Water is a vital source of life on earth, particularly for Human Life. Around 785 million people have no access to clean water, and 144 million people fulfil their needs from surface water [1]. Water vulnerability is affecting 1.42 billion people in the world [2]. Only 3% of water is fresh in the World [3], and this water is becoming scarce due to mismanagement, misuse, contamination, and over-extraction. Urbanization, population growth, and industrialization have increased the water demands in the world. Climate change also contributes to water scarcity as extreme weather conditions like floods, droughts, change in precipitations, and sea-level rise contaminate freshwater resources, destroy water and sewage infrastructure, and reduce available water. Water-related disasters occurred about 74% from 2001 to 2018, destroying water and sanitation infrastructure. These disasters’ frequency will increase due to climate change. On the other hand, global water demand will increase about 20−30% by 2050 because of population growth, high living standards, and food and energy demand [4].
Desalination is an effective technique for removing salts and undesirable quantities of minerals from water [5]. It produces freshwater, which is potable and also useable for sanitary purposes [6]. Desalination of seawater and brackish water is the best alternative technique to cope with the rising issue of scarcity of potable water [7]. This can potentially make the saline water usable on a commercial scale, but energy- and cost-effective solutions are needed along with environmental friendliness and social acceptability [8]. The costs of desalination plants are high because a huge amount of power is required for plant operation. This extensive input power requirement makes these plants nonviable [6]. Global desalination installed capacity has an increasing trend from 1970 at an average of 75% to an average of 7% in the last decade [9]. Land-based desalination plant in the world has the capacity of 69.1 million m3/day. Global Offshore desalination plants’ capacity is 2 million m3/d. Mobile desalination plants contribute globally 67,000 m3/d [9]. The number of desalination plants worldwide is as follows: operational 16,876, under construction 270, and offline 3825 [9]. Reverse Osmosis (RO) is currently applied in 85% of operational desalination plants and about 91% of under-construction plants [10]. The Middle East region accounts for 39% of global desalination capacity, and most operational desalination plants are present here [9]. Fossil fuel (thermal) based desalination plants account for 2/3 of total desalination plants in the Middle East and North Africa (MENA) region for freshwater production. The remaining desalination plants rely on Natural gas to operate RO in the region [11]. The excess amount of Solar and Wind energy potential is present in MENA region compared to Europe, which is abundant with geothermal energy. Solar leads the way in terms of easy access and availability in MENA region [12]. Water desalination is a better option for people of MENA region as 50% population lives nearby coast that makes them accessible of sea water. Combining sea water desalination with solar energy technologies can make best outcome for sustainable water production [13]. Solar assisted desalination remains a viable solution for meeting the growing water demands, yet some technical hurdles needs to be addressed for the commercialization [14].
The current study is being conducted to extensively review desalination techniques powered by renewable energy resources, particularly power input by solar energy. Thus, this review will provide a deep insight into various solar desalination techniques and their effectiveness, focusing on the MENA region. Moreover, it can help develop background knowledge to design an economically feasible desalination system to cope with continuously rising water scarcity issues.
One can achieve desalination using solar energy either directly or indirectly, depending on the technique adopted for solar energy harnessing [15]. Direct solar desalination does not require a large step up of auxiliaries to convert solar thermal energy or electricity from solar panels into other forms of energy. Indirect solar desalination first collects the solar energy and then converts it into another energy form used in desalination [7]. In the current world scenario of climate change and global warming concerns, Photovoltaics (PV) and concentrated solar power (CSP) are the attractive energy source for the desalination of water [16]. Usually, desalination processes are selected based on feed water quality, product purity, climatic conditions, environmental impacts, and, most importantly, site specifications [17]. Figure 1 shows the link between solar energy type and different desalination processes.
Solar energy linked with desalination processes
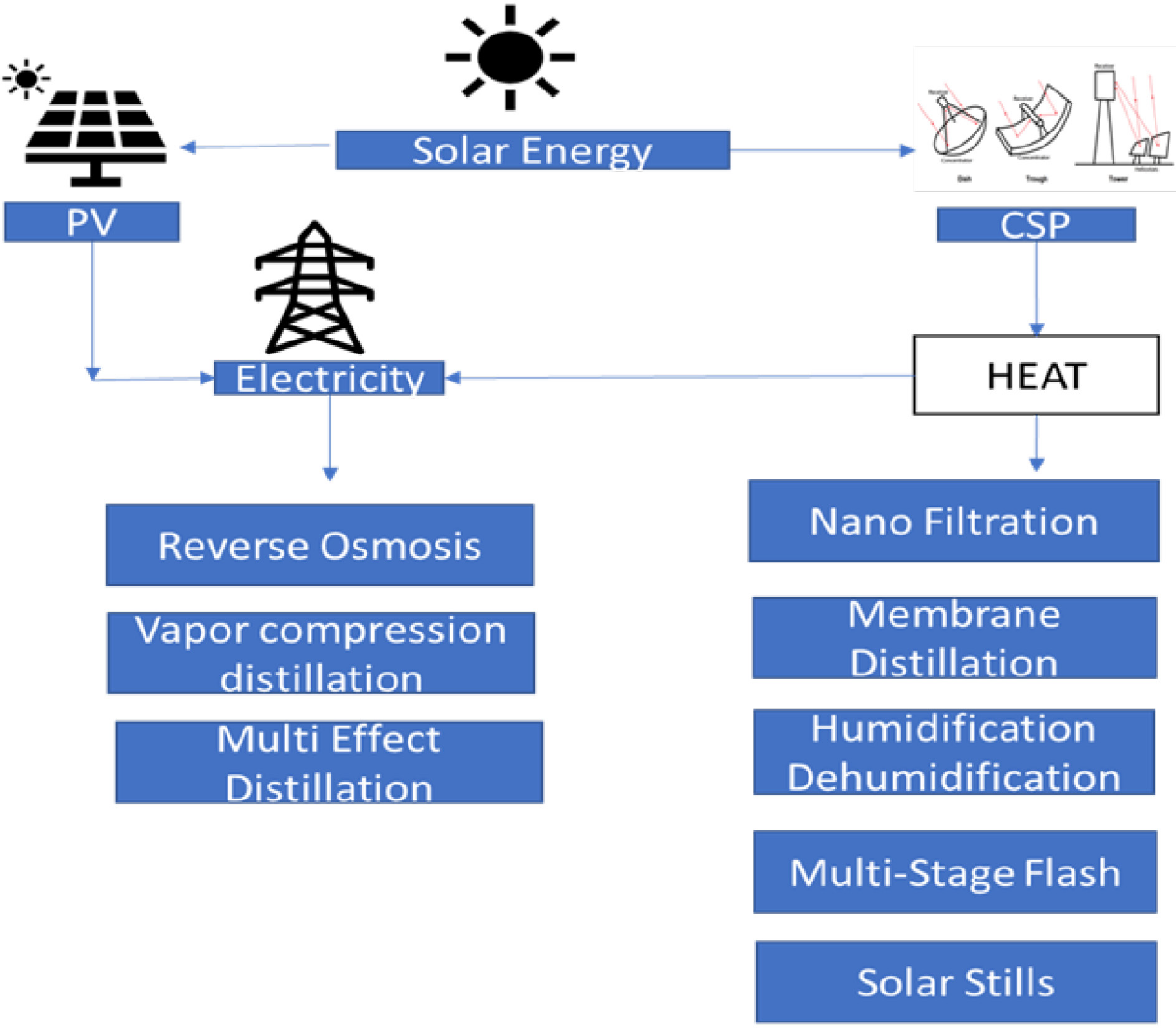
Harvesting solar energy is an attractive proposition for the future [18]. It can be proven to be an effective strategy to power Reverse Osmosis (RO), Vapour Compression Distillation (VCD), and Multi-Effect Distillation (MED) to run auxiliaries (compressors, pumps, heat exchange, and chillers) for the process of desalination. These requirements can either be fulfilled by electricity from PV modules or electricity produced by CSP. The second type of solar energy is heat which is also required in most desalination processes. These processes supply heat to water, and vapour from water is separated in these desalination techniques. The energy to generate water vapour can be collected directly from the heat generated by a CSP setup. It does not require additional cycles and costs to convert heat into electricity. Research currently focuses on improving the solar-based desalination process to convert brackish and seawater water into freshwater.
Two main solar systems are used for membrane-based desalination: PV and CSP. PV-solar-based desalination provides electricity for the membrane desalination (MD) process and auxiliary units. MD systems are suitable for brackish water treatment. Solar configuration coupled with the MD process is used in most areas, but it has a small capacity for water production. The most appropriate solar energy choice for a solar desalination system is CSP because of backup energy and working hours after sunset. The CSP produces electricity and connects with RO System. It also has some limitations, such as in cloudy weather, the direct irradiance reduces, and it only uses direct irradiance from the sun. Due to relatively high humidity in coastal areas, direct irradiance is disturbed. On the other hand, PV has no limitations and can be used on a large-scale system. PV systems are suitable for highly densely populated areas where desalination can use solar energy [19], [20], [21], [22].
According to Li et al., selecting a solar desalination system has some requirements that need consideration before installation. These requirements are a type of solar system, operating conditions, energy demands, and plant configurations. The desalination system selection to couple with the solar system also needs some design considerations that are Capital Expenditures (CAPEX), Operation, Maintenance and Operating Expenditures (OPEX), water quality and clean water requirement, energy demands and site restrictions [14].
Economic analysis is a very important part of installing a desalination plant. For small-scale desalination plants, solar stills, multi-effect evaporation, and RO are suitable depending on land availability. Mechanical vapour compression (MVC) is suitable for a medium-scale desalination plant. Multistage flash (MSF) and multi-effect evaporation (MEE) are dual-purpose distillation methods most suitable for large-scale plants. In remote areas, the best alternative in comparison to other technologies is RO solar desalination plant (SDP). Prototypes of Solar energy from PV for desalination have shown satisfactory results. Solar-assisted RO desalination systems coupled with PV solar collectors are more expensive than other membrane desalination systems [24], [25], [26].
Colombo and co-researchers [26] claimed 25% additional cost for PV solar collectors for MD. Some researchers [28], [29] claimed that the CSP system combined with the RO station is the best and most efficient system for solar desalination. MD system has a better flux rate than solar stills to produce freshwater. The overall efficiency depends on the irradiation intensity responsible for increasing the brine temperature, which helps in the process. SDP has lower thermal efficiency, but it is suitable for small-scale production. Coupling a small-scale desalination system with a PV system neutralizes this drawback in a stand-alone system [30], [31].
Desalination systems need a constant supply of energy to operate the process continuously. In conventional systems, energy is obtained by burning fossil fuels that emit greenhouse gases (GHG), causing environmental concerns and enhancing the cost of the desalination process. Renewable energy resources utilization for energy is getting recognized worldwide to overcome these concerns; typical sources are solar, wind, and hydroelectric power plants. In particular, solar energy to run a desalination system is getting more attention and is considered here. Solar desalination systems use direct solar thermal heating and PV solar energy; these two options and their important parameters are discussed in this review paper.
Solar stills are cost-competitive for small-scale desalination plants compared to other desalination methods [31]. Solar stills work on the principle of saline water evaporation by utilising direct sun radiation [32]. A still has a glass cover and is insulated from all sides. The brine water is fed into the basin of the still. The glass cover enables solar radiation to enter the still and heat the brine water directly. Then evaporation of water starts and vapour rises upwards, trapped by the glass cover. This vapour condenses on the inside surface of the glass cover and is collected in another tank as freshwater. Solar stills are simple, reliable and low-productivity devices [33]. The typical productivity of solar stills is less than 4 kg/m2 day; thus, the installation area increases with the desalination production capacity increase [34]. A simplified solar still mechanism is shown in Figure 2.
