The study and adoption of renewable energy are growing continuously as a result of rising natural resource prices and environmental concerns. Although renewable energy sources are not entirely free from pollution, they are receiving significant attention due to their environmentally friendly nature. As of 2023, most countries have committed to investing in renewable energies and have implemented specific targets for their utilization, as reported by the Renewable Energy Policy Network [1].
From an environmental perspective, fossil fuels are a finite natural resource that will eventually be exhausted. Global oil reserves are projected to be depleted by 2038 based on current consumption trends [2]. The blessing of the Arabian Peninsula starts from its central location to the natural resources it contains such as oil and gas, and throughout history, the sun in this wide and diverse peninsula is free and never ending in its giving, which unfortunately has been neglected as part of the energy mix of the nations within the peninsula. This source of energy covers many aspects of the prospects of a sustainable nation by providing energy security where there is no need for raw fossil fuel energy, which is not eco-friendly, to be imported to nations with solar extraction capability as an available clean option in the Arab world. Considering the hot climate, especially in the Gulf region, investing in solar energy will aid in meeting the electricity demands in the future, as populations are at constant growth, where it can be used in different applications.
In Kuwait, fossil fuels are heavily relied upon for heating, electricity, transportation, and manufacturing. Unfortunately, these activities contribute significantly to air pollution due to the combustion of fossil fuels. The hot and humid climate in Kuwait exacerbates the issue by easily allowing carbon dioxide, a byproduct of fuel combustion, to pollute the air. Due to the hot climate, it is a human necessity to teleport to places using vehicles, yet available cars use fuel that has been proven over the past centuries to have tremendous effects on global warming. Therefore, the adoption of solar-powered vehicles would help reduce air pollution and preserve fossil fuels for future generations by diversifying the available energy sources.
Findings from the study in [3] indicated that, a sustainable global electricity transition will entail increased use of renewable energy sources, particularly wind, solar, and nuclear energy, as low-carbon energy sources. In regions with high gasoline prices, electric cars are gaining popularity. However, electric vehicles continue to grapple with power-related obstacles because they cannot achieve desired distances without requiring recharging. Despite the increasing popularity of hub motors, which are known for their efficiency and ease of installation, electric vehicles still face power-related challenges. Solar cars offer a solution by mitigating the recharging problem and providing a sustainable source of green energy.
In just one hour, the solar energy received by the Earth can meet the energy requirements for an entire year. The United States government has set a national objective of deploying one million electric vehicles (EVs) by 2015. In Canada, specifically in Ontario, the Ministry of Transportation is allocating $20 million from its Green Investment Fund to construct 500 electric vehicle charging stations (EVCSs) [4]. The German National Electric Mobility Platform (NPE) envisions approximately one million EVs and a demand for around 70,000 on-street charging spots by 2020 [5]. To accelerate the global growth of EVs, numerous countries have collaborated to establish the Electric Vehicles Initiative (EVI).
The study conducted in [6] reviewed the design and implementation of a hybrid vehicle in terms of system efficiency and dependency reduction on fossil fuels. This study explains different types of partly and fully electric power vehicles. Nicolae et al. [7], presented a detailed design of the Hybrid Electric Vehicle (HEV) that includes an induction motor and a three-phase Voltage Source Inverter (VSI) with battery bank design steps. This study also explains the concerning aspects of involving VSI in the system.
Researchers in [8] developed an enhanced method for estimating the charging state of an HEV through efficient energy management. Their approach employed a global strategy of Fuzzy Logic Control and extensively discussed various HEV topologies and operating modes. In [9], the authors have presented an HEV model based on the Permanent Magnet-Dual Mechanical Ports machine, known as Electrical Variable Transmission, which considers the specifications of the Prius HEV. They concluded that the proposed HEV system using Permanent Magnet Electric Variable Transmission (PM-EVT) machines could effectively replace the Toyota Hybrid System (THS) transmission, particularly in the Prius Series-Parallel Hybrid Electric Vehicle (SPHEV). A power management system for HEV applications aiming to achieve energy savings by utilizing supercapacitors and the use of a switching-mode power supply with a step-up transformer in a single-phase system was proposed in [10].
Several studies have concentrated on enhancing the performance of solar-powered HEVs by considering various factors. In [11], the authors examined different renewable energy sources and subsequently introduced a hybrid thermoelectric– photovoltaic energy system specifically designed for HEV applications. The system was thoroughly investigated and demonstrated successful outcomes.
Different design specifications and requirements for manufacturing and successfully testing a small solar car have been presented in [12]. Furthermore, the design of a compact solar-powered HEV has been discussed in [9]. This study involved the design and implementation of the HEV system, which included components such as the battery, PV panel, converter, and DC motor.
The Journal of Sustainable Development of Energy, Water and Environment Systems (JSDEWES) is a prominent publication platform for improving and sharing knowledge about methods, policies and technologies that promote sustainability [13]. Several publications within the JSDEWES library delve into various aspects of solar technologies.
The study carried out in [14] reviewed the environmental impacts associated with solar and wind energy. The authors investigated the key factors that affect these renewable sources, including land use, water consumption, impact on biodiversity, visual and noise effects, health issues, and impact on microclimate. Their paper highlights the importance of carrying out comprehensive environmental impact assessments before the installation of solar and wind energy sources. They indicated that with enhancements in planning, understanding and optimization of constructing renewable energy plants, it is possible to reduce or avoid many of the predicted adverse effects.
In their exploration [15], the researchers focused on the impact of shadow and natural dust on the performance of PV modules. According to their study, shading and light obstruction on cells causes a considerable impact on the PV modules performance. The researchers suggested several solutions for maintaining higher efficiencies, including regular cleaning, considering titled modules, and monitoring dust accumulation.
The authors in [16], underscore the environmental advantages of solar-based electric vehicle charging over conventional grid-based methods and emphasize the vital need for a simplified, universally applicable charging management system. They presented a comparison between Raspberry Pi and ESP32 components to develop a reliable charging setup. As a result, the ESP32 integrated with Blynk, appears as a more economical and simpler alternative for managing energy charging.
The authors in [17] had improved the performance of PV panels using a direct water-cooling system. They determined that the efficiency of the panels increases by 12% under laboratory conditions and by 1.2 – 13% under real conditions compared to an uncooled panel. Researchers in [18] addressed the lack of electricity in rural areas by utilizing Particle Swarm Optimization algorithm to enhance the efficiency and planning of the microgrids. The observed outcomes demonstrated that an optimal management ensures a Loss of Power Supply Probability of 0.18%, a Levelized Cost of Electricity amounts to US$ 0.0187, and a Maximum Renewable Factor reaches 98%.
In [19], the authors focus on resolving the power quality issues caused by PV systems when integrated into the grid. The authors identifies that the power electronic converters used in the PV systems are the source of power quality issues, mainly due to harmonic injection and unbalanced currents. The authors suggest utilizing modern active filters to mitigate harmonic distortion equipped with controllers and voltage source converters to adequately reduce the impact of unbalanced currents and harmonic injection.
