To mitigate climate change, a transformation towards a low-carbon economy and society is urgently needed and requires serious changes over all sectors of the economy. For this transformation, the energy sector plays a key role. To reach the goal of limiting global temperature increase to well below 2 °C, energy-related emissions must drop by over 70% in the next 35 years. For a deep decarbonisation, the way energy is produced and consumed must change fundamentally. An energy system transition is unavoidable and comes along with substantial changes of the energy infrastructure [1].
Energy infrastructure projects have a long-time scale and require large investment decisions to be made today. Decisions about economic investment and energy policy shape the future of energy systems for decades. The decision-making for infrastructure investments is guided by assumptions about future developments which lead to a great diversity of energy futures [2]. From an economic point of view, whether and when it is promising to invest depends on expected future returns. Besides the criterion of profitability, the chances of a successful implementation also seem to determine the investment decision. In this context, not only economic factors such as demand or the real interest rate, but also non-economic factors like social acceptance and planning certainty play a crucial role [3].
However, due to increasing uncertainty and complexity related to the development of energy systems, different assumptions and thus future scenarios are possible [4], [5]. Uncertainty and complexity pose major challenges for traditional research approaches and decision-making in companies and public policy, since they can hardly be grasped with traditional methods. Due to the diversity of energy transition scenarios and associated uncertainties, the prevailing understanding of an optimal decision-making' derived from one best-solution' scenario does not work. There is the risk that energy strategies and related large investments are based on inaccurate forecasts and thus miss the intended goal. Considering the urgent need for actions to combat climate change, this might have fatal consequences [2].
This paper assesses how economic energy research can cope with this challenge and accelerate a deep decarbonisation in the energy sector. The authors argue that economists can contribute to accelerated emission reduction by emphasising more how the transformation can be achieved. It implies to consider the feasibility of a successful implementation. To shift the focus to aspects of implementation and feasibility, a more holistic approach is favourable. The line of argumentation runs as follow. Policies that are optimal from an economic perspective are not per se the optimal solution for society. There is the risk that optimal policy recommendations will not be chosen by decision-makers or fail when it comes to the implementation. A theoretical optimal policy that cannot be implemented, can hardly be a suitable recommendation to mitigate climate change. An analysis of future development represents the necessary base for a policy evaluation. Therefore, economic policy evaluations and thus advice should focus on identifying feasible instead of optimal policies. Thus, the paper's main proposition is that to identify feasible policies, economic analysis should include four core features:
Complexity;
Non-economic aspects;
Uncertainty;
Stakeholders.
In this paper, the authors argue that qualitative scenario development represents a foresight approach that meets these requirements. The objective of the paper is to show that qualitative scenario development is a promising alternative to conventional forecast methods to evaluate energy policy. The question of how feasibility and the core features can methodologically be implemented into the analysis of energy transitions is key. The authors develop qualitative socio-technical scenario for a case study. These scenarios are used to assess gas infrastructure modifications in Germany which are part of a European H2/Carbon Capture and Storage (CCS) infrastructure. The qualitative scenarios are not the papers main focus, they are used as an application to demonstrate the value of qualitative scenario development ‒ especially when compared to traditional Cost-Benefit Analysis (CBA)-based approaches.
By talking about conventional economic methods, this paper refers to quantitative neoclassical economic models. They can be described as an approach of optimisation in a fully characterised closed system. Agents are typically assumed to be fully rational, maximise utility and have fixed preferences which lead to a stable equilibrium. Furthermore, neoclassical approaches often neglect non-economic aspects, behavioural changes and transition dynamics, which play a crucial role in transformation processes. Commonly used econometric forecasting models are either explicitly or implicitly based on the assumption of structural stability and equilibrium [3].
In the literature, no economic study has yet analysed the implementation of gas infrastructure modifications with hydrogen and CCS technologies focusing on political feasibility. The paper is the first one providing a methodological discussion to improve economic energy policy by developing qualitative scenarios that enable deep decarbo-nisation in the energy sector.
