materialnomaden, re:store – Harvestmap Austria, https://www.materialnomaden.at/store/, [Accessed: 17-November-2021]
- Superuse Studios NL, Harvestmap Netherland, https://www.oogstkaart.nl/, [Accessed: 17-November-2021]
Model and data management issues in the integrated assessment of existing building stocks. ,Organization, technology & management in construction: an international journal , Vol. 12 (1),pp 2148-2157 , 2020, https://doi.org/https://doi.org/10.1016/j.jobe.2019.01.017
, Calculation and evaluation of circularity indicators for the built environment using the case studies of UMAR and Madaster ,Journal of Cleaner Production , Vol. 243 , 2020, https://doi.org/https://doi.org/10.1016/j.jclepro.2019.118482
, - , United Nations, Growing in Circles, climate-ready, resilient, resource-efficient, and equitable cities, 2018
Integrated construction waste management, a holistic approach. ,Scientia Iranica , Vol. 23 (5),pp 2044-2056 , 2016, https://doi.org/https://doi.org/10.24200/sci.2016.2269
, An overview of construction and demolition waste management in Canada: A lifecycle analysis approach to sustainability. ,Clean Technologies and Environmental Policy , Vol. 15 (1),pp 81–91 , 2013, https://doi.org/https://doi.org/10.1007/s10098-012-0481-6
, Construction and demolition waste best management practice in Europe ,Resources, Conservation and Recycling , Vol. 136 ,pp 166-178 , 2018, https://doi.org/https://doi.org/10.1016/j.resconrec.2018.04.016
, Circular economy in waste management–Socioeconomic effect of changes in waste management system structure. ,Journal of environmental management , Vol. 267 , 2020, https://doi.org/https://doi.org/10.1016/j.jenvman.2020.110564
, - , Construction and Demolition Waste Management in Australia, Final Industry Report, Project 1.65 (2021), Sustainable Built Environment National Research Centre, 2021
- , European Comission, Circular Ecomomy – Principles for Buildings Design, 2020
- , Österreichisches Parlament, AWG - Abfallwirtschaftsgesetz 2002, Gesamte Rechtsvorschrift für Abfallwirtschaftsgesetz 2002, 2018
- , BMLFUW (Österreichisches Bundesministerium für Land- und Forstwirtschaft, Umwelt und Wasserwirtschaft), Gesamte Rechtsvorschrift für Recycling-Baustoffverordnung (BGBl. II Nr. 181/2015), 2016
- , Austrian Standards, ÖNORM B 3151 - Rückbau von Bauwerken als Standardabbruchmethode, 2014
- , Austrian Standards, ÖNROM_EN ISO 16000-32 - Indoor air Identical (IDT) with ISO, 2014
- Recyclingportal, Vienna BauKarussell project creates new jobs in construction with reuse, https://recyclingportal.eu/Archive/32762, [Accessed 25-November-2021]
Energy-Cement-Carbon Emission Nexus and its Implications for Future Urbanization in China. ,Journal of Sustainable Development of Energy, Water and Environment Systems , Vol. 9 (2),pp 1-15 , 2021, https://doi.org/https://doi.org/10.13044/j.sdewes.d8.0354
, - Lafarge, OMV, VERBUND, and Borealis, C2PAT Austria, www.borealisgroup.com, [Accessed: 27-November-2021]
- Holcim, Advancing in CO2 capture and utilization in Austria, https://www.holcim.com/advancing-co2-capture-storage-austria, [Accessed: 27-November-2021]
- , Urban Mining, Leitfaden zur Vermeidung nicht recyclingfähiger Bauabfälle bei künftigen kommunalen Hochbauvorhaben, Fraunhofer IRB Verlag, Stuttgart, 2019
Deconstruction programming for adaptive reuse of buildings. ,Automation in Construction , Vol. 107 , 2019, https://doi.org/https://doi.org/10.1016/j.autcon.2019.102921
, - , IBO (Österreichisches Institut für Bauen und Ökologie GmbH), Leitfaden zur Berechnung des Entsorgungsindikators EI KON für Bauteile und das Entsorgungsindikators EI10 auf Gebäudeebene, Version 2.0, 2018
Life cycle assessment in buildings: The ENSLIC simplified method and guidelines. ,Energy , Vol. 36 (4),pp 1900-1907 , 2011, https://doi.org/https://doi.org/10.1016/j.energy.2010.03.026