Another domain where solar technologies are utilized is in the realm of desalination. The researches in [20] cited that the climatic conditions of the Middle East and North Africa region countries possesses higher solar flux which makes these arears conducive to harnessing solar energy. A road map for the future solar desalination plants were provided, challenges in the technique’s applicability were addressed and strategies to overcome these challenges were proposed.
Additional benefits of employing solar technologies are showcased in the case study of [21]. A model of solar PV system connected to the grid was proposed in the Brazilian semi-grid area which has high solar radiation of 6.03 kWh/m^2. It has been discovered that the system was capable of delivering 3,500 kWh/month to 5,500 kWh/month, estimated by HOMER computational tool, and 4,257.01 kWh/month to 5,504.01 kWh/month estimated by SAM tool, given that the electrical load was 2,350 kWh/month. Additionally, this system generated income for local farmers by enabling the surplus energy to be sold, resulting in a yearly income ranging from US 2,243.63 for HOMER outcomes, US 2,681.50 for SAM outcomes.
Authors in [22] used India as a case study to study the application of using a residential rooftop photovoltaic using the Bass model which is used to predict the diffusion patterns of the product, in this case the photovoltaic. This product is essentially used as an electric source in small communities and will comply with India’s target for renewable energy and its intended nationally determined contribution goals. The finding of this research was that the photovoltaic diffusion in India’s market will be growing at a slow rate in comparison with other photovoltaic markets.
Another case study done on India was about the prediction of global solar radiation using artificial neural network [23]. Places in India with high solar radiation maximizes the returns on solar farms, however the exact values aren’t available for everyone. Hence, this paper introduces a function, obtained by Artificial Neural Networks (ANN), that takes an input parameter of seven geographical and meteorological parameters affecting the radiation, and outputs as a result Global Solar Radiation.
The journal in [24], addressed the main issues with solar cells research papers as they are rapidly increasing, however research trends and research issues are hard to recognize due to the many subdivided topics related to the same field of study. To solve this, authors apply machine learning techniques in which they were able to extract emerging research papers, frequently cited articles, and old research papers that might arise in the future.
Norway has deployed the Kyoto Protocol, energy transition, as a result of the global climate change and to ensure the security of their renewable energy sources as mentioned in [25]. In which energy security consists of availability, accessibility, affordability and acceptability, where the paper studied the relationship between energy efficiency and the deployment of renewable energy related to energy transition. Also, it identifies the accurate indicators for the components of the Renewable Energy Solutions, Inc.
With the rise of sustainable energy technologies, it is necessary to prioritize the global warming gases emissions that are caused by home appliances such as refrigerators. In this study [26], autonomous solar refrigeration was evaluated with the small capacity DC refrigerator with internal heat exchange systems using R600a, R290, R717, and R313a, at certain evaporation and condensation temperatures. As the paper suggested ideal values for the different internal heat exchange systems used, to ensure high effectiveness.
A study done on a solar panel [27] introduced a technique to control low speed orientation of the panel. The technique used variable mass system and a torsional spring, and their effectiveness were evaluated on the solar panel. The first parameter, the variable mass system, produced a torque the controls the platform orientation which was tested by using an electric pump to move the water from different sides of the panel. Thus, the energy consumption of using an electric pump for controlling the orientation of the solar panel was significantly less than using an actuator.
As many countries try to utilize different renewable energy resources to meet future demands and to ensure a secure energy, individual and households should be willing to pay as suggested by a case study in Nigeria [28]. The study uses Nigeria’s history with electricity outages and urges the urban households to be part in adopting off-grid solar photovoltaic systems. Although the percentage of households that are willing to pay is high, yet it provides suggestions that will yield more participation.
Authors in [29], viewed the rapidly increasing market of electric vehicles and the shift towards the usage of different renewable electric sources. It also studies the different factors that caused a variation in the market in different locations due to the social and technical capability of the community. With the increase of electric demand and population, it is necessary to highlight the need for energy solutions and the energy efficiency strategies. Hence, the paper persuades in electric vehicle adoption that can efficiently meet the energy demand required.
Another study done on combining distiller technology with a solar energy introduced a design for the Hybrid single slope Solar distiller with PV powered Thermoelectric [30]. The output is sustainable and environmentally friendly in which it will provide water without emissions. The significance of the design lies in the high efficiency of the product at which it utilizes the vapor produced by the heat and sends it to the water, which reduces losses in comparison with the traditional distiller technique. Also, they provided a mathematical function too accurately describe its behaviour and functionality.
A case study in Morocco [31] emphasizes aerosols as the primary factor causing attenuation in solar radiation, under clear sky conditions. The findings indicated a drop in global horizontal irradiance compared to the global irradiance at the top of the atmosphere of 24% for aerosol optical depth values of 0.02 and surpassing 53% for 0.6. The direct normal irradiance drops in relation to extraterrestrial irradiance as aerosol optical depth rises. At an optical depth of 0.2, there has been a reduction of 66%, increasing to 92% at an optical depth of 0.6.
This section briefly discusses different implementations of solar cars. Each car had different features along with advantages and disadvantages.
The Surya solar car shown in Figure 1a was implemented to participate in the Atacama solar race conducted in Latin America. The solar car has six flexible solar panels with an efficiency of 22.5%, a nominal power of 100 W, three 48 V lithium batteries, and 1 kW magnet motor [32].
Another racing solar car was designed by students from a South African university to participate in the South Africa 2012 Solar Challenge. Due to the strict conditions of the race regarding the component’s ratings, the team was obliged to use lithium-ion cells battery that weighted 30 kg, two 165 V Marand motors, two Tritium Wave Sculptor controller with a 98% efficiency, and four wheels. The team had to regroup the battery blocks into boxes, where the number of cells used was 5300 cells. The main drawbacks of the design shown in Figure 1b were the fragile photovoltaic cells and the unreliable connections of the motor with the control system [33].