Solar still diagram [39]
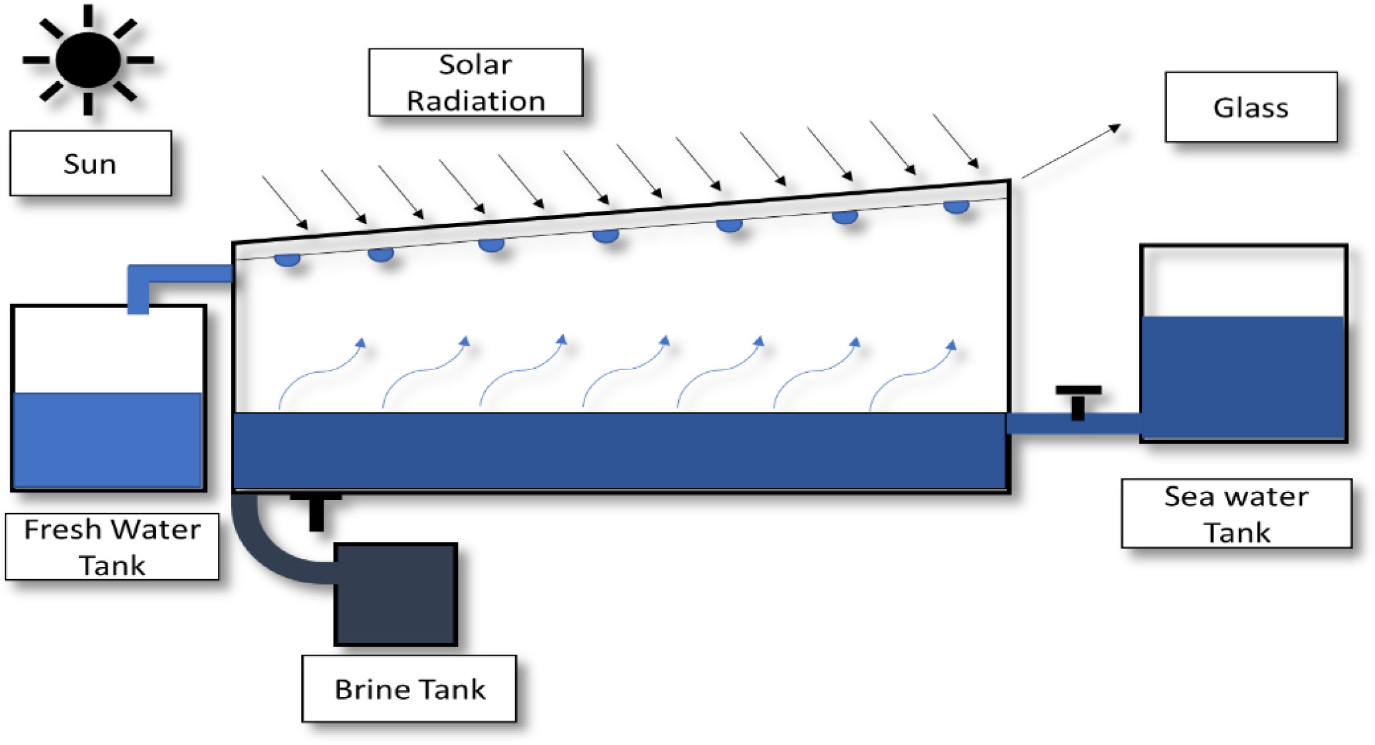
Several factors affect a solar still's evaporation rate and performance [34]. Among them, direct solar radiation intensity plays a crucial role in improving the efficiency of Solar Stills. The early afternoon has the maximum distillate production rate as it reports the highest solar radiation intensity [35]. The still's exposure area or active area is related to the evaporation rate. As the water basin area increases in solar still, the distillate production increases. Another factor is water bath depth, which can also hinder still performance [36]. The deeper the water bath in the solar still, the lesser its distillate product; thus, the daily production of the still can be increased by reducing the water basin depth [36].
The latitude angle and tilt of solar still can enhance the still productivity. However, it depends on the installation's location to predict the optimum angles for enhanced output. The main challenge for the solar still has been the low productivity per unit installation area. It can be enhanced by optimizing and tuning various parameters, including design configuration, glass thickness, light transmittance, cover slop, still geometry, brine depth, and heat transfer management inside the solar still [37]. Sensible- and latent-heat storage materials can be integrated with solar-still desalination for utilizing the energy in the off-sunshine hours or cloudy weather. Moreover, nanofluids can be adopted to increase the thermal conductivity of water, causing an increase in the absorption rate of solar radiation that will further enhance solar still productivity. An experimental study reported that using the nanofluids enhanced the performance of solar still integrated with external condenser by about 116% [38].
The glass cover thickness is also very important, as it is the major provider of incoming radiation, affecting the heat transfer rate. It is reported that a 3 mm thickness of glass cover plate gives the highest production rate of 15.5%, and an increase in this thickness reduces the overall production [40]. The heat transfer between glass and atmosphere occurs by convection and depends on wind velocity. Increased wind velocity produces more distillate by improving condensation [39]. Condensation surfaces also increase water production from stills up to 20% [41]. The amount of distillate produced by solar still is about 5 L/m2 day, which is lesser than the water demand of a person in a day [42]. To fulfil this demand, the yield of solar stills needs to be enhanced by modification and increasing the still active area [43]. Conventional solar stills have less productivity than solar stills with vertical and inclined fins, as a larger convection heat transfer area enhances production [44]. The water production rate in solar stills can increase by cooling the glass cover. Using solar stills incorporated with PV can increase thermal and electrical efficiency. Single-slope still running on thermoelectric heating and cooling can be the most efficient technique for water production [45]. Another suitable approach to enhance solar still productivity is to enter the hot air; results showed that daily freshwater productivity had reached approximately 9.36 kg/m2 for modified still while the value of conventional solar still is about 4.5 kg/m2 [46].
In Solar Humidification-Dehumidification Desalination (SHDD), hot air is the process medium. A solar air heater enters the humidifier and transfers heat to hot seawater, also heated by a solar water heater [47]. The temperature and humidity of air increase with the contact time. The moist air enters the dehumidifier, where the water is separated from the air by condensation and collected as desalinated water [48]. The dehumidifier consists of a condenser in which cold saline water circulates in the coil, working as the heat-exchanging fluid. The supplied heat is later used to preheat the feed water in the humidifier [49]. A scheme of the SHDD system is shown in Figure 3.
Solar humidification-dehumidification desalination process [53]
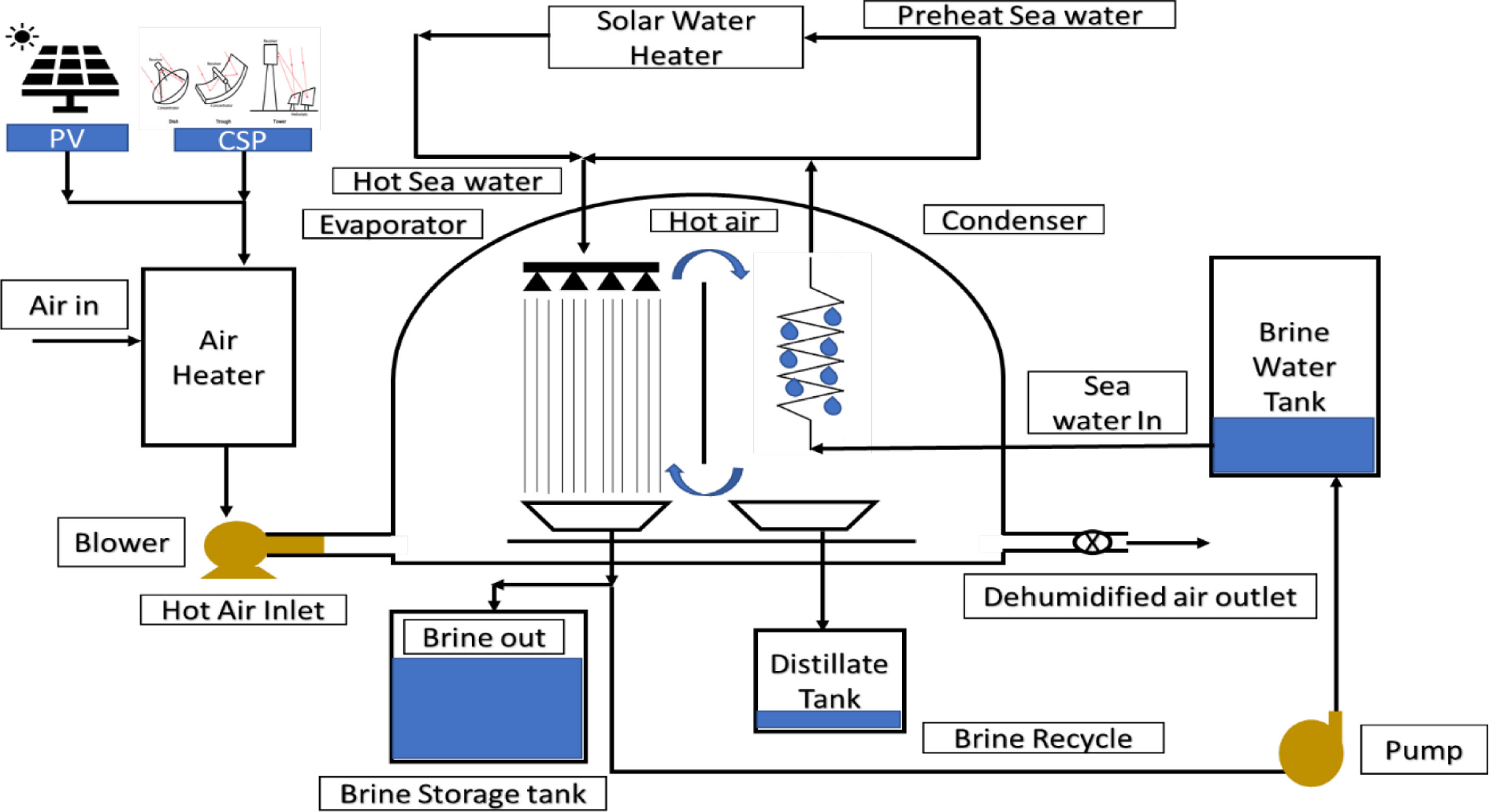
For seawater desalination, humidification-dehumidification is very important as it runs stably with an easy control system and no or low pre-treatment requirements. At the same time, the operation takes place at atmospheric pressure with no scaling and low maintenance. If incorporated with solar energy, it is more efficient than solar still technology [50]. Solar energy incorporation into humidification-dehumidification is most suitable for remote areas and small-scale desalination applications. An SHDD desalination unit has a higher water production cost than fossil-fuel desalination units but is more environmentally benign as it produces less GHG emissions [51]. Other benefits of SHDD include working under normal pressure, lower cost of the assets, low pre-treatment required for desalination, scale-up flexibility and simple working equipment [52]. Thus, the use of hot air supply in solar still significantly improves the evaporation rate, leading to higher distilled productivity.
The productivity of an SHDD unit is higher in summer. Air humidity is the significant process parameter, as 1 kg of dry air can carry 0.5 kg of vapour and about 670 kcal (2803 kJ) when its temperature increases from 30 °C to 80 °C [54]. Moreover, it is reported that the desalinated water produced from a humidification-dehumidification unit costs 0.032–0.038 USD/L [55]. The humidity and inlet temperature of air should be considered carefully to enhance the performance of SHDD process [56].
Solar multistage flash desalination (SMSFD) works as the seawater is heated above saturation temperature and enters the flash tanks, where low pressure is maintained by the vacuum pump [57]. The first flash tank has the highest pressure, and the last tank has the lowest pressure. The pressure drops facilitate the flow of brine along the flash tanks. The distillate tray in each flash tank collects the flashed-off vapour in the tank which collects it as the final water product. The condenser in each tank works as the preheater for feed water. The heating system of the process is facilitated by solar power [58]. A simple SMSFD unit is schematically shown in Figure 4. The conventional multistage flash process runs on fossil fuel to produce desalinated water. Multistage flash requires more energy than other desalination technologies but is reliable and can be applied in large-scale plants. Water production cost and capital investment of an SMSFD unit were 3–10 times higher than the conventional desalination method using solar still for the same plant capacity [59]. The seawater or brine temperature rises to 90–120 °C. The multistage flash unit technique depends upon distillate requirement, thermal storage present, water salinity, brine heater's fouling resistance and solar technology [60].
Solar-powered multistage flash desalination system [53]
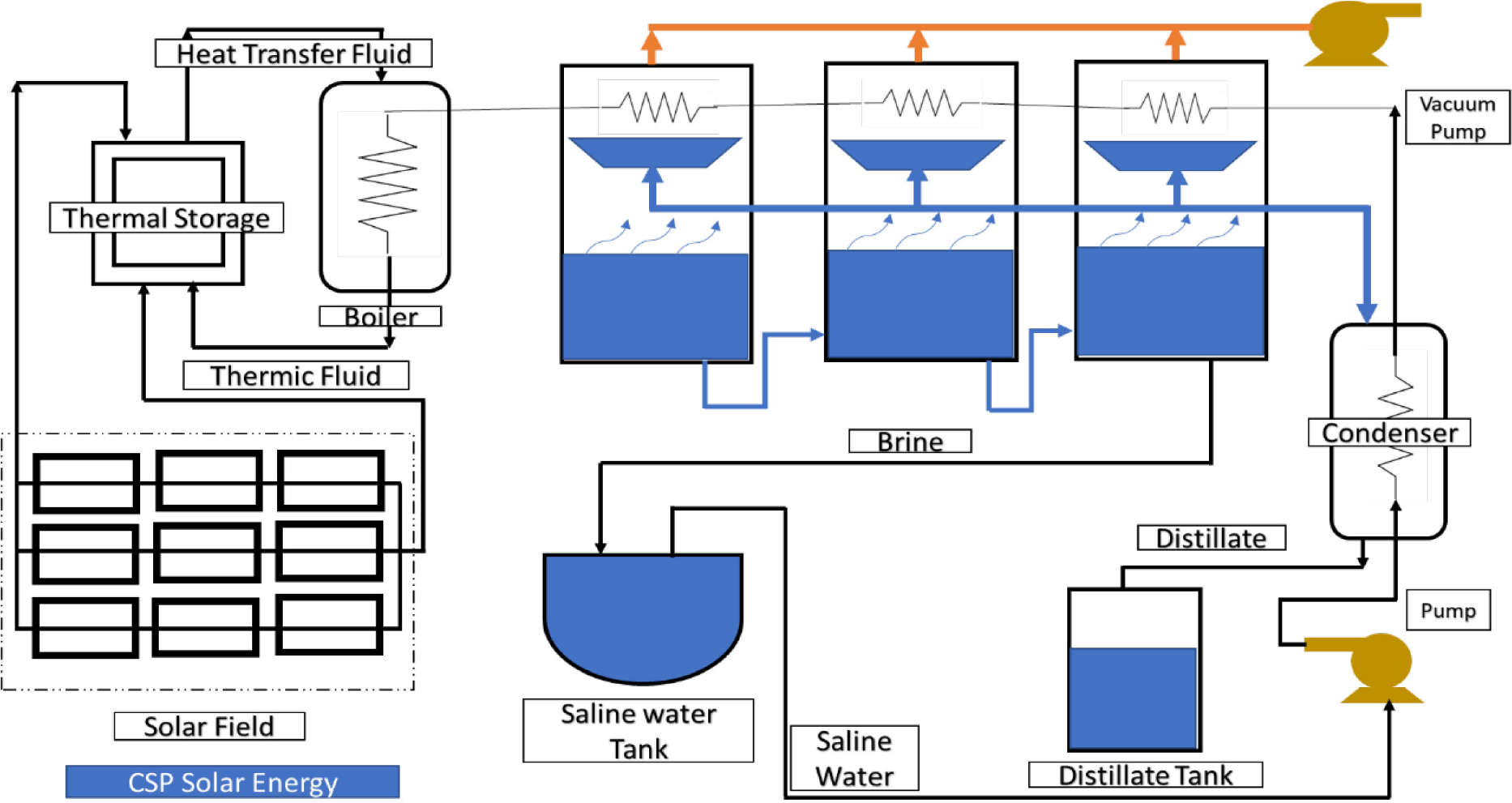
Sometimes a medium or heat exchanger is used to raise the brine's temperature, and other techniques use solar arrays to circulate and heat the brine. The water production from brine depends upon the amount of water required, which dictates whether a plant will work only in daylight hours or in the evening as well with the help of thermal storage. The amount of water produced from a multistage flash varies between 10–60 L/m2 per day, depending on the solar radiation available and system efficiency [61].