There are many studies in climate change research and related fields, that use scenario analyse to assess climate mitigation strategies. Especially energy scenarios are commonly used to evaluate energy strategies [6]. Quantitative scenario approaches like energy modelling and techno-economic scenarios still dominate the scientific discourse [7]. Bauer et al. [8], for example, quantify socio-economic pathways using energy system models integrated with models of land use and the macroeconomy to analyse global emission mitigation policies. Robinius et al. [9] develops techno-economic pathways using energy modelling to identify cost-effective emission reduction strategies. Qualitative scenario approaches are mainly used in the field of future studies, where they originate [10], but gain more and more importance. O'Neill et al. [11] develop qualitative narratives for shared socio-economic pathways. Weimer-Jehle et al. [12] combine a quantitative energy modelling with a qualitative scenario approach to propose sociotechnical energy scenarios.
This development results from the understanding that social acceptance or political feasibility of low-carbon transformation are essential for a successful implementation of climate strategies. These factors are hardly considered in quantitative analyses [13]. Currently, a rethinking of energy research takes place. The attention is changing from technical and economic feasibility to a more holistic assessment [14]. Similarly, Schubert et al. [15] observes that research questions are shifting from if' to how' (or under what conditions) the transition can be realised by policy-makers. It implies addressing social acceptance and political feasibility.
Consequently, the young field of transition studies is growing. The difficulty of understanding the transition comes with the fact that different aspects such as economic development, technical innovation or political change influence the transformation [16]. A variety of disciplines, e.g., economics, politics, geography, sociology or philosophy now study the transition from different perspectives using different methods [17]. This diversity is due to the disciplines' different scope and focus, e.g., sustainability transition' [18], energy transition' [19] or low-carbon transition' [13]. Related to the rethinking of energy policy analysis, the search for the appropriate method is ongoing and represents a central point of the development [12]. Thus, reflections on the methodology for understanding the low-carbon transformation are required. Considering different theories and methods is an integral part of transition research and exceeds disciplinary boundaries [20].
In economics, however, qualitative scenario approaches are barely mentioned. Donges and Freytag [21] describe qualitative scenario analyses in a textbook as an alternative to traditional forecasts to provide orientation for decision-maker to cope with unexpected development. According to them, scenario analysis could have helped to avoid false estimations in the context of the transformation process related to the reunification of Germany. Still, traditional forecasting methods dominate the discussion about the future development. It contrasts with the often-raised critique that these methods are hardly helpful in the case of climate change and energy analyses [22]. The paper argue that the science of economics now lags behind the rethinking of energy (policy) research taking place in others research field. In economic literature, there is a lack of methodological discussion of economics foresight methods such as qualitative scenario approach.
This paper addresses the lack and makes an original contribution to the existing literature in energy policy research as well as economic foresight in different ways:
Firstly, it contributes to the catch up of economics with the rethinking of energy policy research that takes place in other disciplines. It does so in a methodological sense and proposes a way how to integrate political feasibility in the economic assessment of energy policies;
Secondly, the paper represents an example of how to develop qualitative socio-technical scenarios from a complexity economics perspective, as a response to the limitations of traditional quantitative methods. By doing so, it contributes to the improvement of energy scenarios and energy policy research by including stakeholders, non-economic aspects, complexity and uncertainty;
Thirdly, the approach of qualitative scenario development is applied to the case of infrastructure modification in Germany. In this way, new insights to the current debate on factors that foster or hinder deep decarbonisation of the energy system in Germany are provided.
The paper is structured as follows. Section 2 provides an overview of qualitative scenario development in general and describes the approach of the case study in more detail. In addition, the paper shows in how far qualitative scenario development is used to incorporate the four core features by referring to the case study example. In section 3, the results of the case study are contrasted exemplarily with a conventional cost-effective optimisation analysis. By doing so, the value of qualitative socio-technical scenarios as economic foresight method is shown. Section 4 discusses challenges and chances of qualitative scenario development as a promising alternative to economic forecasting that go beyond the case study. The paper ends with concluding remarks.
This section introduces the method of qualitive scenario analysis in general. The approach of this paper's case study is described in more detail. It is shown how the scenario analysis allows to consider the four core features.