The previous solar car attempts: First Duoc-UC’s Solar Energy Powered Car [32] (a); Ilangal Solar Car [33] (b); Qatar University First Solar Car [34] (c); Solar Car018 [35] (d)
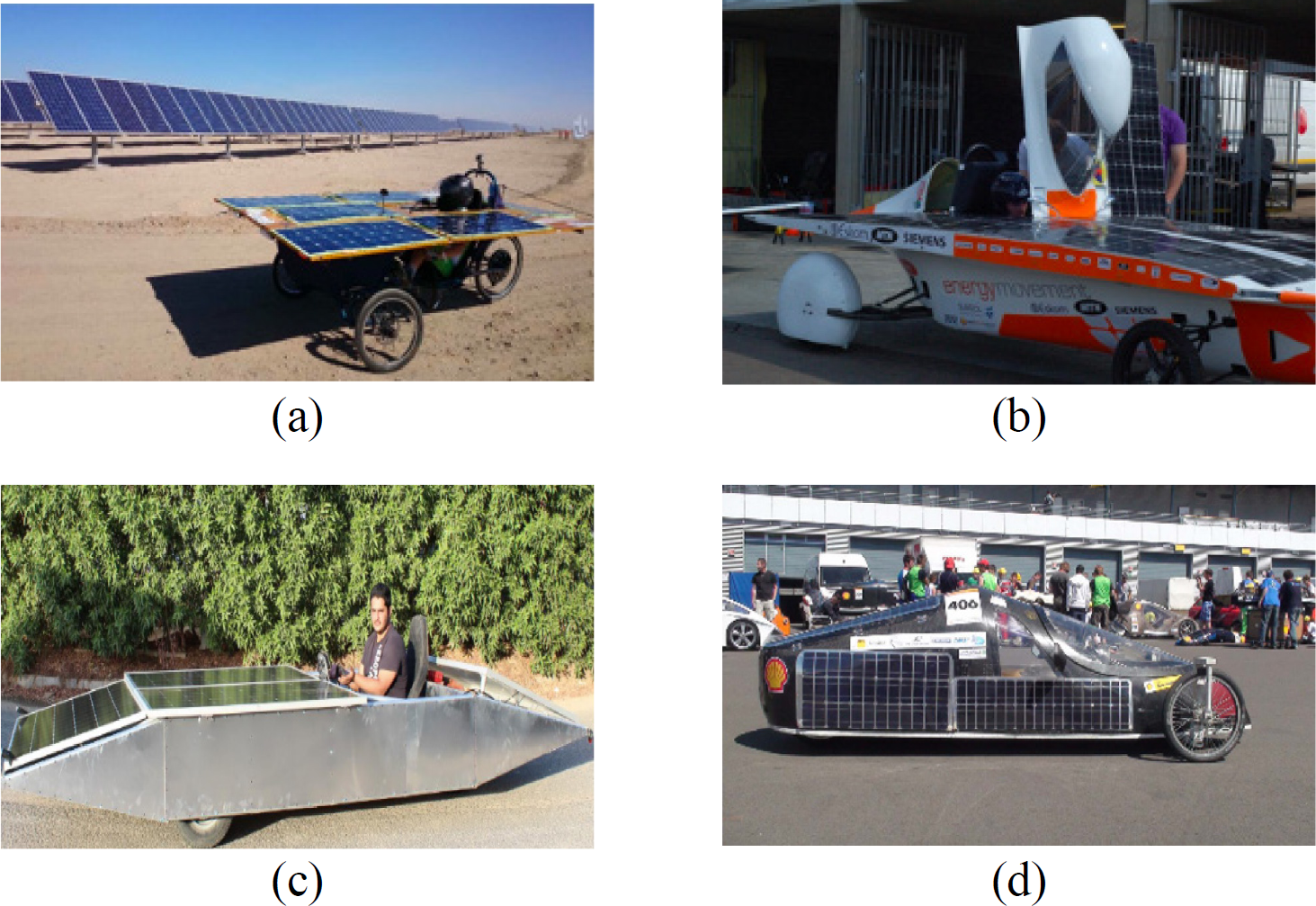
Students from Qatar university built a solar car, named GERNAS1 presented in Figure 1c, to take part in the Shell Eco-Marathon Europe race in Germany. Due to the requirements of the race, the team was restricted with the design and components used for the car. The team analysed the environment of the race to best identify the suitable design of the car which had a speed of 40 km/h. The solar panels used were semi-flexible with a 260 W of power at 12% efficiency. The team used three Lithium Polymer batteries that have 1.7 kWh total power along with a brushless DC motor operated at 7.5 kW mounted to the rear wheels to provide two gear stages [34].
In 2018, students from the American University of Kuwait assembled the solar car that underwent six stages before they reached their final product shown in Figure 1d. The solar car exhibited the following features: weight of 500 kg, four solar panels that had a 300 W capacity each, a brushless DC motor of 8000 W operated the car, and a Lithium-ion battery with a 96 V and 60 A ratings. These features, including the heavy weight and unsafe design, prevented the car from reaching its highest potential [35].
The American University of Ras Al Khaimah designed and implemented a hybrid solar electrical vehicle. The main objective was to design an economic car that can carry two passengers and still have enough power to reach a speed of 100 km/h. The total weight was 500 kg, had three solar panels that generated 960 W and the total power of the motor is 12 kW. The battery bank chosen to sustain the motors was 200 Ah, four 12 V batteries had to be used to reach the design requirements. The vehicle also struggles from the weight, high power consumption and can only function for 48 minutes [36].
This paper introduces a new approach to solar vehicle design and implementation, addressing the drawbacks discussed earlier.
Efficiency poses a significant challenge when it comes to harnessing solar energy for use in solar cars. Power gain of solar panels can be influenced by factors such as radiation and temperature, which are region-dependent rather than design-dependent. Expanding the solar area available on the car would increase its weight, necessitating more powerful motors that consume the already limited harvested energy. The proposed new design primarily emphasizes the enhancement of efficiency and reduction of total weight while considering power consumption demands.
In order to achieve an efficient car design, it is crucial to prioritize a lightweight structure. To reduce the overall weight of the car, it is divided into two primary segments: the shell, as depicted in Figure 2a, and a sturdy frame capable of supporting the car's weight. By reinforcing the areas experiencing the highest levels of stress, it is possible to reduce the weight of the chassis. Furthermore, the constant vibrations resulting from uneven terrain can lead to chassis fatigue. Therefore, the chassis must possess sufficient tensile strength to withstand shocks caused by potholes and road bumps. To ensure long-term structural integrity and prevent deformation, the overall yield strength of the pipe-shaped chassis must be sufficiently high. Iron, known for its high yield strength, is utilized instead of aluminium to avoid potential breakdowns.
The driver's maximum weight is assumed to be around 100 kg, thus becoming the highest load that the chassis should be designed to handle at any given point. To manage the weight and shocks effectively, the overall bending stress δb,max of the chassis should not exceed 2 MPa. The chassis dimensions are set at 2 × 2.5 m to provide adequate space for the components and the driver. The moment of bending M is derived from the tangential force Ftan exerted by the weight m and the moment of inertia Ic, as indicated in eq. (1). Substituting the variables into eq. (2) provides an estimation of the cross-sectional distance to the centre c as 0.204 m. The height of the beams is chosen to be 6 cm, as it can withstand more than 3 MPa of stress.
(1)
(2)
To minimize the drag losses resulting from aerodynamic friction with the front side of the car, the vehicle's overall shape was designed to resemble an air foil, as depicted in Figure 2b. An air foil essentially represents a cross-sectional portion of an airplane wing, characterized by a smooth and uniform surface. The key feature of this design is an arc with a shallow angle of attack, which optimizes the panel area [37]. Given that the sun in Kuwait, where the study had been conducted, generally shines at a vertical angle of 30° during most months, the arc was intentionally set at approximately 30°. The car's height and length were chosen to be around 3 m and 0.7 m, respectively. Figure 2c displays the final shell design.