Around the world, multistage flash is more widely used than other desalination techniques. Arab countries have also done 80% of their desalination using multistage desalination. Around 86.7% of installed desalination plants in the MENA region apply multistage flash because these systems can produce water and electricity when installed with a power plant. Multistage flash plants have a long life, produce the best water product, and require minimum maintenance while avoiding the pre-treatment of brine and high-temperature operation [62]. Brine temperature control is needed for stable operation when solar technology is applied in multistage flash for heat requirements. It is reported that 81–106 kWh/m3 of specific energy is required for a solar-powered multistage flash when a 10–45 °C temperature difference between inlet seawater and brine is present [63]. If a multistage flash is operating at 40% efficiency producing 1000 m3/day of water, and the solar technology applied costs around 100 USD/m2, the water production cost will be 0.97 USD/m3 [64].
The most important commercial desalination plants in the MENA region are multistage flash desalination. The majority of these are operated by the electricity produced from fossil fuels. Solar technology incorporated with multistage flash is the need of the hour as it will help in sustainable water production and develop this region socioeconomically [65].
Solar-powered multi-effect distillation desalination (SMEDD) system works with low-pressured vessels spraying brine from the top. The brine converts into vapour in the first vessel by heating. The vapour enters the second chamber and acts as a heat source, producing more vapour in the second chamber. This way, latent heat of vaporization is utilized to heat more brine solution. A solar thermal system can do the heating of brine. Additional energy for pump and vacuum can be collected from solar PV or thermal power generation. This system is more compatible with solar thermal desalination [66]. The water production cost from solar-powered SMEDD is comparable to the conventional desalination plant, which depends on salt cost and can decrease by increasing solar pond area. Although the system does not need feedwater pre-treatment and its GHG emission is less than SMSFD, corrosion risk and high energy demand make it less acceptable [57]. A typical schematic of SMEDD is shown in Figure 5. The performance evaluation of a SMEDD distiller with a freshwater capacity of 3 m3/day showed that the distiller uses about 40 kW heating and 35 kW cooling [67].
Solar-powered multi-effect distillation system [53]
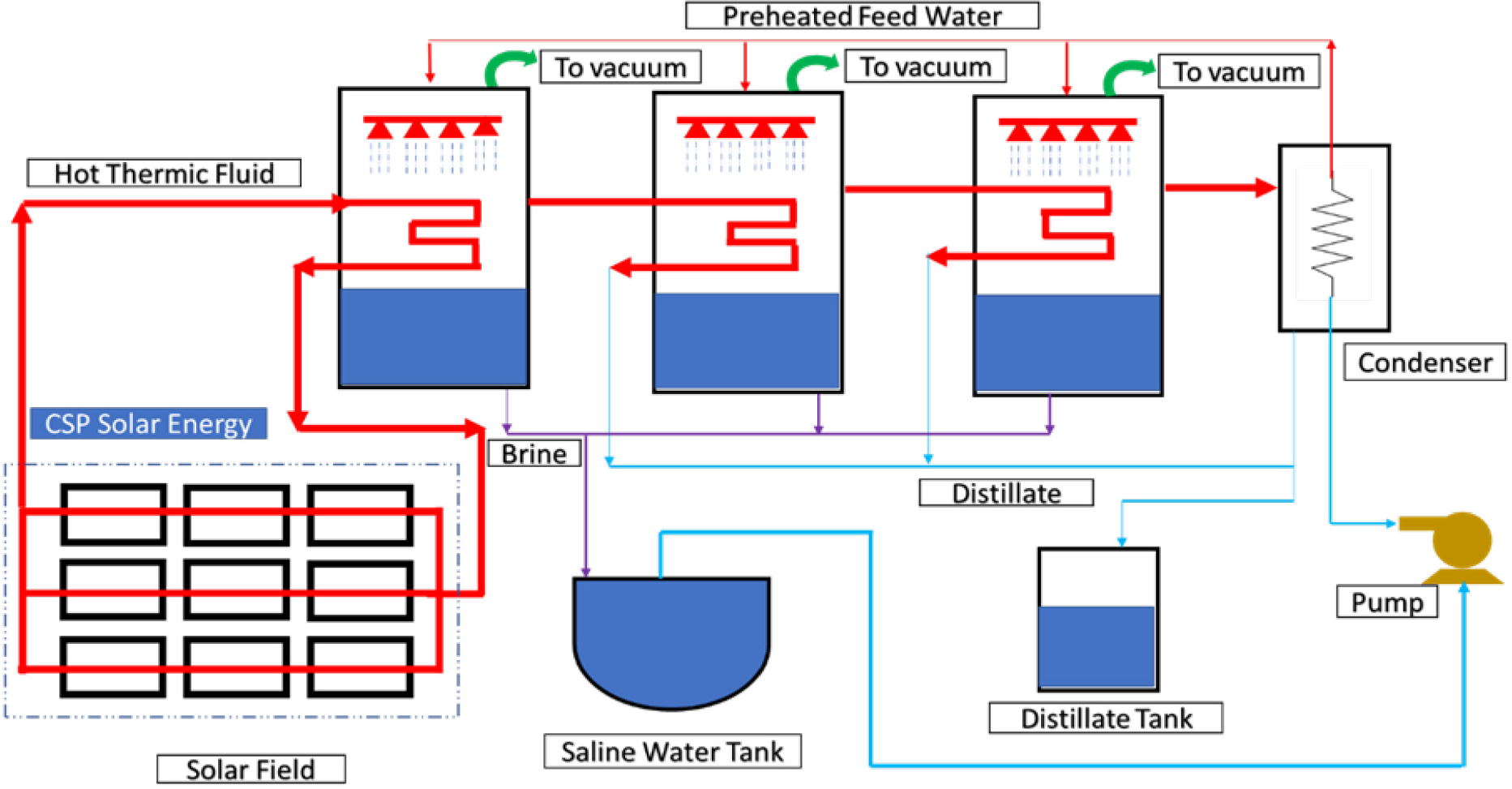
For large-scale applications, SMEDD is the most appropriate technology for solar desalination. Multi-effect distillation has a low levelized cost of water, efficient heat-transfer and better thermodynamic characteristics; however, RO systems have better equivalent electrical energy than any other desalination system. Research should be done to find the best scheme to couple multi-effect distillation with solar energy [68]. The multi-effect distillation requires a lower top brine temperature, making it appropriate to utilize low-temperature heat sources. Renewable energy systems can provide low-grade heat. For a solar-powered distillation, modification in plant layout will reduce cost and energy requirements because multi-effect can use steam of 70 °C at 0.3 bar while multistage flash uses steam at 180 °C at 3 bar. Multi-effect distillation units coupled with solar energy can enhance global water production efficiency through desalination [69]. Energy requirements of multi-effect distillation are lower than multi-stage flash [70]. Multi-effect distillation unit can also produce less costly water compared with a multi-stage flash because it can use steam of 70 °C at 0.3 bar, while a multi-stage flash uses steam at 180 °C at 3 bar [68]. The number of effects required in multi-effect distillation depends upon available heat exchanging area, water product required, distillate to steam ratio, capital cost and temperature difference between seawater and steam source [71].
In a mechanical vapour compression (MVC) system, the feed water is heated externally with solar energy. The hot brine is flashed in the vessels, and the flash vapour is compressed, resulting in increased condensation pressure and temperature. The compressed vapour is used to increase the brine temperature in the same stage or the next stage. MVC desalination units can be powered by a hybrid PV/diesel system; however, the hybrid system requires higher power input and generates a higher carbon footprint [57]. Solar PV is a better alternative in this desalination system than conventional electricity, although renewable energy can also provide external power such as wind, hydrothermal or geothermal [72]. Solar-powered mechanical vapour compression desalination (SMVC) is schematically shown in Figure 6.
Solar-powered mechanical vapour compression desalination system
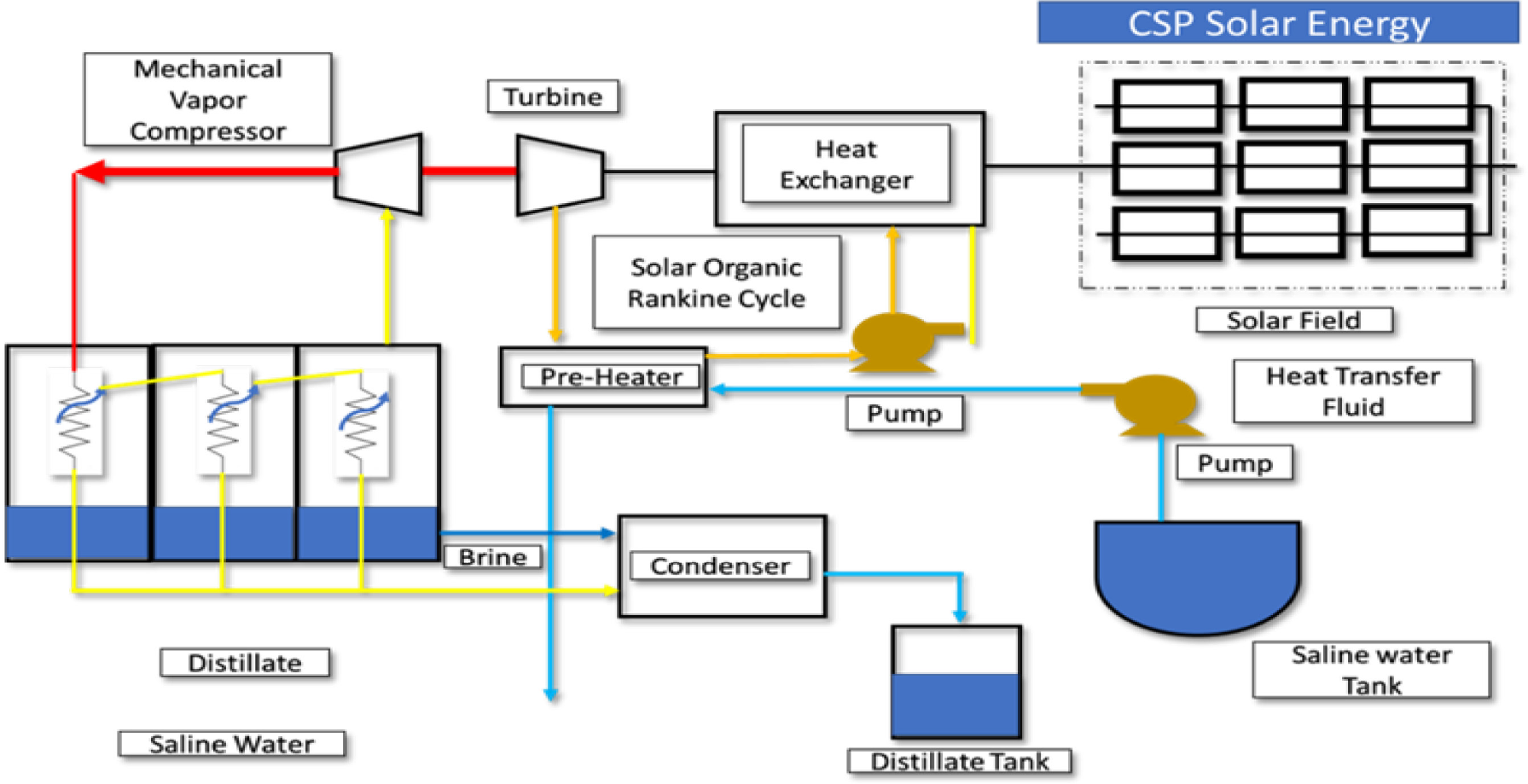
A large-scale SMVC plant has a cost comparable to a conventional system. If the plant capacity is more than 120 m3/day, one can reduce water production costs by coupling it with solar PV [73]. A hybrid system is also capable of reducing CO2 emission by 179 t/year. This system is highly efficient and environmentally friendly, but the corrosion risk in the compressor, low capacity, high capital and water production cost makes it less feasible [57]. For a small-scale application such as 5000 m3/day of water production from desalination, MVC is most suitable. The small MVC recovers heat after partial compression from the produced vapour; moreover, it is compact, flexible in operation, requires less power for pumping, and achieves a high heat transfer coefficient. MVC has some limitations as it requires electricity to run, thus not being suitable for remote applications. It also needs a large vacuum and evaporator due to the top brine temperature of 60–70 °C, which increases the capital and operational cost [74]. Although, MVC does not depend upon external heat supply, which facilitates a reliable and stable operation [75].