The concept of scenarios has its origin in the field of future studies, which is also called futurology [23]. There is no single scenario approach in the literature but many divergent classifications and definitions [24]. In general, scenarios describe "a possible situation in the future, based on a complex network of influence factors" [25]. In this sense, they "reflect different assumptions about how current trends will unfold, how critical uncertainties will play out and what new factors will come into play" [26]. Unlike forecasting, scenarios do not claim to accurately predict the future, nor to be self-fulfilling or complete. Scenario methods normally do not aim to identify the most likely' future. They rather explore a wide range of plausible' future developments without estimating and assigning probabilities [27].
From a methodological perspective, scenario development represents a tool to explore how the future might look like [28]. It allows to consider all core features, which will be shown subsequently. Qualitative scenario development represents a promising alternative to quantitative forecasting methods in general and a suitable tool to assess energy strategies and policies. As complexity and uncertainty are inherent elements of energy systems [29], qualitative scenario development also improves the development of energy scenarios [7] and contributes to understanding energy system transitions.
Scenarios can have different functional purposes. Kosow and Gaßner [26] analyse the purpose of scenarios and categorize four related types of functions. Firstly, scenarios can have an explorative function' in the sense that they expand our knowledge about the present. According to ICIS [30], they also enable to identify limits of knowledge by highlighting uncertainties and complexity. Secondly, scenarios have a communicative function', since they require the scenario team to discuss and integrate different perspectives as Gaßner and Steinmüller [31] explain. Thirdly, by helping to assess the desirability of possible futures [32], scenario development has a goal setting function'. Lastly, scenarios allow to assess different strategies as Eurofound [33] highlights and thus has a decision-making function'.
Boerjeson et al. [34] distinguish between three main types of scenarios. This typology is based on different questions posed by the developer: what will happen-questions are answered by predictive scenarios' and aim to predict what will happen in the traditional sense of a quantitative forecast. Contrary, explorative scenarios' ask what can happen and normative scenarios' address the questions of how a specific goal can be reached.
The process of qualitative scenario development is divided into different phases. The number of phases and related steps vary depending on the specific approach [35]. Broadly speaking, the scenario process consists of three main phases, namely:
Idea creation and project definition;
Idea integration and pathways identification;
Scenario description and evaluation [36].
The scenario phases can consist of different steps. Schwartz [37] uses a process of 10 steps. Others also use an eight-step scenario process [38]. Besides, a wide range of tools and methods is used including the participation of external experts and stakeholders [39]. This methodological flexibility represents a main strength of the scenario approach and allows to customise the process for a particular purpose.
Despite the diversity of methods, scenario development approaches apply a backward-looking perspective from an imagined future to the present. After developing multiple scenarios, the desirability of each one is assessed. Hence, strategies to reach a desirable future development and avoid undesirable developments can be identified [40].
Working backward implies that the future is unpredictable [35], but malleable. This view represents the middle positions of three different views about the future. The future can be either predictable, malleable or evolutive. Compared to traditional forecasting, the future is not regarded as predictable. Therefore, precise calculations via a statistical trend extrapolation are hardly possible. The future is not evolutive in the sense of being fully chaotic and uncontrollable. Instead, the future is assumed to follow certain dynamics, so that the development can partly be shaped by the interaction of different actors [41]. Following this understanding, the qualitative scenarios developed in this study aim to explore possible future outcomes and thus, describe explorative scenarios'.
Describing the paper's approach and process of scenario development in more detail also shows how the four core features are considered. For the paper's case study, qualitative socio-technical scenarios for the economic assessment of different gas infrastructure modifications in Germany were developed. The papers' case study belongs to a broader interdisciplinary research project. The German gas infrastructure is part of a future European CCS/H2 infrastructure linking Germany, Norway, Netherland, the UK and Switzerland. Focusing on the methodological perspective, a deeper discussion of the different infrastructure options and the link to the other countries' infrastructure strategies exceed the scope of this paper. The process of scenario development consists of four phases, as displayed in Figure 1.