Design and implementation of the solar car main frame: the chassis (a); shell design (b); final car with shell (c)
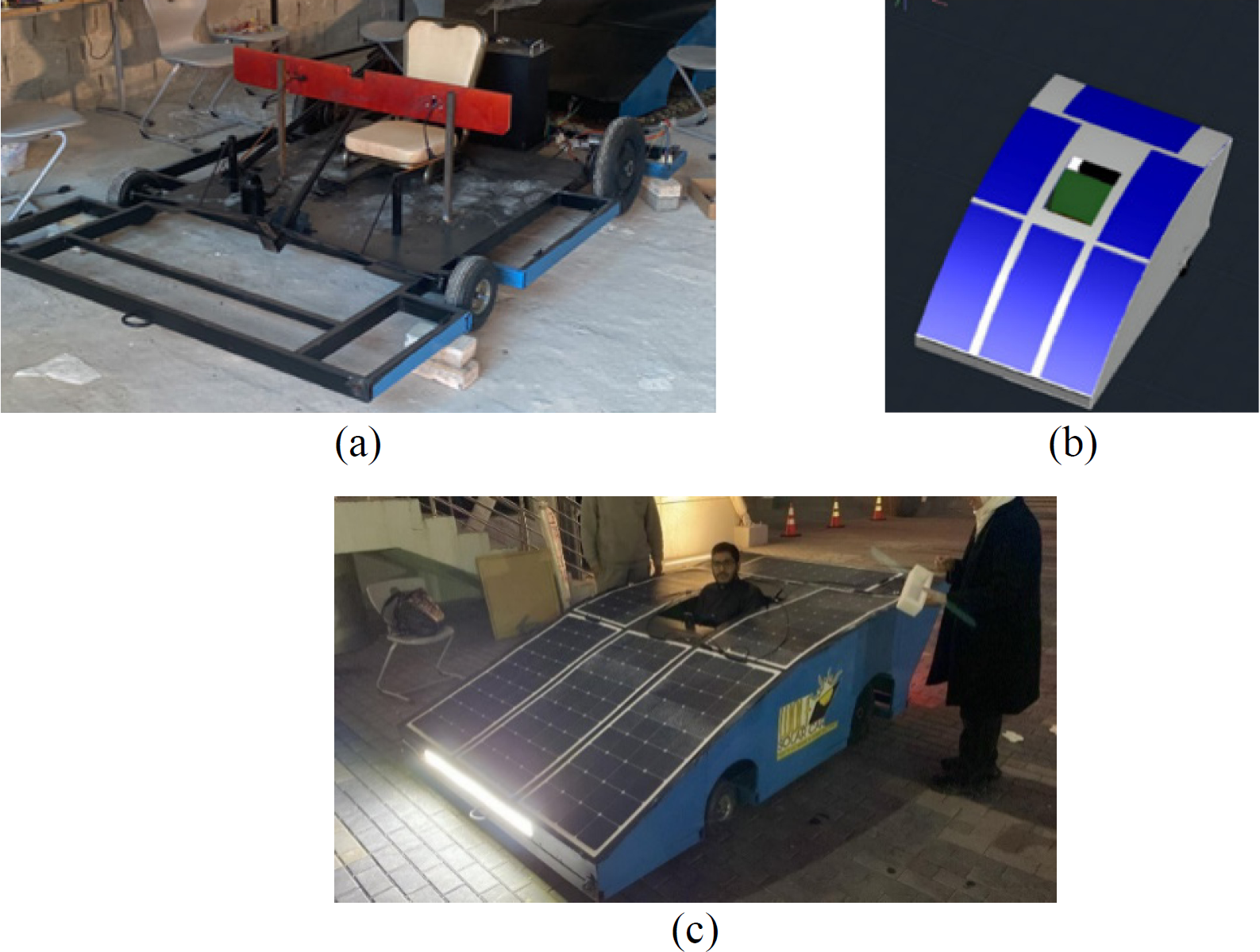
The applied forces acting on the car encompass acceleration, drag, and aerodynamic resistance. The total weight, including the driver, is approximated to be 260 kg. Considering the resistance coefficient 〖C〗_r between the tires and ground to be 0.03, and the body of the car’s body having a drag coefficient 〖C〗_D of 0.2. After calculating all the forces Froll , FDrag, and Facceleration, the mechanical power 〖P〗_mech required to propel the car can be estimated as depicted in eq. (3). However, since the motors are not ideal, the electrical power should exceed the mechanical power when accounting for the efficiency η. Related mechanical parameters and the range of required power for different vehicle speeds are presented in Table 1 and Table 2, respectively.
(3)
The list of desired mechanical parameters
Parameter |
Value |
---|---|
Mass (m) [kg] |
260 |
Velocity (Vm) [m/s] |
11.1 |
Rolling coefficient (Cr) [-] |
0.02 |
Earth gravity (g) [m/s2] |
9.8 |
Air density (ρ) [kg/m3] |
1.2 |
Drag coefficient (CD) [-] |
0.4 |
Frontal area (A) [m2] |
0.7 |
The required power for different speeds of the solar car
Speed [km/h] |
Solar HEV speed (Vm) [m/s] |
Power (Pe) [W] |
---|---|---|
10 |
2.778 |
239 |
20 |
5.556 |
642 |
30 |
8.333 |
1221 |
40 |
11.111 |
1990 |
50 |
13.889 |
2959 |
60 |
16.667 |
4142 |
70 |
19.444 |
5551 |
80 |
22.222 |
7197 |
90 |
25.000 |
9094 |
100 |
27.778 |
11254 |
The system begins the solar radiation being captured by the flexible SunPower ETFE Solar panels illustrated in Figure 3a. These panels are an excellent choice for the car due to their flexibility. Each panel can produce 135 W with an efficiency of 23%. To increase the total rated power to 810 W, six panels were installed on the car. In Figure 3b, the Lifepo4 51.2 V 100 Ah Lithium Battery 48 V 100 Ah is showcased, which not only supports the motor's power consumption at top speed but also matches the voltage rating of the panels. The solar panels transmit a portion of this energy as electrical energy to the Sunwhale Maximum Power Point Tracking (MPPT) depicted in Figure 3c. The main function of the MPPT is to regulate and maximize the charging of the panels while preventing overcharging of the battery [38]. Additionally, the option of charging from the main electrical supply is incorporated to facilitate charging during nighttime and adverse weather conditions.