Membrane distillation occurs by water penetration through a porous hydrophobic membrane that separates the saline water from the product water. The most widely used membrane materials are inert, hydrophobic, low-thermal-conductance polymers, including polyvinylidene difluoride (PVDF), polytetrafluoroethylene (PTFE), and polypropylene (PP) [76].
The hot saline water enters the system. The hot feed water passes through the membrane due to the pressure difference between the membrane surfaces. Sweeping-gas, air-gap, direct-contact and vacuum-membrane distillations are different types of this process. The distillate (product water) is collected on the other side of the membrane. Solar Membrane Desalination (SMD) process yield can be determined by feed water temperature, membrane thickness, membrane thermal conductivity and porosity, and long-term operation. Expensive membranes increase the cost of this system. Low operation temperature and efficient product yield make this system compatible with a small-scale capacity [57]. A typical layout of the SMD system is shown in Figure 7.
Solar-powered membrane distillation [57]
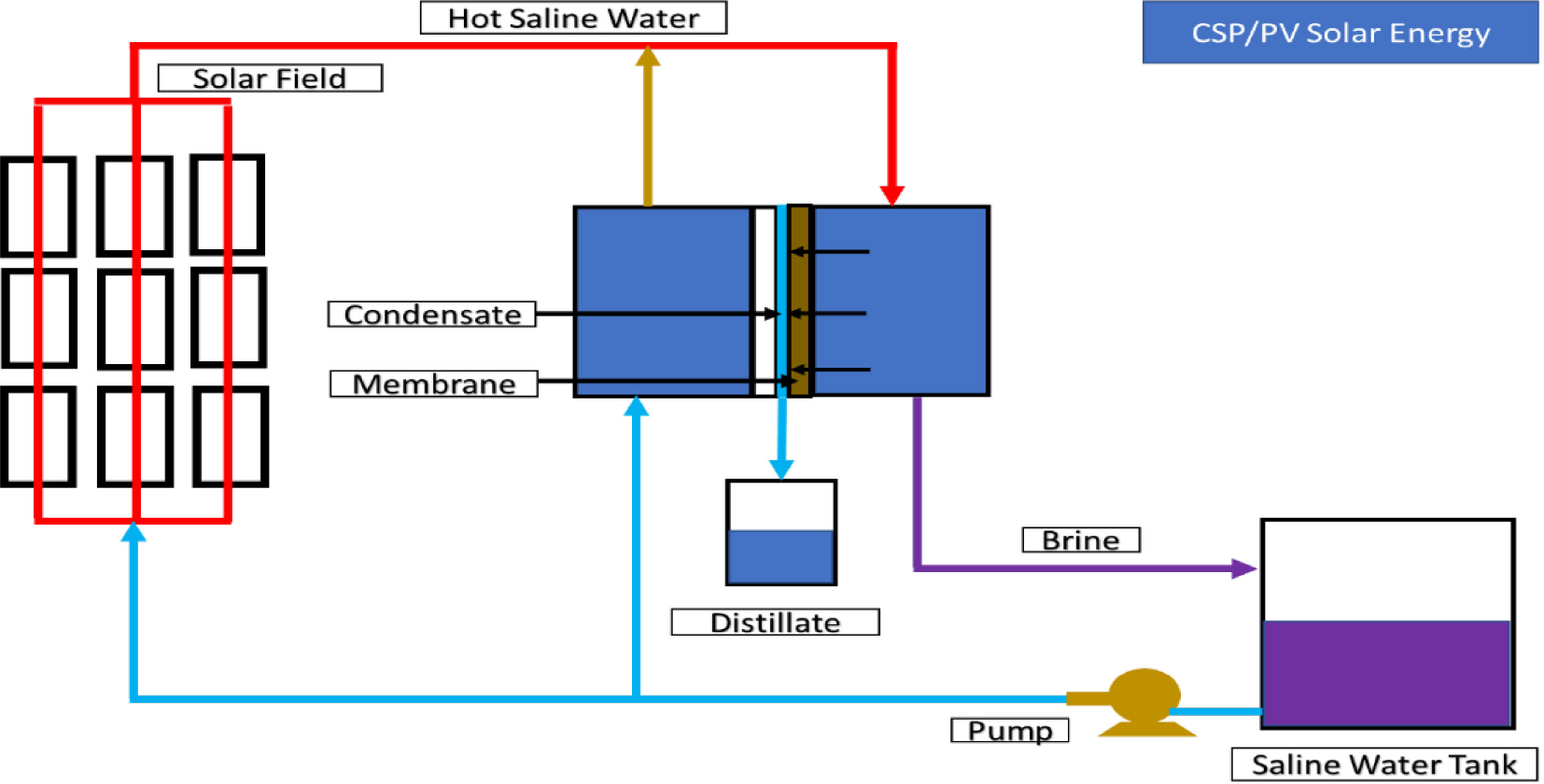
As the energy requirement of membrane distillation does not depend on water salinity, this technology is suitable for all kinds of saline water desalination. On the other hand, an SMD system can be easily damaged by inorganic material that attaches to the membrane surface as fouling or scaling. Maintaining the pre-treatment of saline water for inorganic compounds is necessary before it enters into contact with the membrane [77].
In the solar-driven Rankine cycle of RO, the solar energy is stored in the form of heat in thermal storage. This stored energy is used to heat boiler fluid. The boiler fluid runs the turbine, which produces power to run the high-pressure pump. The pump provides high-pressure feed for the RO unit to obtain freshwater product and concentrated brine. Membrane degradation by biological fouling and the short lifetime of the membrane makes the system even more costly. Despite these problems, it is easily expandable, while low energy requirement and low operation temperature make the process more feasible and acceptable [57]. The RO desalination plant efficiency depends on operational parameters, type of membrane employed and feed water characteristics (seawater or brackish water) [78]. A scheme of the solar-powered reverse osmosis (SRO) system is shown in Figure 8.
Solar organic Rankine cycle powered RO [57]
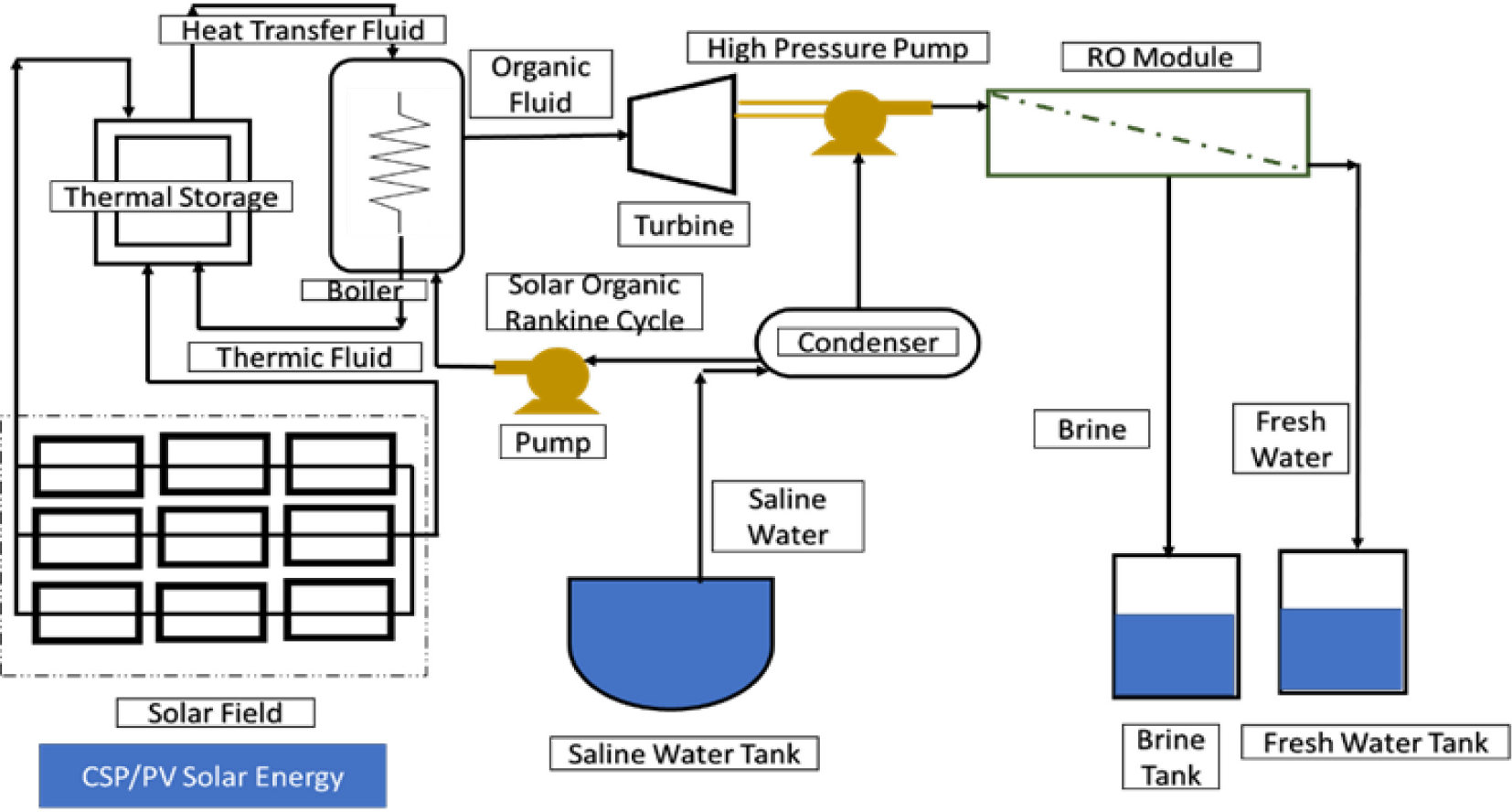
Worldwide 52% of RO desalination plants are powered either by PV modules or CSP, while 40% are PV-RO, 8% PV-wind RO, and 4% Solar Rankine RO [79]. PV panels are used to power a RO plant, but it is impossible to secure a continuous power supply due to the intermittent nature of solar energy [82], [83]. The discontinuities increase SRO plant cost and necessitate numerous start-stop cycles and operation periods with partial load and limited availability of the desalination plant. PV-RO systems are also location- and environment-dependent [11]. The energy requirement of large-scale RO plants is around 5 kWh/m3, therefore much lower than thermally driven desalination techniques [79].
SRO plants can work in small-scale applications with a capacity of 1 m3/day to large-scale plants having a capacity of 500,000 m3/day [82]. Feed water salinity is not restricted in an SRO plant as it can operate with fluctuating water salinities. Reliable and continuous operation without shutting down is also an advantage of SRO plants [83]. The emissions from RO plants range from 1.7 to 2.8 kg CO2/m3 [84].
The literature has shown that the MENA region is facing extreme water scarcity, which is expected to rise in the near future [85]. Currently, water consumption statistics show that the trend is expected to rise many folds by 2050 due to various reasons, including agriculture and industrial usage. The current section will deal with statistics on overall water consumption in the MENA region.
The population, water demands, and per capita water consumption in the MENA region are represented in Table 1. The data show the MENA region's demand forecast and available exploitable water. One can conclude that demand, consumption, and population will increase with time, but water quantity is limited. Alternative freshwater resources are necessary to meet the MENA region's demands in time [88], [89]. Agriculture is the biggest sector to use water resources. It is required to maintain the region's food security while it is already short on adequate water supply [88]. Agricultural demands are responsible for 85% of the water demand in the region, where countries such as Syria and Yemen consume about 90% and 95% of the country's water in the agricultural sector, respectively [89]. While the MENA region's rapid urbanization, economic expansion and water requirements are necessary and need of the time, relevant actions need to be taken to overcome these barriers, particularly for meeting the water needs [90].