Phases of qualitative scenario management (authors' own contribution)
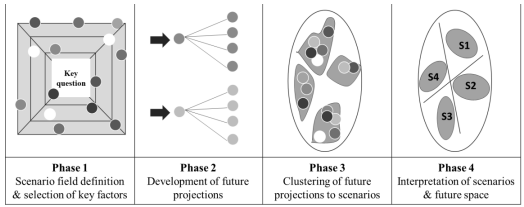
The scenarios are developed by a core scenario team of three economic researchers (the authors). Scientists from engineering, social science and law, as well as external experts joined the process at different stages. The first phase comprises the scenario field definition, and selection of key factors. The following scenario field definition includes key aspects such as the time horizon, the topic and the following scenario objective: The scenarios describe alternative future developments of conditions that are relevant for a gas infrastructure modification for the year 2035 which are in line with the German energy transition and sector coupling' [42]. Subsequently, different steps of the scenario development are linked to the four core features.
Complexity results from the interconnectedness of systems, sectors and related non-linearities as well as feedback mechanism. Increasing complexity especially applies to environmental systems and respectively energy systems [29]. This is due to the fact that energy systems are embedded in a socio-economic context [7], undergo rapid technological progress and depend on the interaction of heterogeneous agents [43] and climate dynamics [44].
Qualitative scenario development grasps complexity related to the development of energy system transformation in different ways. McInerny et al. [45] argues that visualisation is a technique to capture complexity, since it helps to clarify complex (inter)relations. Visualising the scenario field in form of a system image captures the complexity of the scenario objective. In a structured hierarchical way, system levels and topic areas are defined (Figure 2). The challenge is twofold. On the one hand, developing a system image for the gas sector requires to identify relevant sectors and actors, their interconnection, but also background conditions. On the other hand, these aspects need to be visualised in a systematic way by prioritising system levels. In this sense, developing a system image represents a tool of thought. It helps the scenario developers to test their initial intuition. Visualising can reveal limits of knowledge, inconsistencies, unclear relations and uncertainties.
System image (authors’ own contribution)
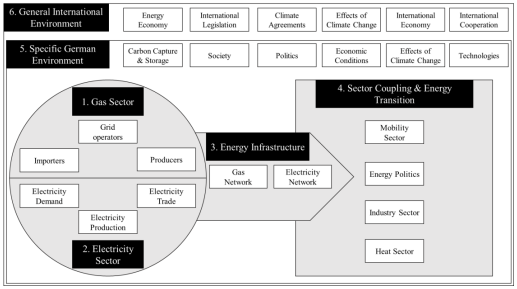
The paper's system image highlights the importance of the energy sector as the focal point of analysis. The energy sector is characterised by close relations between the gas and electricity sector which form system level 1 and system level 2. The energy infrastructure (system level 3) links the energy sector on the supply side with the demand of coupled sectors (system level 4). Since energy politics mainly shape the energy transition and thus the demand and supply side, it represents a central element of the system level 4. System levels 1-4 form the core area of the scenario field. System levels 1-4 are embedded in the specific German environment (system level 5) and the general international environment (system level 6). System levels 5 and 6 are rather general and comprise relevant framework conditions. Thus, creating system images broadens the perspective from a rather narrow techno-economic focus to a more holistic socio-technical perspective.
By moving from a techno-economic to a socio-technical perspective, non-technical and non-economic aspects need to be considered, too. This new perspective is due to the fact that climate change concerns the society and economy in all aspects. Thus, assessing energy transformation policies is an interdisciplinary task [7]. Energy systems are embedded in a wider socio-economic context, which is why social aspects such as behaviour and social acceptance are essential. Hence, including also non-economic aspects in economic energy policy evaluation is key.