The MPPT device effectively receives and regulates incoming energy, directing it to the battery when the source transfer switch is activated. Alternatively, the battery can receive energy through a manual direct plug if the switch is not enabled. To meet the motor's requirements, two QS 205 motors depicted in Figure 3d are selected due to their 88% efficiency, rated power of 1000 W, and ability to function as generators during braking. For adequate motor support and control, two Kelly KLS7218S controllers shown in Figure 3e are chosen, as a single controller is insufficient to handle both motors. These motors and controllers are designed to operate in all four quadrants, enabling forward and reverse motoring while also allowing the motor to function as a generator during braking intervals, thereby returning some of the kinetic energy back to the battery [39]. However, the manufacturer advises installing a disc brake for additional safety, as the electrical brake may require more time to bring the car to a complete stop. To ensure safety, it is crucial to avoid direct installation of the motor controllers and MPPT on metal surfaces. Instead, a non-conducting surface must be utilized for all high voltage components to prevent current flow through the chassis or shell [40].
Main electrical components: Sunpower ETFE solar panels (a); Lifepo4 51.2 V 100 Ah Lithium Battery 48 V 100 Ah (b); Sunwhale 20 A/30 A/40 A MPPT Solar Charge Controller QS (c); Motor 205 1000 W (d); Kelly controller KLS7218S (e)
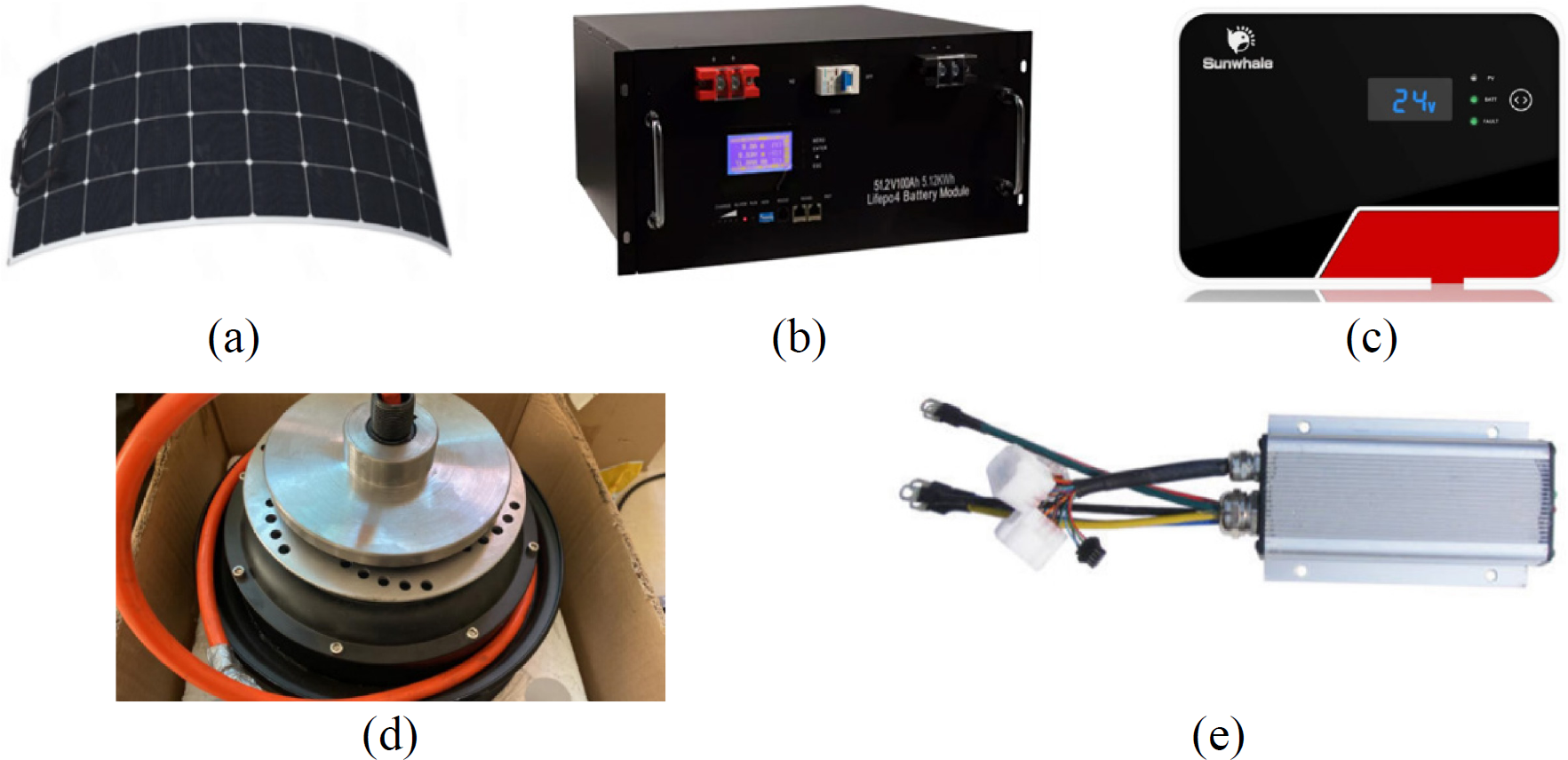
The design of the solar car system represents a broader perspective on traditional solar or electric cars. This car can be regarded as a hybrid vehicle that integrates solar power as a fundamental aspect of its performance, while also offering the option of direct plug-in charging. The block diagram of the solar car is illustrated in Figure 4. The system incorporates a manual source switch between the MPPT and the converter, enabling alternative charging methods for the solar car battery. Additionally, the car features two identical motor controllers paired with adjacent motors, contributing to a rear-wheel-drive experience. To safeguard the motor controllers, the system includes protective elements like an in-line fuse, which directly connects to the battery.