Aggregated data for MENA countries until 2050 [86]
Total MENA | Unit | 2000 | 2010 | 2020 | 2030 | 2040 | 2050 |
---|---|---|---|---|---|---|---|
Population | million | 316.4 | 382.0 | 452.0 | 514.5 | 568.5 | 612.2 |
Exploitable water | km3/y | 327.9 | 327.9 | 327.9 | 327.9 | 327.9 | 327.9 |
Sustainable water used | km3/y | 220.2 | 235.2 | 253.9 | 271.9 | 291.5 | 313.8 |
Agriculture demand | km3/y | 237.6 | 265.6 | 293.5 | 313.8 | 327.4 | 334.1 |
Municipal demand | km3/y | 21.2 | 28.7 | 38.4 | 50.0 | 64.1 | 81.2 |
Industrial demand | km3/y | 10.3 | 14.2 | 19.5 | 26.3 | 35.2 | 46.4 |
Total demand MENA | km3/y | 269.1 | 308.5 | 351.4 | 390.1 | 426.7 | 461.7 |
Per capita consumption | m3/cap y | 851 | 808 | 777 | 758 | 751 | 754 |
Compared to the world, the MENA region is still underdeveloped in producing electricity from renewable resources as it has only 2 GW of wind farms installed. MENA region is suitable for solar power and desalination plants as it receives 2000–2800 kWh/m2 y of direct radiation from the sun [91]. Recently, Gulf Cooperation Council (GCC) has adopted renewable energy policies. All the MENA countries have also presented ambitions to adopt clean energy plans and carbon emission reduction [92]. The MENA region from Morocco in northwest Africa to Iran in Southwest Asia has arid and semi-arid climatic conditions and very low water availability. It has less than 1% of the renewable freshwater reserve of the world [91].
On the other hand, its population is about 6% of the world [89]. These countries rely on rainfall, river water coming from other countries and aquifers [88]. Freshwater resources are depleting in Middle Eastern and North African countries. Due to climate change and population growth, freshwater demand in these regions will increase in the future, and the limited resources will be unable to meet the water demands. Brackish and seawater desalination in these regions can facilitate future water demands. Annual water demand for this region, total unmet demand and water resources under average climate change scenario are represented in Figure 9, Figure 10 and Figure 11.
Water demand of MENA region from 2020-2050 [93]
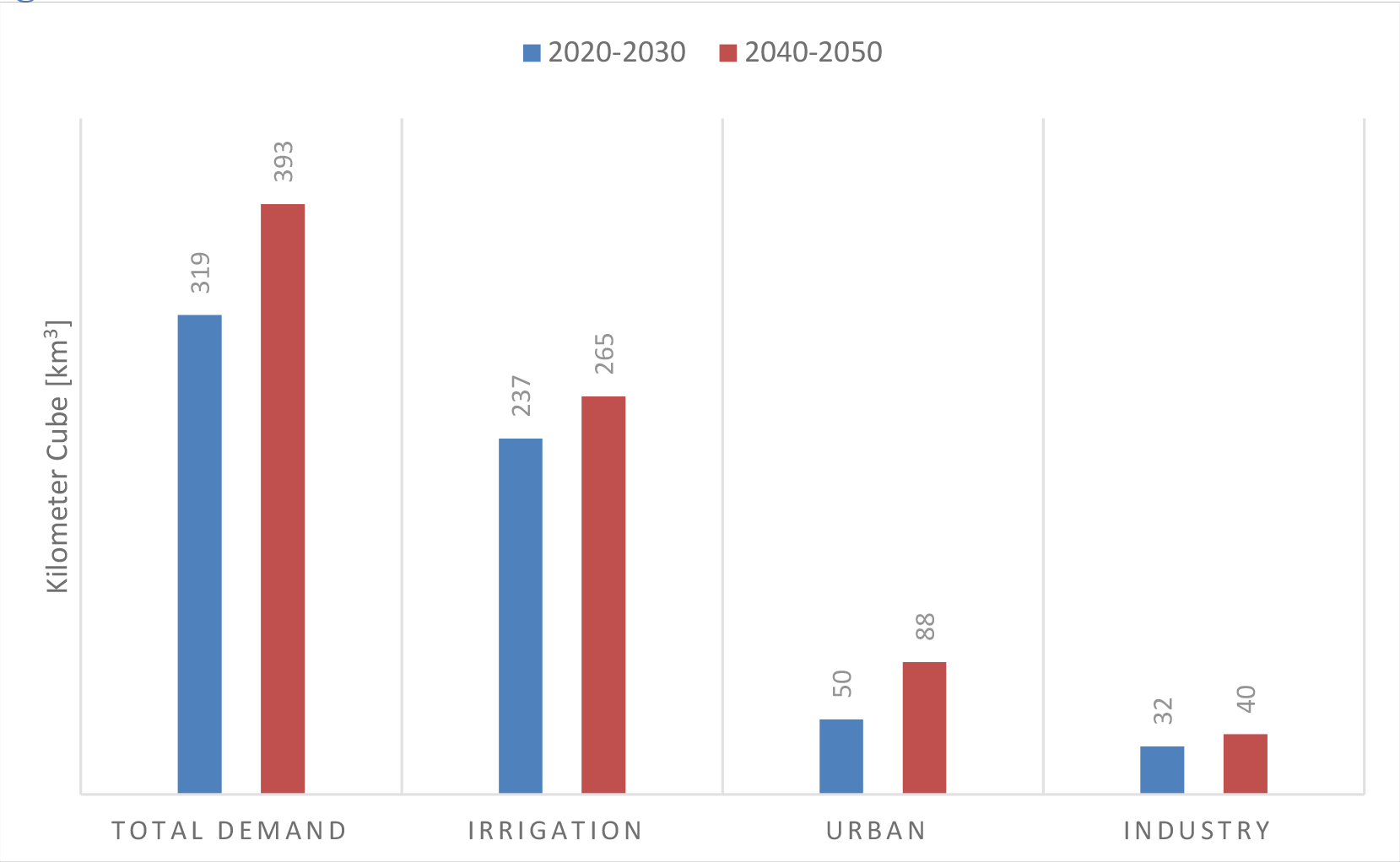
Water supply of MENA region from 2020 to 2050 [93]
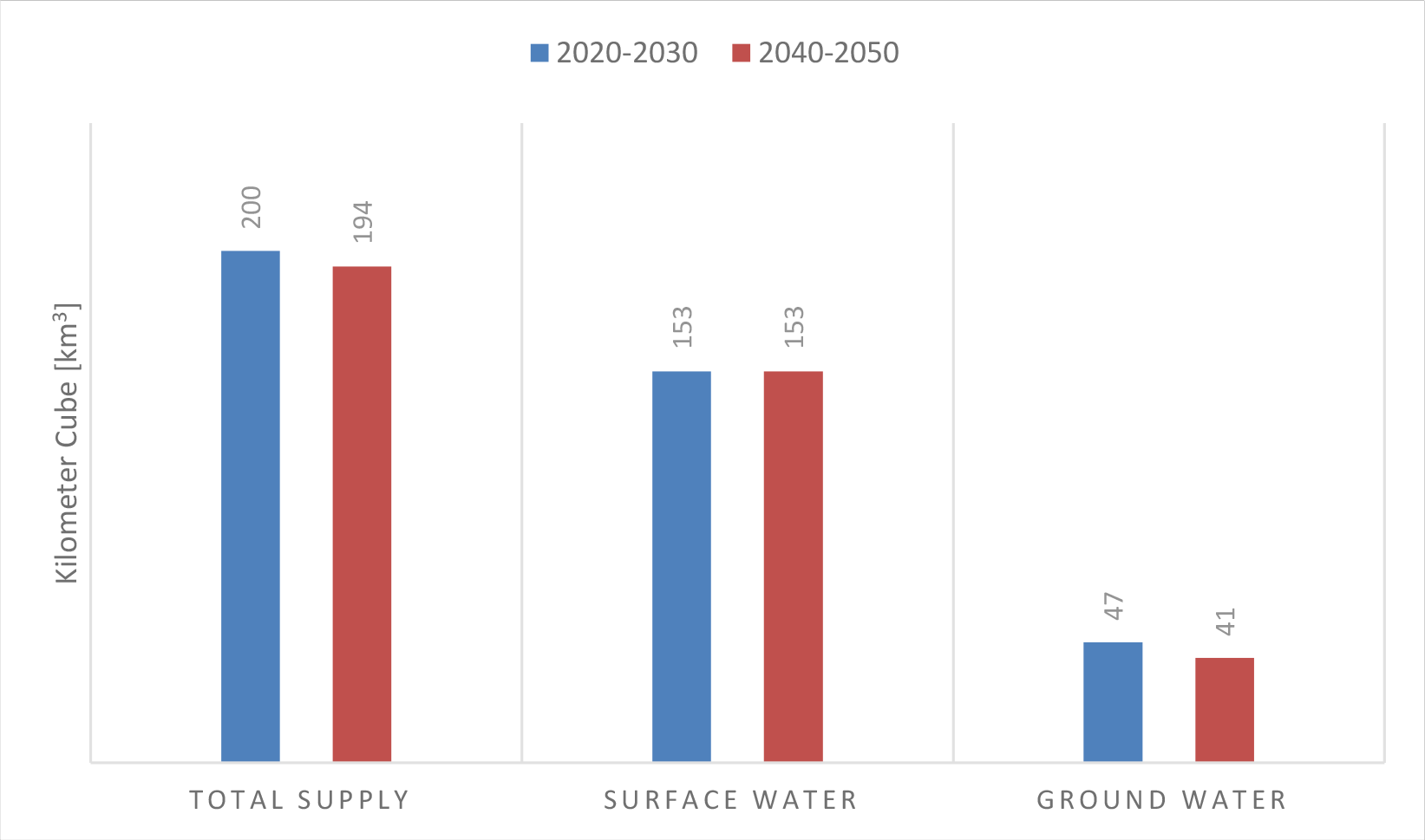
Unmet water demand of MENA region from 2020 to 2050 [93]
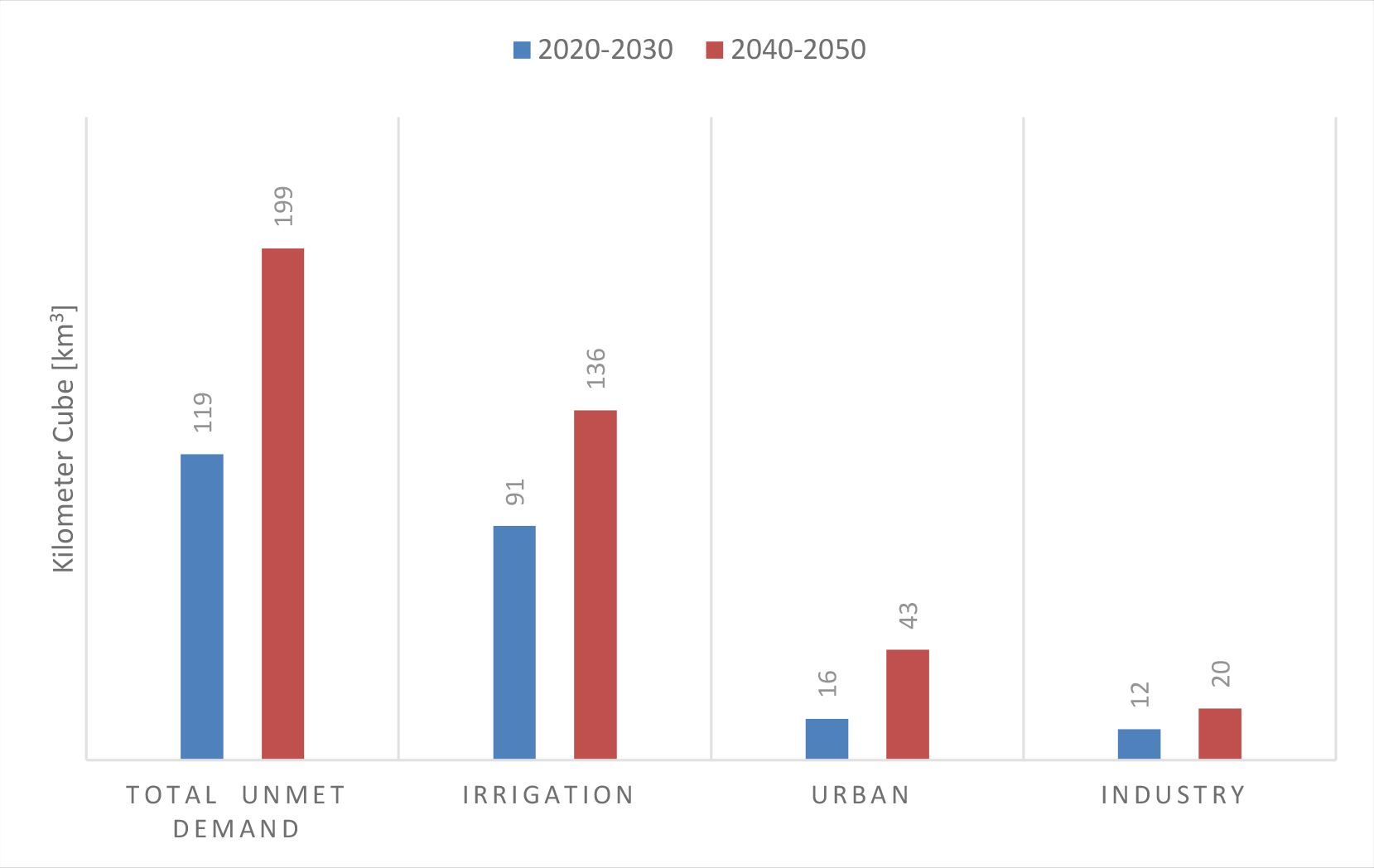
The statistics show that the demands will rapidly increase in the upcoming years, the water resources will deplete, and unmet water supply demands will also increase in different sectors of life. Some critical measures are required to tackle these demands and supply gaps; otherwise, bigger problems would also arise. That would not only disturb the region's economy, but disasters and many causalities could also be expected if no serious actions are taken against the water crisis. Climate change is also a big factor in the exhaustion of freshwater resources and excessive use of water in daily life [93].