Referring to the paper's case study approach, non-economic aspects are displayed in the system image and specified in form of key factors. In a literature-based brainstorming process, the scenario team listed 111 influence factors. This number of influence factors were condensed to 52 and matched to all system levels and topic areas. By conducting an interconnection-relevance analysis, the core team reduced the 52 influence factors in a more systemic way. In a 52 × 52 matrix, the core team ranked the influence from each factor on all other factors. A range from 0 (no influence) to 3 (strong, direct influence) was used. As a result, each influence factor is described by an activity score and a passivity score. While the activity score describes the factor's direct influence on other factors, the passivity score defines the influence of other factors. These scores allow to classify the influence factors according to their system relevance in four categories (Figure 3). Influence factors with the highest system relevance, namely system nodes, represent the driving forces of the scenario field. The interconnection-relevance analysis for the case study yields 23 key factors which are further explained in the result section.
System relevance analysis (authors' own contribution)
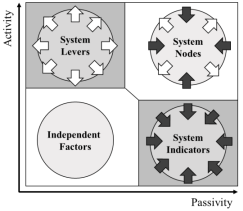
In phase 2, for each of the 23 key factors possible future developments in form of future projections are defined. In this way, uncertainty related to future development is considered. Considering uncertainty is important, because today's reality is characterised by intensifying uncertainties due to climate change, globalisation, economic instabilities, and new technologies. Therefore, decision-makers are faced with the challenge of how to adapt the decision process to an uncertain future [46]. For policy-makers, uncertainty reveals itself in a knowledge gap between what they actually know and what they need to know for decision-making [47]. Especially social and economic systems cannot be understood without dealing with uncertainties. Due to the interconnection of different systems, consequences of policy-making are fare reaching.
There exist different types of uncertainty. According to Walker et al. [48], uncertainty can be classified in four levels that lie between the extremes of determinism (knowing everything) and total ignorance (knowing nothing). Level 1 and 2 uncertainties can be formalised in statistical terms. Trend-based single forecast with a confidence interval (level 1) or assigning probabilities to alternative outcomes (level 2) can be used. Level 3 and 4 uncertainties represent deep uncertainties that cannot be captured in form of statistical probabilities. Level 3 uncertainties can be described by plausible futures without assigning probabilities. For Level 4 uncertainties, predictions about plausible development is not possible [48]. To cope with a high level of uncertainty, a more flexible and adaptive approach to assess future developments and related strategies is needed [49]. Scenario approaches are widely regarded as suitable and flexible tool to deal with high level of uncertainty [50].
On an abstract level, scenario development captures uncertainty in different ways:
It helps to identify and understand uncertainty;
It avoids missing unknown opportunities by showing all possible developments;
It broadens the researcher's horizon by identifying unexpected future developments [49].
Instead of only reacting to a single predicted future [46], scenario approaches encourage decision-makers to accept uncertainty. Uncertainty is not seen as a threat, but as an opportunity to shape the future. The paper's case study on decarbonising the German energy system also involves many uncertainties. Most of them represent level 3 uncertainties: how will the energy economy develop over time with regards to, e.g., energy prices, energy mix and energy demand? How will public acceptance develop regarding sustainable lifestyle or the use of CCS and hydrogen technologies? How will macroeconomic conditions, such as, e.g., GDP, the interest rate or the investment environment develop? How will the political environment develop? What role will, e.g., phase in/phase out of renewable gas or subsidies for low-carbon technologies such as e-mobility play? How will the demand for clean energy and hydrogen develop over time? These questions exemplarily demonstrate the uncertainty inherent in energy systems' transitions.
On a practical level, uncertainty is considered in phase 2 in form of future projections. Future projections describe possible pathways for each key factor. While the aim is not to identify the most likely future in terms of probability, it is about developing a holistic picture. This picture also includes extreme events. To do so, 4-5 projections are assigned to each key factor. For the key factor Realisation of National Climate Goals', for example, 4 projections are plausible. These are combinations of two dimensions which both exhibit two manifestations. The first dimension displays potential changes of current climate goals and the second dimension captures the level of realisation. In total, 100 projections sketch the potential development pathways of all 23 key factors.
Hence, in phase 3, a consistency analysis§ was used to assess the compatibility of different key factors' projections. Figure 4 displays an excerpt and shows the consistency check of two key factors' future projections.