The solar car system diagram
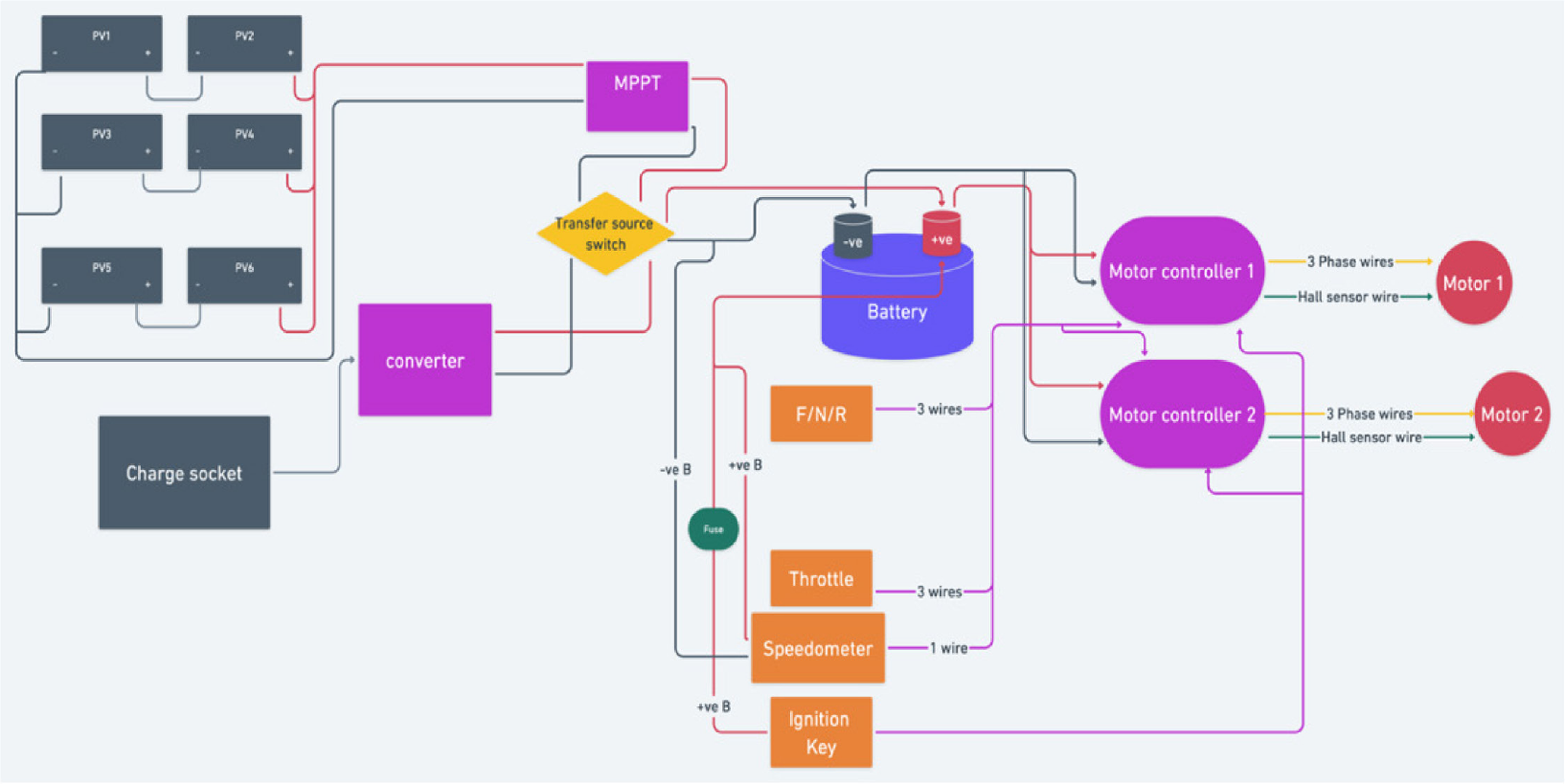
The software programming for the solar car primarily relied on prebuilt programs provided by the manufacturers of the selected components. These programs focused on two main elements. The first component was the motor controller, which utilized the user program developed by Kelly motor controller. This program offered various predefined parameter settings and included features that facilitated customization of the solar car's motor performance. The programming process encompassed four key steps. Initially, angle identification was conducted to ensure accurate motor readings and proper functionality. The second step involved setting vehicle parameters, including operational voltage limits and additional features to be integrated into the motor controller, such as specifying the type of gear to be used. The third step is the motor parameter setting which involved adjusting numerous parameters such as the number of motor poles. Lastly, the control parameter setting entailed configuring parameters like acceleration time.
The MPPT used was a smart device that was accompanied by a very handy user program named as ChargePro 2.0, which provides useful information regarding the solar panels' output, the level of the battery charging, and many other important information that could be accessed by the solar car user.
The testing of the PV system was done in December, the solar irradiance is rather low during the study time period. Since the battery can only store 5000 W, it is rather difficult to test the power gain for an entire month, so the study was simplified to observe Table 3 shows the average voltage and current readings of the MPPT reading during daytime. The maximum voltage appearing on the MPPT was never higher than 48 V and the current did not exceed 6 A largely due to the season, while the fluctuation on Table 3 are due to cloudy and rainy weather. The MPPT reading never dropped the voltage below 44 V, it can be neglected since the battery was not charging when the voltage was on that level.
MPPT readings
Time |
Average current, [A] |
Average voltage, [V] |
---|---|---|
7 AM |
2.6 |
46.7 |
8 AM |
4.3 |
47.3 |
9 AM |
3.7 |
47.3 |
10 AM |
4.6 |
47.6 |
11 AM |
5.8 |
47.7 |
12 AM |
6.3 |
47.8 |
1 PM |
6.2 |
47.8 |
2 PM |
4.2 |
47.5 |
3 PM |
2.7 |
44.0 |
4 PM |
4.5 |
47.5 |
5 PM |
4.3 |
47.6 |
6 PM |
1.3 |
44.5 |
The MATLAB model shown in Figure 5 is best used to verify the current input of the PV system, given that the average irradiance is around (400 kW/m^2). The output current is 7.564 A which can confirm the low current given in the actual study.
The solar system model
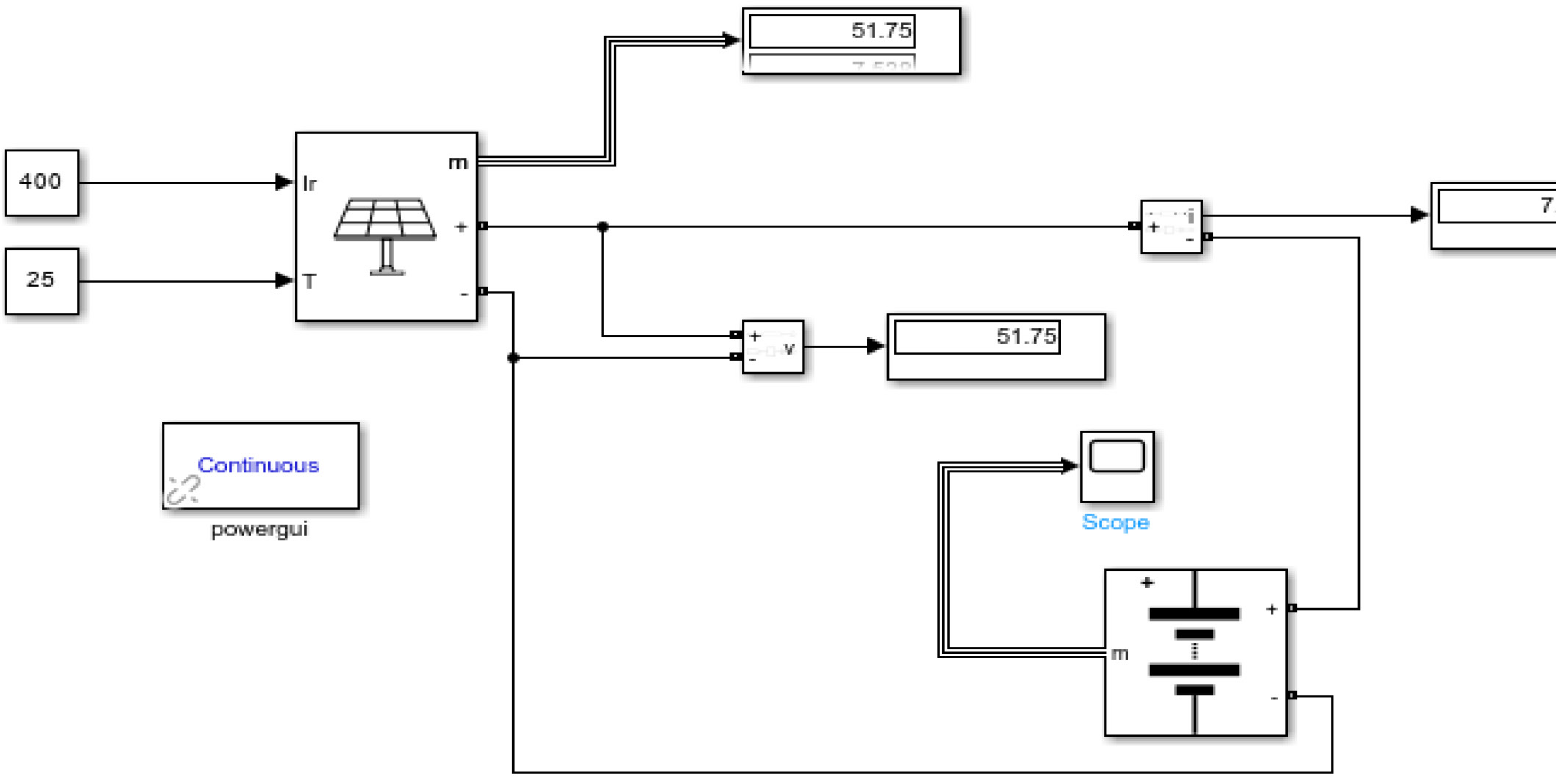
The primary objective of the proposed solar car design is to optimize efficiency by reducing weight and creating an electrical system that provides high-torque and low-power consumption. The chassis material and strength are carefully chosen to withstand prolonged stress and absorb shocks while driving on uneven terrain. The shell design mimics an air foil to minimize drag losses while maintaining a large surface area. The angle of attack is strategically adjusted to maximize the average solar power intake in the specific region. The power ratings of the motor are calculated based on the anticipated weight and desired speed of the car, ensuring compatibility with the motor controller and battery requirements. The battery and solar system are positioned in close proximity to the motor, ensuring that their capacity and rating can handle the power consumption demands of the entire system. Prebuilt software provided by the manufacturer is utilized, enabling customization of the system settings according to specific equipment parameters. The estimated charging time is determined based on the battery size and power generation capacity of the solar system. Likewise, the travel time is calculated considering the battery size and motor consumption.
To enhance the performance of the solar car, future work can focus on several aspects. Firstly, using PV panels with higher. Secondly, choosing thinner wheels can help minimize friction effects and improve overall efficiency. Additionally, reducing the structural mass of the car by utilizing a fiberglass shell to enhance performance. Implementing these features would undoubtedly result in notable improvements.
This publication was made possible by the support of the AUK Open Access Publishing Fund.
A |
frontal area |
[m2] |
Cr |
resistance coefficient |
[-] |
CD |
drag coefficient |
[-] |
c |