MENA region has very small renewable water resources available [94]. Renewable Water availability of 1400 m3/cap in the MENA region is much lower than the corresponding global statistics. The region's countries consume 80% of their available water [89]. Many of them, such as Yemen, Jordan, Libya, Algeria, and Palestine, are experiencing an acute water shortage (less than 500 m3/cap y).
MENA region has almost half (48%) of the global desalination capacity, which is with Saudi Arabia (15.5%), UAE (10.1%) and Kuwait (3.7%). Brine production in the MENA region is also 70.3% of global production; this is double the amount of desalinated water produced, indicating that the plants in this region work at a very low average water recovery ratio of 0.25. The total number of desalination plants worldwide is 15,096, with a capacity of 95.37 million m3/day and brine production of 141.4 million m3/day. MENA region has 4826 desalination plans installed; their capacity is 45.32 million m3/day with brine production of 99.4 million m3/day [95].
The SDPs operated in the MENA region are still small-scale in remote locations [96]. Because of their high capital and water production cost, these plants have been constructed for research and demonstration purposes. The data on some existing PV-SDPs in the MENA region, including their desalination technology and production scale, are shown in Table 2, Table 3 and Table 4 [97].
Existing PV-RO desalination plants in the MENA region [97]
Location | Year | Additional Power Supply | Production [m3/day] | Operator |
---|---|---|---|---|
Abu Dhabi, UAE | 2008 | Diesel | 20 | NEWRC |
GECOL, at Ras Ejder, Libya | 2005 | Wind & grid | 300 | GECOL |
El Hamrawein, Egypt | 1986 | – | 240 | - |
Hassi Khebi, Algeria | 1988 | – | 22.8 | - |
Sadous Riyadh Region, KSA | 2001 | – | 14.4 | - |
Maagan Michael, Israel | 1997 | Diesel | 9.6 | - |
Aqaba, Jordan | 2004 | – | 81.6 | NERC |
Ksar Ghilane, Tunisia | 2006 | – | 50.4 | ITC |
Benhsaine, Morocco | 2007 | – | 24 | ITC |
Msaim, Morocco | 2007 | – | 24 | ITC |
Jordan Valley, Jordan | 2010 | – | 30 | - |
Tasekra, Morocco | 2008 | – | 24 | - |
Existing solar membrane distillation desalination plants in the MENA [97]
Location | Year | Additional Power Supply | Production [L/day] | Operator |
---|---|---|---|---|
Morocco | 2005 | – | 150 | Fraunhofer ISE |
Aqaba, Jordan | 2005 | – | 1000 | Fraunhofer ISE |
Existing solar CSP multi-effect distillation desalination plants in the MENA [97]
Location | Year | Additional Power Supply | Production [m3/day] | Operator |
---|---|---|---|---|
Qatar | 2012 | – | 150 | Fischer |
Alexandria, Egypt | 2018 | – | 250 | Fraunhofer ISE |
The cost of a desalination plant in this region varies depending on multiple factors, including the type of system, quality of water, quality of rejected water, location, and plant size. This cost also includes the pre- and post-treatment of water in the region, depending on the containments in the water. However, as the focus is shifting towards alternative and better sustainable options to desalinate seawater, the cost of these plants is also rapidly increasing, making the systems more demanding and increasing the water production cost. Still, capital costs for conventional and renewable desalination systems are the same. Operational cost also varies depending on several factors, such as transportation and site conditions [98]. The total cost of a desalinating plant can range from 1.06–1.59 USD/m3 depending on energy technology, plant location and energy cost [93]. For the cost of the desalination system, industrial studies have shown the costs of SMEDD and SMSFD are 1200–1500 USD/m3 day and 900–1500 USD/m3 day, respectively. PV-RO costs 700–900 USD/m3 day. The efficiencies of SMEDD and SMSFD are 60–70% and 60–70%, respectively. PV-RO has 15–20% efficiency. Combining these systems’ costs and efficiencies will give a better look at these technologies’ actual water production costs. SMEDD and SMSFD will have the actual water production cost of 1430 USD/m3 day and 2140 USD/m3 day. PV-RO will have 4500 USD/m3 day, the highest water production cost among all [99].
The countries in the MENA region have an abundance of seawater and solar energy. It makes solar desalination plants viable for small- and large-scale freshwater production. Several other factors affect the application of new technology. First is the water resource: in the case of seawater desalination, neither solar energy nor seawater is limited or will be limited. Secondly, one must consider the capacity of the production units, annual water demand, replacement of old plants and product cost comparison to other technologies. All these factors play a very important role in the commissioning and installation of desalination plants. Every country in the MENA region must consider these parameters for economic and feasibility analysis.
Several studies have been done on desalination plants in the countries of this region. These studies have analysed economic and environmental factors and problems in the project commissioning. There are different hurdles in every part of the region, which make the direct application of these projects unacceptable. Some of these studies in the main MENA countries are discussed below, highlighting the drawbacks and problems in project commissioning and application.
MENA region is mainly consisting of sunny areas. Renewable energy, i.e., solar and wind energy, have the potential to provide future energy demands for MENA countries. Table 5 shows the comparison between renewable energy technologies for desalination systems.
Comparison of renewable technologies for desalination in the MENA region [100]
Characteristics | Unit | Wind | Photovoltaics | Concentrated Solar Power |
---|---|---|---|---|
Power dispatch type | – | Unpredictable, fluctuating | Predictable, fluctuating | Flexible, demand-dependent |
Capacity range | MW | >0.05 | >0.005 | >20 |
Plant lifetime | y | 25 | 25 | 35 |
Capacity factor | – | <0.45 | <0.25 | <0.75 |
Total installed capacity | GW | 238 | 67 | 2 |
Investment cost | USD/kWel | 2,000 | 2,600 | 4,500−7,000 |
Storage option | – | Batteries, CAES | Batteries, CAES | Thermal storage |
Storage cost | USD/kWel | 650 | 650 | 100−200 |
Backup option | – | Diesel, conventional power | Diesel, conventional power | Backup boiler |
Backup cost | USD/kWel | 1,500 | 1,500 | 370 |
Table 5 data indicate that solar PV is the best solution for desalination plants. The wind is unpredictable, which eliminates it from the race. Although CSP's backup and storage costs are lower than PV's, its investment is higher, making the water production cost infeasible. One can alter PV backup and storage by providing excess energy into the grid during normal production and taking energy from the grid in case of the unavailability of solar resources. Such a situation eliminates the higher cost of backup and storage, so the cost of produced water is comparable to a conventional desalination system. As the potential of grid cooperation can vary from country to country, hybrid PV-thermal solar can be applied to cover the system's energy requirements. The energy requirement of the desalination system depends upon the type of technology selected. The selection of technology is based on the salinity of seawater, end product quality and available resources [100].
As already mentioned, the MENA region's desalination technology for seawater has been established. Conventional fossil fuel resources fulfil the energy demand for plants. Uncertainty of fossil fuel price and availability makes the future sustainability of existing desalination plants in MENA unclear. Solar energy provides a better solution for a sustainable future in terms of continuous water availability and electricity generation. All these countries have an abundance of seawater and solar irradiance. The only problem with solar-powered systems is their intermittent energy supply which can also be tackled by energy storage for night or cloudy days [97].
There are some driving factors for any technology to be applied in a system. For solar PV applications in existing and future desalination plants, one must consider some critical aspects before determining whether they are sustainable.
The availability of solar irradiance is a precondition for applying a solar PV system for the energy requirement of the desalination plant. Production capacity and energy requirements will be considered for new plants, and solar technology such as PV, CSP, or hybrid PV-CSP system will be installed along with the backup and storage system. One can also use the electricity grid in case of excess and deficit of electricity or install batteries and a thermal storage system. These decisions should be made considering the available and cheapest option in mind. Land availability for the applied technology also plays an important part in the final planning decision.
The economic viability of the considered system is very important to decide on solar technology. Two costs are considered when making the plan. One is the overall cost of the system and its lifetime cost recovery; the second is the cost of water produced. The former is always included in the planning of a project. The latter is important for solar PV desalination systems; it will decide how to make the cost of water production comparable to conventional desalination systems by increasing capacity or changing backup and storage.
Environmental concerns are the most important part of a project as they will decide the legislation and plant output criteria regarding product and waste material. GHG emissions from conventional desalination plants are higher than from solar PV desalination plants. Solar PV plants have no emissions during operation, but manufacturing and commissioning emissions are considered when comparing these to conventional plants. Another environmental factor is brine discharge from the plant, which is the cause of pollution on land or water bodies. A proper technique should be considered for brine disposal.
After deciding about the plant's capacity, technology, economics and environmental aspects, the project's commissioning starts. Several barriers exist, hindering the acceptability and wide applications of solar PV desalination in the MENA region.
Limited financial resources restrict the acceptability of solar desalination. It influences the investors to not switch from conventional systems, although the operational cost is increasing because of the fluctuation of fossil fuel prices and environmental restrictions. Lack of information also limits the investors from entering a new market which will be economically viable and sustainable in the future [97].
Most of the MENA countries have water and power subsidies in conventional systems, which makes the cost of Solar PV electricity and water production higher than the actual cost. These subsidies create unfair competition between conventional and renewable systems and shift the acceptability of renewable systems further away [97].
The old mindset rooted in the administrative structure prefers conventional desalination for the MENA countries and limits the spread of solar PV desalination systems in the region. The centralized administration also discourages independent water production plants. These barriers hinder the acceptability of solar PV systems [97].
The barriers identified above make it hard for the solar PV desalination system acceptance in the region. One can envisage some steps to overcome these barriers.
Proper awareness campaigns from higher authorities will make it helpful for the investors to know about the advantages of renewable over conventional systems. It should also guide the investors about the global market and its shift toward renewable desalination, thus helping them to decide to move into another market.
Subsidies on renewable technologies will make the competition fair other than in the conventional desalination market. It will help make the renewable system economically viable in the MENA countries. It will also decrease the water production cost and uncertainty of revenues. Eventually, investors will start to trust these technologies.
Independent water production should be encouraged to gain investors’ trust, allowing them to select renewable technologies in the desalination system. It will help change the old bureaucratic mindset that prefers the conventional desalination system. The spread of independent production can shift towards renewable desalination technologies.
Solar PV desalination systems are reliable and can potentially be the future of desalination plants in the MENA region. Technical and economic advancements in renewable energy will make the technology applicable from small- to large-scale capacity. GHG emissions from desalination plants are also a big concern that solar PV systems will reduce. Uncertainty in fossil fuel costs will make conventional systems unreliable, but solar desalination systems will provide revenue certainty [101].
There are two major impacts of desalination plants on the environment: marine and soil pollution by brine rejection and air pollution by GHG emissions from the plants’ energy systems. The brine contains residues of flocculants (cationic polymers), coagulants (FeCl3, Fe2(SO4)3), and antiscalants (polyphosphates, phosphonates, and polycarbonic acid). Brine may also carry the residuals and impurities from equipment washing and pre- and post-treatment operations. All these components are detrimental to marine life and degrade the environment. It is estimated that the world desalination plants produce 128,652,000 m3/day of brine in return average of 40% freshwater recovery. Different technologies, feed water quality, product quality and pre- and post-treatment of feedwater define the quantity and quality of brine discharge. Membrane-based technologies have a brine salinity of 60–85 g/L TDS, while thermal technologies have a brine salinity of 55–65 g/L TDS. The high salinity of the former technology contributes to its higher recovery rate of clean water [102].
Brine discharges pollute the water bodies by producing stratification in water due to the salinity of discharged liquid. This phenomenon reduces the dissolved oxygen content in the receiving water body. The high salinity of the water is also detrimental to seawater organisms. It gradually changes the photosynthesis and metabolic growth rates of the flora in water bodies. High turbidity and increased suspended solids reduce light penetration in the water, disturbing the natural processes. The chemicals in the brine pollute the water and form toxic compounds for aquatic life. High-temperature discharge of brine also disturbs the temperature of water bodies, hence disturbing aquatic life [103].
Regardless of membrane technology or thermal desalination, both types of desalination plants are highly energy-intensive. The energy usually comes from fossil fuels in the form of electricity or heat. These plants produce COx, NOx, and SOx, along with particulate matter from fossil fuel combustion, a source of air pollution and global warming. Seawater RO has a global warming potential of 1.77 kg CO2 eq/m3 [103]. The emissions from desalination plants can be reduced using renewable energy.