The consistency matrix (authors' own contribution)
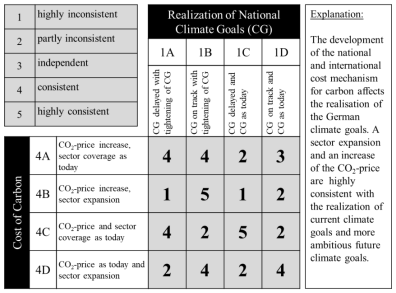
The logical occurrence of two projections within one future scenario is determined by assigning values from 1 (highly inconsistent) to 5 (highly consistent). In a next step, highly consistent future projections are combined to projection bundles, so called raw scenarios. Each raw scenario comprises one future projection of each key factor. Based on a cluster analysis, the number of scenarios was reduced to six (see result section). Phase 4 consists of a deeper analysis of the scenarios for the purpose of evaluating the different infrastructure options, which goes beyond the scope of this paper.
The stakeholder perspective is a core element of the case study process. It assumes that the influence of different stakeholders plays a crucial role when it comes to political decisions and their implementation. Ignoring stakeholders' interests increase the risk that the related policy cannot be successfully implemented and can result in protests [51]. Especially for the realisation of large infrastructure projects, social acceptance plays a crucial role [52]. Hence, the stakeholder perspective should be an integral part of the paper's case study and the assessment of energy policy.
Different stakeholders can get involved in the process of developing scenarios through participatory methods. Although participation is commonly used in the field of scenario development, it is unusual in science of economics. Participatory methods offer a possibility to generate qualitative data that are required for the scenario development [53]. Furthermore, different types of knowledge and perspectives can be synthesised. Divergent beliefs and concepts can be identified and implicitly reveal conflicting view. By bringing together different participants, participation can enrich the process and helps to avoid common biases [54]. There are different participatory techniques such as workshops or interviews which can be applied to different process phases. For the quality of the results, the design of the participation [55] and the choice of participants is decisive but often neglected.
Referring to the case study example, the stakeholder perspective is included in a practical and theoretical sense. As Table 1 displays, the authors applied participatory methods at different stages of the scenario process.
Overview of participation
Participant category | Background | Number of scientists key factors-feedback | Number of scientists, business representatives, evaluation workshop |
---|---|---|---|
Core scenario team | Economics | 3 | 3 |
Case study team | Social sciences | 2 | 2 |
Law | 2 | - | |
Engineering | 2 | 1 | |
Total internal participants | 9 | 5 | |
External participantss | - | ||
Scientific institutes | 2 | ||
Science | 1 | ||
Public company energy sector | 1 | ||
Private company gas sector | 5 | ||
Total external participant | 9 | ||
Total | 9 | 14 |
The case study team provided feedback on the conceptual work of phase 1 and 2 in an open workshop discussion. To broaden the economics perspective, the core scenario team discussed the completeness of the system image and key factors (phase 1). In phase 2, the discipline specific feedback supported the design of future projections of non-economic issues, e.g., social acceptance. Following Ernst et al. [7], the participation in the first participatory phase was restricted to scientists from the interdisciplinary case study team. The reason for this is that they are trained to work on an abstract analytical level and familiar with case study details. In the second participatory phase, the participation was extended to external stakeholders who discussed the desirability of the 6 scenarios. The choice of participants aimed to bring together professional expertise in energy research and related sectors, as well as representatives of social actors.
In a theoretical sense, the identification of stakeholder is part of the process of scenario development. To represent the influence and possible action of different agents related to gas infrastructure modification, the core team identified stakeholder groups (see result section).
The section shows that qualitative scenario analysis is a promising foresight method and an alternative to conventional approaches to evaluate energy policy. It also presents the six qualitative scenarios of the paper's case study in brief. A comparison between this paper's case study and a conventional study demonstrates three limitations of the latter and shows the value of qualitative scenario analysis.