cross-sectional distance to the centre |
[m] |
Froll |
roll force |
[N] |
Fdrag |
drag force |
[N] |
Facceleration |
acceleration force |
[N] |
Ftan |
tangential force |
[N] |
Ic |
moment of inertia |
[m4] |
M |
bending moment |
[Nm] |
m |
mass |
[kg] |
Pmech |
mechanical power |
[W] |
Pe |
electrical power |
[W] |
VM |
motor speed |
[m/s] |
Greek letters |
||
δb,max |
overall bending stress |
[Pa] |
η |
efficiency |
[-] |
ρ |
air density |
[kg/m3] |
- Renewables 2023 global status report, 2022, https://www.ren21.net/gsr-2023, Accessed Dec. 15
Electric Power Systems (review of "Modern Electric, Hybrid Electric, and fuel cell vehicles: Fundamentals, theory, and design, second edition; Ehsani, Y.G. and emadi, A.; 2010) [Book news] ,IEEE Industrial Electronics Magazine , Vol. 4 (1),pp 75–75 , 2010, https://doi.org/https://doi.org/10.1109/mie.2010.936103
, Sustainable energy transition for renewable and low carbon grid electricity generation and supply ,Frontiers in Energy Research , Vol. 9 , 2022, https://doi.org/https://doi.org/10.3389/fenrg.2021.743114
, Optimal design of Electric Vehicle Charging stations considering various energy resources ,Renewable Energy , Vol. 107 ,pp 576–589 , 2017, https://doi.org/https://doi.org/10.1016/j.renene.2017.01.066
, Feasibility Study of a solar-powered electric vehicle charging station model ,Energies , Vol. 8 (11),pp 13265–13283 , 2015, https://doi.org/https://doi.org/10.3390/en81112368
, Hybrid electric vehicles for Sustainable Transportation: A Canadian perspective ,MDPI , , https://doi.org/https://doi.org/10.3390/wevj3030551
, On designing of the main elements of a hybrid-electric vehicle driving system ,Journal of Power and Energy Engineering , Vol. 02 (04),pp 103–112 , 2014, https://doi.org/https://doi.org/10.4236/jpee.2014.24016
, Energy management strategy implementation for hybrid electric vehicles using genetic algorithm tuned Pontryagin’s minimum principle controller ,International Journal of Vehicular Technology , Vol. 2016 ,pp 1–13 , 2016, https://doi.org/https://doi.org/10.1155/2016/4234261
, Different architectures and modes of operation of HEV based on permanent magnet-electric variable transmission with rule-based and Fuzzy Logic Global Control Strategy ,International Journal of Electric and Hybrid Vehicles , Vol. 4 (1),pp 69 , 2012, https://doi.org/https://doi.org/10.1504/ijehv.2012.047873
, Design and Implementation of Power Management System Utilizing Supercapacitors for Hybrid Vehicles. ,International Journal of Scientific Engineering and Technology , Vol. 3 (8),pp 1074-1077 , 2014
, Design and implementation of a thermoelectric- photovoltaic hybrid energy source for Hybrid Electric Vehicles ,World Electric Vehicle Journal , Vol. 3 (2),pp 271–281 , 2009, https://doi.org/https://doi.org/10.3390/wevj3020271
, Some issues on the design of a solar vehicle based on Hybrid Energy System ,International Journal of Energy Engineering , Vol. 2 (1),pp 15–21 , 2012, https://doi.org/https://doi.org/10.5923/j.ijee.20120201.03
, Journal of Sustainable Development of Energy, Water and Environment Systems - Volume XI ,J. sustain. dev. energy water environ, syst. , Vol. 11 (4),pp 1110479 , 2023, https://doi.org/https://doi.org/10.13044/2023.11.ed
, Environmental Impact of Solar and Wind energy- A Review ,J. sustain. dev. energy water environ. syst. , Vol. 10 (2),pp 1090387 , 2022, https://doi.org/https://doi.org/10.13044/j.sdewes.d9.0387
, Impact of Dust and Shade on Solar Panel Efficiency and Development of a Simple Method for Measuring the Impact of Dust in any Location ,J. sustain. dev. energy water environ. syst. , Vol. 11 (2),pp 1110448 , 2023, https://doi.org/https://doi.org/10.13044/j.sdewes.d11.0448
, Simple Photovoltaic Electric Vehicles Charging Management System Considering Sun Availability Time ,J. sustain. dev. energy water environ. syst. , Vol. 12 (1),pp 1110476 , 2024, https://doi.org/https://doi.org/10.13044/j.sdewes.d11.0476
, Improving the performance of photovoltaic panels using a direct water cooling system ,J. sustain. dev. energy water environ. syst. , Vol. 11 (4),pp 1110468 , 2023, https://doi.org/https://doi.org/10.13044/j.sdewes.d11.0468
, Energy Flow Management in a Smart Microgrid Based on Photovoltaic Energy Supplying Multiple Loads ,J. sustain. dev. energy water environ. syst. , Vol. 12 (1),pp 1110473 , 2024, https://doi.org/https://doi.org/10.13044/j.sdewes.d11.0473
, Voltage Quality Improvement Using Solar Photovoltaic System ,J. sustain. dev. energy water environ. syst. , Vol. 3 (2),pp 140-150 , 2015, https://doi.org/http://dx.doi.org/10.13044/j.sdewes.2015.03.0011