Brine disposal methods for a desalination system depend upon composition, quantity and quality of brine, available site, geographical location, and the methods’ permissibility. The site's capital and operational costs and future extension capacity are also important. Surface discharge of brine is the most adopted method in desalination plants globally. It consists in discharging the brine into the river, sea or any water body directly but accounting for the fact that the composition of the brine should match the composition of receiving body. Brine pre-treatment to eliminate toxic pollutants is suggested to avoid the contamination of receiving body.
Sewerage discharge of brine solution is also an acceptable method for small-scale desalination plants. This method increases the TDS of the wastewater, which may hinder the performance of the wastewater treatment plant. In that case, brine pre-treatment is required to neutralize pH and eliminate toxic compounds and heavy metals. Deep well injection of the brine to the depth of 500 m to 1500 m is another widely accepted technique. This method creates a risk of contaminating the freshwater aquifers nearby. An extensive study of the plant location is required to eliminate that hazard. Pond evaporation of brine in lined ponds to avoid groundwater contamination may be accepted in the dry areas of desalination plants. The land application of brine is also a valid disposal method. Still, it has many restrictions, as brine spraying on salt-tolerant plants can disturb the salinity of ground aquifers. It also has some restrictions regarding the salinity of irrigation land [104].
A solar desalination system provides a better alternative for reducing carbon emissions. It does not provide any emissions during operations, thus reducing air pollution by eliminating COx, NOx and SOx, which are usually present in fossil fuel processes. Conventional desalination generates 0.3% of the total global load of CO2. Solar technologies provide desalination with no regional emissions and protect the environment [101].
PV solar is an advanced and quite new technology for water desalination systems. The world is going to deal with high water stress in the near future. It will necessitate adopting the water reservoirs’ maintenance to secure a clean freshwater supply. The desalination systems are not applicable worldwide, but some regions abundant in seawater and solar insolation can easily accept and apply solar desalination technology. The MENA region is likely susceptible to future freshwater crises. Population growth, urbanization, industrialization, climate change, and environmental factors are responsible for the very limited freshwater supply remaining in this region. Underground and surface water resources are scarce or depleting because of excessive use or prevailing condition of climate change. Almost all countries in the MENA region are either in shortage of water supply for use or will be in the coming years. Governments of these countries are already planning to produce potable freshwater from alternative resources, especially renewable ones. They consider water desalination plants to produce freshwater from seawater and brackish water on a large scale.
PV solar desalination systems are an attractive option to desalinate seawater and brackish water using renewable resources. However, there are some limitations in the public acceptance of renewable desalination systems. One of the most viable methods is offering government subsidies on PV coupled desalination systems, which are much needed to make these systems economically viable. In some countries, concentrated and non-concentrated solar technologies gave good outcomes. Hybrid renewable systems are also feasible in some cases to install immediately and are ready for production. Desalination systems are also very much feasible for remote areas or those areas where power transmission is not easy.
This article concludes that future water demands depend on sustainable energy desalination systems to ensure water availability. Actual analysis and installation should be the next action and should be taken immediately because the climate changes and renewable water resources are either depleting or not usable. The article has given a roadmap for PV solar desalination, discussing barriers and steps to overcome the challenges in the MENA region. Existing solar desalination plants can expand, and new plants can be installed to provide future water demands.
CAES | Compressed Air Energy Storage |
CAPEX | Capital Expenditures |
CSP | Concentrated Solar Power |
GCC | Gulf Cooperation Council |
GDP | Gross Domestic Product |
GHG | Greenhouse Gas |
MD | Membrane Desalination |
MED | Multi-Effect Distillation |
MEE | Multi-Effect Evaporation |
MENA | Middle East and North Africa |
MSF | Multi-Stage Flash |
MVC | Mechanical Vapour Compression |
OPEX | Operating Expenditures |
PTFE | Polytetrafluoroethylene |
PV | Photovoltaic |
PVDF | Polyvinylidene Difluoride |
RO | Reverse Osmosis |
SDP | Solar Desalination Plant |
SHDD | Solar Humidification-Dehumidification Desalination |
SMD | Solar Membrane Desalination |
SMEDD | Solar Powered Multi-Effect Distillation Desalination |
SMSFD | Solar Multistage Flash Desalination |
SRO | Solar Powered Reverse Osmosis |
TDS | Total Dissolved Salts |
VCD | Vapour Compression Distillation |
- “Drinking-water,” World Health Organization. Water, Sanitation and Health Team, 2004, https://apps.who.int/iris/handle/10665/42852
- https://www.cdc.gov/healthywater/global/wash_statistics.html , 2022,
- https://www.usbr.gov/mp/arwec/water-facts-ww-water-sup.html , 2020,
Water security ,Water Well J. , Vol. 56 (3),pp 58 , 2002, https://doi.org/https://doi.org/10.1201/9780203878057.pt2
, The ABCs of desalting , Vol. 2 , 2000
, Hybrid concentrated solar power (CSP)-desalination systems: A review ,Desalination , Vol. 468 ,pp 114083 , 2019, https://doi.org/https://doi.org/10.1016/J.DESAL.2019.114083
, Solar powered desalination – Technology, energy and future outlook ,Desalination , Vol. 453 ,pp 54–76 , 2019, https://doi.org/https://doi.org/10.1016/J.DESAL.2018.12.002
, Desalination and water reuse to address global water scarcity ,Rev. Environ. Sci. Biotechnol. , Vol. 16 (4),pp 591–609 , 2017, https://doi.org/https://doi.org/10.1007/s11157-017-9449-7
, The global status of desalination: An assessment of current desalination technologies, plants and capacity ,Desalination , Vol. 495 (July),pp 114633 , 2020, https://doi.org/https://doi.org/10.1016/j.desal.2020.114633
, The future of seawater desalination: Energy, technology, and the environment ,Science (80-.). , Vol. 333 (6043),pp 712–717 , 2011, https://doi.org/https://doi.org/10.1126/SCIENCE.1200488/SUPPL_FILE/ELIMELECH.SOM.PDF
, A feasibility study of solar-powered reverse osmosis processes ,Desalination , Vol. 500 ,pp 114885 , 2021, https://doi.org/https://doi.org/10.1016/J.DESAL.2020.114885
, - , Renewable Energy, a Clean Environment and Solar Desalination, Water management in MENA region, 2020
- , Concentrating Solar Power and Desalination Plants, Solar resource mapping, 2019
Solar assisted sea water desalination: A review ,Renew. Sustain. Energy Rev. , Vol. 19 ,pp 136–163 , 2013, https://doi.org/https://doi.org/10.1016/J.RSER.2012.04.059
, Environmental sustainability: Role of green technologies ,Environ. Sustain. Role Green Technol. ,pp 1–324 , 2015, https://doi.org/https://doi.org/10.1007/978-81-322-2056-5/COVER
, Recent Developments in Solar Thermal Desalination Technologies: A Review ,Energies , Vol. 1212 (1),pp 119 , 2019, https://doi.org/https://doi.org/10.3390/EN12010119
, Solar energy for water desalination ,Procedia Eng. , Vol. 46 (0),pp 220–227 , 2012, https://doi.org/https://doi.org/10.1016/j.proeng.2012.09.468
, Effect of ZnO nanostructures on the performance of dye sensitized solar cells ,Sol. Energy , Vol. 230 ,pp 492–500 , 2021, https://doi.org/https://doi.org/10.1016/J.SOLENER.2021.10.045
, A review on the coupling of cooling, desalination and solar photovoltaic systems ,Renew. Sustain. Energy Rev. , Vol. 47 ,pp 703–717 , 2015, https://doi.org/https://doi.org/10.1016/j.rser.2015.03.083
, Use of low grade in driving small freezing units for desalination ,Desalination , Vol. 61 (2),pp 169–183 , 1987, https://doi.org/https://doi.org/10.1016/0011-9164(87)80016-4
, A discussion of ‘Heat pumps as a source of heat energy for desalination of seawater,’ ,Desalination , Vol. 169 (2),pp 161–165 , 2004, https://doi.org/https://doi.org/10.1016/S0011-9164(04)00523-5
, Membrane distillation: A comprehensive review ,Desalination , Vol. 287 ,pp 2–18 , 2012, https://doi.org/https://doi.org/10.1016/j.desal.2011.08.027
, Cost analysis of different solar still configurations ,Energy , Vol. 35 (7),pp 2901–2908 , 2010, https://doi.org/https://doi.org/10.1016/j.energy.2010.03.021
, Economics of desalination for three plant sizes ,Desalination , Vol. 78 (2),pp 187–200 , 1990, https://doi.org/https://doi.org/10.1016/0011-9164(90)80042-A
, Overview of solar desalination ,Desalination , Vol. 93 (1–3),pp 563–579 , 1993, https://doi.org/https://doi.org/10.1016/0011-9164(93)80131-6
, An energy-efficient submarine desalination plant ,Desalination , Vol. 122 (2–3),pp 171–176 , 1999, https://doi.org/https://doi.org/10.1016/S0011-9164(99)00038-7
, Renewable energy applications in desalination: State of the art ,Sol. Energy , Vol. 75 (5),pp 381–393 , 2003, https://doi.org/https://doi.org/10.1016/j.solener.2003.08.005
, Thermoeconomic analysis of a solar parabolic trough collector distillation plant ,Desalination , Vol. 122 (2–3),pp 215–224 , 1999, https://doi.org/https://doi.org/10.1016/S0011-9164(99)00051-X
, Exploitation of solar energy collected by solar stills for desalination by membrane distillation ,Renew. Energy , Vol. 25 (2),pp 293–305 , 2002, https://doi.org/https://doi.org/10.1016/S0960-1481(01)00058-1
, Desalination by a ‘compact SMADES’ autonomous solarpowered membrane distillation unit ,Desalination , Vol. 217 (1–3),pp 29–37 , 2007, https://doi.org/https://doi.org/10.1016/j.desal.2006.11.028
, Experimental evaluation of hybrid solar still using waste heat ,Desalination , Vol. 379 ,pp 1–9 , 2016, https://doi.org/https://doi.org/10.1016/J.DESAL.2015.10.004
, Different parameters affecting the rate of evaporation and condensation on passive solar still – A review ,Renew. Sustain. Energy Rev. , Vol. 38 ,pp 309–322 , 2014, https://doi.org/https://doi.org/10.1016/J.RSER.2014.05.092
, Solar energy technologies: principles and applications ,Renewable-Energy-Driven Futur. ,pp 3–42 , 2021, https://doi.org/https://doi.org/10.1016/B978-0-12-820539-6.00001-7
, PV and thermally driven small-scale, stand-alone solar desalination systems with very low maintenance needs ,Desalination , Vol. 225 (1–3),pp 58–69 , 2008, https://doi.org/https://doi.org/10.1016/J.DESAL.2006.11.029
, Recent fresh water augmentation techniques in solar still and HDH desalination – A review ,Renew. Sustain. Energy Rev. , Vol. 82 ,pp 629–644 , 2018, https://doi.org/https://doi.org/10.1016/J.RSER.2017.09.056
, Experimental study of the enhancement parameters on a single slope solar still productivity ,Desalination , Vol. 209 (1–3),pp 136–143 , 2007, https://doi.org/https://doi.org/10.1016/J.DESAL.2007.04.022
, Developments in Solar Still Desalination Systems: A Critical Review ,Crit. Rev. Environ. Sci. Technol. , Vol. 42 (19),pp 2078–2112 , 2012, https://doi.org/https://doi.org/10.1080/10643389.2011.574104
, Enhancement of modified solar still integrated with external condenser using nanofluids: An experimental approach ,Energy Convers. Manag. , Vol. 78 ,pp 493–498 , 2014, https://doi.org/https://doi.org/10.1016/J.ENCONMAN.2013.11.013
, Factors influencing the performance and productivity of solar stills - A review ,Desalination , Vol. 435 ,pp 181–187 , 2018, https://doi.org/https://doi.org/10.1016/J.DESAL.2017.09.031
, Software to analyze solar stills and an experimental study on the effects of the cover ,Desalination , Vol. 114 (1),pp 37–44 , 1997, https://doi.org/https://doi.org/10.1016/S0011-9164(97)00152-5