, - , IBO (Österreichisches Institut für Bauen und Ökologie GmbH), Leitfaden zur Berechnung des Oekoindex OI3 für Bauteile und Gebäude, Version 4.0, 2018
- , Materials passports–Best practice., Innovative solutions for a transition to a circular economy in the built environment, 2019
- German Sustainable Building Council, DGNB Certification System 2018 (in German), https://www.dgnb-system.de/de/gebaeude/neubau/kriterien/oekobilanz-des-gebaeudes/index.php, [Accessed: 1-December-2021]
- Austrian Society for Sustainable Building ÖGNB, TQB (Total Quality Building) Certification System (in German), https://www.oegnb.net/tqkrit.htm, [Accessed: 26-November-.2021]
- International Initiative for a Sustainable Built Environment, SBTool (Sustainable Building Tool), Version 2012, http://www.iisbe.org/node/140, [Accesssed: 26-November-2021]
- BRE Global, BREEAM (Building Research Establishment Environmental Assessment Method), Version 2015, https://www.bregroup.com/greenguide/calculator/page.jsp?id=2071, [Accessed: 1-December-2021]
- U.S. Green Building Council, LEED (Leadership in Energy and Environmental Design), Version 2020, https://www.usgbc.org/leed/v41, [Accessed: 1-December-2021]
- Klimaaktiv, Klimaaktiv Certification System 2020 (in German), https://www.klimaaktiv.at/bauen-sanieren/gebaeudedeklaration.html, [Accessed: 10-December-2020]
- , International Organization for Standardization, ISO 14040: Environmental management - Life cycle assessment - Principles and Framework, 2006
- , International Organization for Standardization, ISO 14044: Environmental management — Life cycle assessment — Requirements and guidelines, 2006
- , European Committee for Standardization, EN 15978: Sustainability of construction works - Assessment of environmental performance of buildings - Calculation method, 2011
- , State of the art for use of LCA in building sector: Deliverable D2 of the project ENSLIC_BUILDING: Energy Saving through Promotion of Life Cycle Assessment in Buildings, 2009
- Austrian Institute for Building Biology, Baubook - Passive House-Component Catalogue 2020 (in German), https://www.baubook.info/eco2soft/;Eco2soft,2020.baubook-eco2soft, [Accessed: 15-November-2020]
Exploration of the Urban Stocks ,Journal of Environmental Economics and Management , Vol. 18 (1),pp 41-48 , 2008
, - , Regulation (EU) 2020/852 of the European Parliament and the Council of 18 June 2020, on the establishment of a Framework to facilitate sustainable investment, and amending Regulation (EU) 2019/2088.
What is energy efficiency?: Concepts, indicators and methodological issues ,Energy policy , Vol. 24 (5),pp 377-390 , 1996, https://doi.org/https://doi.org/10.1016/0301-4215(96)00017-1
, - , BMLFUW (Österreichisches Bundesministerium für Land- und Forstwirtschaft, Umwelt und Wasserwirtschaft), Gesamte Rechtsvorschrift für Abfallverzeichnisverordnung (BGBl. II Nr. 570/2003), 2020
- , Economic effects of recycling selected existing substances and wastes., Austrian Institute for Economic Research, In Recycling und Rohstoffe Band 11, 2016
A general data model for socioeconomic metabolism and its implementation in an industrial ecology data commons prototype. ,Journal of Industrial Ecology , Vol. 23 (5),pp 1016-1027 , 2019, https://doi.org/https://doi.org/10.1111/jiec.12890
, Economic viability of the deposit refund system for beverage packaging waste–identification of economic drivers and system modelling ,Journal of Sustainable Development of Energy, Water and Environment Systems , Vol. 9 (3),pp 1-33 , 2021, https://doi.org/https://doi.org/10.13044/j.sdewes.d8.0386
, - Lukas Lang Building Technologies, Modular Building System Lukas Lang (in German), https://www.lukaslang.com/de/startseite/, [Accessed 25-November-2021]
- , Material Matters, Ullstein Buchverlag GmbH, 2021