, Solar Desalination: Current Applications and Future Potential in MENA Region – A Case Study ,J. sustain. dev. energy water environ. syst. , Vol. 11 (2),pp 1100437 , 2023, https://doi.org/https://doi.org/10.13044/j.sdewes.d10.0437
, Solar model for Rural Communities: Analysis of Impact of a Grid-Connected Photovoltaic System in the Brazilian semi-arid region ,J. sustain. dev. energy water environ. syst. , Vol. 10 (3),pp 1090405 , 2022, https://doi.org/https://doi.org/10.13044/j.sdewes.d9.0405
, Analysis of Residential Rooftop Photovoltaic Systems Diffusion in India through a Bass Model Approach ,J. sustain. dev. energy water environ. syst. , Vol. 10 (4),pp 1080423 , 2022, https://doi.org/https://doi.org/10.13044/j.sdewes.d8.0423
, Prediction of Global Solar Radiation in India using Artificial Neural Network ,J. sustain. dev. energy water environ. syst. , Vol. 4 (2),pp 94-106 , 2016, https://doi.org/http://dx.doi.org/10.13044/j.sdewes.2016.04.0009
, Identifying Emerging Research Related to Solar Cells Field using a Machine Learning Approach ,J. sustain. dev. energy water environ. syst. , Vol. 4 (4),pp 418-429 , 2016, https://doi.org/https://dx.doi.org/10.13044/j.sdewes.2016.04.0032
, Analyzing the Role of Renewables in Energy Security by Deploying Renewable Energy Security Index ,J. sustain. dev. energy water environ. syst. , Vol. 11 (4),pp 1110463 , 2023, https://doi.org/https://doi.org/10.13044/j.sdewes.d11.0463
, Energy performance analysis of a solar refrigerator using ecological refrigerants ,J. sustain. dev. energy water environ. syst. , Vol. 11 (2),pp 1110446 , 2023, https://doi.org/https://doi.org/10.13044/j.sdewes.d11.0446
, Low Speed Orientation Control Using Variable Mass System: Application In Solar Panel ,J. sustain. dev. energy water environ. syst. , Vol. 11 (2),pp 1110456 , 2023, https://doi.org/https://doi.org/10.13044/j.sdewes.d11.0456
, Assessing Urban Households’ Willingness to Pay for Standalone Solar Photovoltaic Systems: A Case Study of Lagos, Nigeria ,J. sustain. dev. energy water environ. syst. , Vol. 7 (3),pp 553-566 , 2019, https://doi.org/https://doi.org/10.13044/j.sdewes.d7.0274
, Karameros, Athanasios Chassiakos, Stylianos Karatzas, Design and Evaluation of a Micro-Grid Energy Management Scheme Focusing on the Integration of Electric Vehicles ,J. sustain. dev. energy water environ. syst. , Vol. 11 (1),pp 1090413 , 2023, https://doi.org/https://doi.org/10.13044/j.sdewes.d9.0413
, Novel Design of Hybrid Single Slope Solar Distiller with Photovoltaic Powered Thermoelectric System ,J. sustain. dev. energy water environ. syst. , Vol. 12 (1),pp 1110474 , 2024, https://doi.org/https://doi.org/10.13044/j.sdewes.d11.0474
, Evaluation of Aerosols Impact on global and direct irradiance attenuation under clear sky condition: A case study in Morocco ,J. sustain. dev. energy water environ. syst. , Vol. 11 (1),pp 1100433 , 2023, https://doi.org/https://doi.org/10.13044/j.sdewes.d10.0433
, Design and implementation of the first Duoc-UC's solar energy powered car. ,In Tenth International Conference on Ecological Vehicles and Renewable Energies (EVER) ,pp 1-7 , 2015, https://doi.org/https://doi.org/10.1109/EVER.2015.7112978
, High Power Electrical Systems Design for a Solar Car: Designing and building the solar car IlangaI.I. ,In 2013 Africon ,pp 1-5 , 2013, https://doi.org/https://doi.org/10.1109/AFRCON.2013.6757697
, Design of Qatar University's first solar car for Shell Eco-marathon competition. ,In First International Conference on Renewable Energies and Vehicular Technology ,pp 49-54 , 2012, https://doi.org/https://doi.org/10.1109/REVET.2012.6195247
, Design, analysis, and mechanical assembly of a three-wheeled solar-powered electric vehicle ,International Journal of Computing and Digital Systems , Vol. 9 (3), 2020, https://doi.org/https://doi.org/10.12785/IJCDS/090315
, New Design and Implementation of a Solar Car of the American University of Ras Al Khaimah: Electrical Vision ,J. sustain. dev. energy water environ. syst. , Vol. 8 (3),pp 452-463 , 2020, https://doi.org/https://doi.org/10.13044/j.sdewes.d7.0281
, - , Fundamentals of Aerodynamics., 2001
- , Design and analysis of MPPT Charge Controller, 2021 International Conference on Simulation, Automation & Smart Manufacturing (SASM), 2021
- , Electric Motors and Drives Fundamentals, Types and Applications., 2006
Electrical Safety of Electric Vehicles. In IEEE/IAS 53rd Industrial and Commercial Power Systems Technical Conference (I&CPS) , 2017, https://doi.org/https://doi.org/10.1109/ICPS.2017.7945
,