, Efficiency improvement of solar stills through wettability alteration of the condensation surface: An experimental study ,Appl. Energy , Vol. 268 ,pp 114923 , 2020, https://doi.org/https://doi.org/10.1016/J.APENERGY.2020.114923
, Parameters influencing the productivity of solar stills – A review ,Renew. Sustain. Energy Rev. , Vol. 49 ,pp 585–609 , 2015, https://doi.org/https://doi.org/10.1016/J.RSER.2015.04.136
, A review of efficient high productivity solar stills ,Renew. Sustain. Energy Rev. , Vol. 101 ,pp 197–220 , 2019, https://doi.org/https://doi.org/10.1016/J.RSER.2018.11.013
, Experimental and water quality analysis of solar stills with vertical and inclined fins ,Groundw. Sustain. Dev. , Vol. 11 ,pp 100410 , 2020, https://doi.org/https://doi.org/10.1016/J.GSD.2020.100410
, A review of techniques for simultaneous enhancement of evaporation and condensation rates in solar stills ,Sol. Energy , Vol. 225 ,pp 666–693 , 2021, https://doi.org/https://doi.org/10.1016/J.SOLENER.2021.07.028
, The performance of a modified solar still using hot air injection and PCM ,Desalination , Vol. 379 ,pp 102–107 , 2016, https://doi.org/https://doi.org/10.1016/J.DESAL.2015.11.007
, Water desalination using humidification/dehumidification (HDH) technique powered by solar energy: a detailed review ,Desalin. Water Treat. , Vol. 52 (25–27),pp 4622–4640 , 2014, https://doi.org/https://doi.org/10.1080/19443994.2013.804457
, Water Desalination Using a Humidification-Dehumidification Technique—A Detailed Review ,Nat. Resour. , Vol. 4 (3),pp 286–305 , 2013, https://doi.org/https://doi.org/10.4236/NR.2013.43036
, - , Humidification-Dehumidification Desalination, Desalination: Water from Water, 2019
A study on the maximum gained output ratio of single-effect solar humidification-dehumidification desalination ,Sol. Energy , Vol. 157 ,pp 1–9 , 2017, https://doi.org/https://doi.org/10.1016/J.SOLENER.2017.08.014
, Solar humidification-dehumidification desalination systems: A critical review ,Energy Convers. Manag. , Vol. 201 ,pp 112129 , 2019, https://doi.org/https://doi.org/10.1016/J.ENCONMAN.2019.112129
, Experimental investigation of a new solar desalination prototype using the humidification dehumidification principle ,Renew. Energy , Vol. 35 (11),pp 2610–2617 , 2010, https://doi.org/https://doi.org/10.1016/J.RENENE.2010.03.033
, Solar Desalination ,Desalination and Water Treatment, IntechOpen ,pp 127–150 , 2018, https://doi.org/https://doi.org/10.5772/INTECHOPEN.76981
, Solar desalination with a humidification-dehumidification technique — a comprehensive technical review ,Desalination , Vol. 160 (2),pp 167–186 , 2004, https://doi.org/https://doi.org/10.1016/S0011-9164(04)90007-0
, Performance and cost assessment of solar driven humidification dehumidification desalination system ,Energy Convers. Manag. , Vol. 132 ,pp 28–39 , 2017, https://doi.org/https://doi.org/10.1016/J.ENCONMAN.2016.10.005
, Potential of a dual purpose solar collector on humidification dehumidification desalination system ,Desalination , Vol. 404 ,pp 35–40 , 2017, https://doi.org/https://doi.org/10.1016/J.DESAL.2016.10.015
, A review of solar energy driven desalination technologies ,Renew. Sustain. Energy Rev. , Vol. 41 ,pp 1080–1118 , 2015, https://doi.org/https://doi.org/10.1016/J.RSER.2014.09.002
, Multistage flash desalination: present and future outlook ,Chem. Eng. J. , Vol. 73 (2),pp 173–190 , 1999, https://doi.org/https://doi.org/10.1016/S1385-8947(99)00035-2
, Performance ratio, area economy and economic return for an integrated solar energy/multistage flash desalination plant ,Desalination , Vol. 73 (C),pp 191–195 , 1989, https://doi.org/https://doi.org/10.1016/0011-9164(89)87013-4
, Techno-economic assessment and environmental impacts of desalination technologies ,Desalination , Vol. 266 (1–3),pp 263–273 , 2011, https://doi.org/https://doi.org/10.1016/j.desal.2010.08.035
, A novel design for a solar powered multistage flash desalination ,Sol. Energy , Vol. 153 ,pp 348–359 , 2017, https://doi.org/https://doi.org/10.1016/J.SOLENER.2017.05.082
, Performance evaluation of a brine-recirculation multistage flash desalination system coupled with nanofluid-based direct absorption solar collector ,Renew. Energy , Vol. 122 ,pp 140–151 , 2018, https://doi.org/https://doi.org/10.1016/J.RENENE.2018.01.050
, Experimental characterization of a solar powered MSF desalination process performance ,Therm. Sci. Eng. Prog. , Vol. 10 ,pp 154–162 , 2019, https://doi.org/https://doi.org/10.1016/J.TSEP.2019.01.018
, Technoeconomic analysis of solar thermal desalination ,Desalination , Vol. 474 ,pp 114168 , 2020, https://doi.org/https://doi.org/10.1016/J.DESAL.2019.114168
, New opportunities in mass and energy consumption of the Multistage Flash Distillation type of brackish water desalination process ,Sol. Energy , Vol. 153 ,pp 115–125 , 2017, https://doi.org/https://doi.org/10.1016/J.SOLENER.2017.05.021
, Proof-of-concept study of an integrated solar desalination system ,Renew. Energy , Vol. 34 (12),pp 2798–2802 , 2009, https://doi.org/https://doi.org/10.1016/J.RENENE.2009.06.002
, Performance evaluation of multi-effect distiller for optimized solar thermal desalination ,Appl. Therm. Eng. , Vol. 61 (2),pp 491–499 , 2013, https://doi.org/https://doi.org/10.1016/J.APPLTHERMALENG.2013.08.006
, Energy efficient multi-effect distillation powered by a solar linear Fresnel collector ,Energy Convers. Manag. , Vol. 171 ,pp 576–586 , 2018, https://doi.org/https://doi.org/10.1016/J.ENCONMAN.2018.05.082
, Multi-effect distillation plants for small-scale seawater desalination: thermodynamic and economic improvement ,Energy Convers. Manag. , Vol. 205 ,pp 112337 , 2020, https://doi.org/https://doi.org/10.1016/J.ENCONMAN.2019.112337
, Thermodynamic and economic analysis of the integration of Organic Rankine Cycle and Multi-Effect Distillation in waste-heat recovery applications ,Energy , Vol. 161 ,pp 456–469 , 2018, https://doi.org/https://doi.org/10.1016/J.ENERGY.2018.07.150
, A novel design for solar integrated multi-effect distillation driven by sensible heat and alternate storage tanks ,Desalination , Vol. 468 ,pp 114061 , 2019, https://doi.org/https://doi.org/10.1016/J.DESAL.2019.07.001
, Water desalination system using solar heat: A review ,Renew. Sustain. Energy Rev. , Vol. 67 ,pp 1308–1330 , 2017, https://doi.org/https://doi.org/10.1016/J.RSER.2016.08.058
, An optimization model for a mechanical vapor compression desalination plant driven by a wind/PV hybrid system ,Appl. Energy , Vol. 88 (11),pp 4042–4054 , 2011, https://doi.org/https://doi.org/10.1016/J.APENERGY.2011.04.031
, Energy/exergy analysis of solar driven mechanical vapor compression desalination system with nano-filtration pretreatment ,Desalination , Vol. 509 ,pp 115078 , 2021, https://doi.org/https://doi.org/10.1016/J.DESAL.2021.115078
, Performance analysis of the mechanical vapor compression desalination system driven by an organic Rankine cycle ,Energy , Vol. 141 ,pp 1177–1186 , 2017, https://doi.org/https://doi.org/10.1016/J.ENERGY.2017.10.014
, Photothermal nanocomposite membranes for direct solar membrane distillation ,J. Mater. Chem. A , Vol. 5 (45),pp 23712–23719 , 2017, https://doi.org/https://doi.org/10.1039/C7TA04555G
, Scaling Resistance in Nanophotonics-Enabled Solar Membrane Distillation ,Environ. Sci. Technol. , Vol. 54 (4),pp 2548–2555 , 2020, https://doi.org/https://doi.org/10.1021/ACS.EST.9B07622/SUPPL_FILE/ES9B07622_SI_001.PDF
, Reverse osmosis desalination: A state-of-the-art review ,Desalination , Vol. 459 ,pp 59–104 , 2019, https://doi.org/https://doi.org/10.1016/J.DESAL.2019.02.008
, A comprehensive techno-economical review of indirect solar desalination ,Renew. Sustain. Energy Rev. , Vol. 15 (8),pp 4187–4199 , 2011, https://doi.org/https://doi.org/10.1016/J.RSER.2011.05.012
, Autonomous reverse osmosis units driven by RE sources experiences and lessons learned ,Desalination , Vol. 221 (1–3),pp 29–36 , 2008, https://doi.org/https://doi.org/10.1016/J.DESAL.2007.02.048
, World’s first solar powered reverse osmosis desalination plant ,Desalination , Vol. 41 (2),pp 233–237 , 1982, https://doi.org/https://doi.org/10.1016/S0011-9164(00)88726-3
, Laboratory setup for water purification using household PV-driven reverse osmosis unit ,New pub Balaban , Vol. 7 (1–3),pp 53–59 , 2012, https://doi.org/https://doi.org/10.5004/DWT.2009.695
, Canary Islands Institute of Technology (ITC) experiences in desalination with renewable energies (1996–2008) ,New pub Balaban , Vol. 7 (1–3),pp 220–235 , 2012, https://doi.org/https://doi.org/10.5004/DWT.2009.733
, Reverse osmosis (RO) membrane desalination driven by wind and solar photovoltaic (PV) energy: State of the art and challenges for large-scale implementation ,Renew. Sustain. Energy Rev. , Vol. 112 ,pp 669–685 , 2019, https://doi.org/https://doi.org/10.1016/J.RSER.2019.06.008
, The application of water–energy nexus in the Middle East and North Africa (MENA) region: a structured review ,Appl. Water Sci. , Vol. 12 (5),pp 1–21 , 2022, https://doi.org/https://doi.org/10.1007/S13201-022-01613-7/TABLES/2
, Seawater Desalination Markets in MENA ,Desalination ,pp 97–118 , 2007
, - By the numbers: Facts about water crisis in the Arab World, 2015, https://blogs.worldbank.org/arabvoices/numbers-facts-about-water-crisis-arab-world
Wastewater production, treatment, and irrigation in Middle East and North Africa ,Irrig. Drain. Syst. , Vol. 24 (1–2),pp 37–51 , 2010, https://doi.org/https://doi.org/10.1007/s10795-009-9081-y
, - Water resources and climate change in MENA region, 2021, http://www.cadtm.org/Water-resources-and-climate-change,5080
- , , Water reuse in Middle Eastern and North African countries, 2008
Wastewater production, treatment, and irrigation in Middle East and North Africa ,Irrig. Drain. Syst. , Vol. 241 ,pp 24 , 20092009, https://doi.org/https://doi.org/10.1007/S10795-009-9081-Y
, Overview of the integration of CSP as an alternative energy source in the MENA region ,Energy Strateg. Rev. , Vol. 29 ,pp 100493 , 2020, https://doi.org/https://doi.org/10.1016/J.ESR.2020.100493
, - Renewable energy desalination: an emerging solution to close the water gap in MENA, 2013, http://hdl.handle.net/10986/11963
A flexible techno-economic model for the assessment of desalination plants driven by renewable energies ,New pub Balaban , Vol. 55 (11),pp 3091–3105 , 2014, https://doi.org/https://doi.org/10.1080/19443994.2014.946718
, The state of desalination and brine production: A global outlook ,Sci. Total Environ. , Vol. 657 (March),pp 1343–1356 , 2019, https://doi.org/https://doi.org/10.1016/j.scitotenv.2018.12.076
, Different configurations of humidification-dehumidification desalination systems: Thermal and economic assessment ,Energy Convers. Manag. , Vol. 258 ,pp 115470 , 2022, https://doi.org/https://doi.org/10.1016/J.ENCONMAN.2022.115470
, - SolarDesalMENA: Overview of Solar Seawater Desalination in the MENA Region, 2015, https://www.giz.de/en/downloads/giz2015_en_SolarDesalMENA_Overview_of_Solar_Seawater_Desalination_in_the_MENA_Region.pdf
Renewable Energy in Desalination and Hybrid Technologies ,Gas Hydrate Water Treat. ,pp 237–267 , 2022, https://doi.org/https://doi.org/10.1002/9781119866145.CH10
, - , 2013, www.worldbank.org/mna/watergap
Renewable desalination: A methodology for cost comparison ,Desalin. Water Treat. , Vol. 51 (4–6),pp 1171–1189 , 2013, https://doi.org/https://doi.org/10.1080/19443994.2012.715446
, Technology development and application of solar energy in desalination: MEDRC contribution ,Renew. Sustain. Energy Rev. , Vol. 15 (9),pp 4410–4415 , 2011, https://doi.org/https://doi.org/10.1016/j.rser.2011.06.017
, Environmental impacts of desalination and brine treatment - Challenges and mitigation measures ,Mar. Pollut. Bull. , Vol. 161 (October), 2020, https://doi.org/https://doi.org/10.1016/j.marpolbul.2020.111773
, Environmental impact of desalination technologies: A review ,Sci. Total Environ. , Vol. 748 ,pp 141528 , 2020, https://doi.org/https://doi.org/10.1016/j.scitotenv.2020.141528
, Desalination brine disposal methods and treatment technologies - A review ,Sci. Total Environ. , Vol. 693 , 2019, https://doi.org/https://doi.org/10.1016/j.scitotenv.2019.07.351
,