This paper' scenario development results in six qualitative socio-technical scenarios, which are displayed in Table 2. From a systems perspective, these scenarios can be interpreted in terms of their overall level of low-carbon transformation. The overall level of transformation appears to be most characterising as it mainly determines the feasibility of this case study's different infrastructure options. To be more precise, the further a country's transformation towards more sustainability has progressed, the more feasible it is to implement extensive infrastructure modifications. Although there is no single most important factor, the scenarios reveal that the stakeholder dynamics play a central role. Stakeholder dynamics mainly determine the scenario setting and thus the overall level of transformation. Differences in the overall level of transformation can be explained by varying stakeholder dynamics displayed by different levels of conflict and engagement. The analysis shows that availability of technologies plays a minor role, which is in line with the paper's understanding of political feasibility. Whether or not low-carbon technologies are used depends on other key factors such as public acceptance or subsidies for transformation technologies. For modifying the gas infrastructure, the broader context, legal framework and long-term planning revealed to be crucial. Furthermore, the engagement of all stakeholder groups towards the transformation is relevant. This means, on the one hand, that the bottom-up commitment of the economy and of society is not sufficient to foster a high overall level of transformation (scenario 6), as long as political commitment is missing. On the other hand, political decisions are necessary but not sufficient for a high level of transformation (scenario 5), which also requires economic and societal commitment.
Socio-technical scenarios | Level of transformation | Level of engagement | Level of conflict |
---|---|---|---|
Fossil revival instead of green progress | 0 | 0 | 0 |
Technology-open green transformation | 2 | 2 | 1 |
Green transformation with hydrogen | 2 | 2 | 0 |
Incremental green transformation | 1 | 1 | 1 |
Top-down effort & conflicting interests | 1 | 2 | 2 |
Bottom-up effort & political inaction | 1 | 1 | 2 |
0 = no progress, 1 = low level, 2 = high level |
In scenario 3, for example, a low-carbon transformation focusing on hydrogen-based energy and technologies takes place. The government, industry and society are highly committed to foster the low-carbon transformation. The public and the strong green-lobby groups support the decision of the government to expand renewable energies and to phase-in renewable gases. Government's clear course provides planning security for the energy sector and investors. First H2 production plants are profitable and new infrastructure plans to adjust to hydrogen are about to be implemented. Extensive incentive programs for hydrogen technologies and application in the heating and mobility sector directs Germany to a low-carbon hydrogen future. Electrification plays a minor role, after subsidies for bridging electrification technologies expire. In contrast to scenario 3, scenario 2 shows a technology-open transformation. Government and industry follow a dual-track and promote both electrification and hydrogen-based technologies. In the mobility and heating sectors, both low-carbon technologies are widely used and almost replaced fossil applications. Regional projects in both technologies are preferred over large-scale national project to stay technology open.
While scenario 2 and 3 can be interpreted as best-case scenarios, other developments are also possible. Scenario 1 presents, as the name fossil revival instead of green progress' indicates, a negative development. A worsening of the current status quo took place where the whole society jointly turns away from sustainability and insists on fossil fuels again. The other scenarios are characterized by conflicting stakeholder interests and different levels of commitment. In scenario 5, for example, the government is highly committed to foster the low-carbon transformation, but is faced with strong opposition from fossil lobby groups. Fossil lobby groups actively hinder the transformation whereas green interest groups have difficulties to unit in order to build a strong countermovement. The public is open for new technologies but is not satisfied with the government's top-down strategy. Extensive governmental resources are used but show only little effects.
When talking about conventional approaches, the paper refers to mostly neoclassical-dominated research, that consists of an economic CBA. In the classical CBA, costs and benefits are expressed in monetary terms. Based on the costs and benefits, economists recommend the policy or project which is most desirable for society from an economic perspective. Policies with a positive net-benefit, i.e., whose expected benefits outweigh the expected costs enter the analysis. The option with the highest net-benefit is recommended. Thus, desirability is related to the maximisation of welfare, i.e., the minimisation of costs or maximisation of benefits.
In other disciplines and in practice, the economic CBA approach is also widely applied. CBA-based optimisation approaches are common practice in energy system and energy policy research. Due to the interdisciplinary character of this research, other aspects, i.e., techno-economic considerations are integrated. The recent study of Robinius et al. [9] combines a CBA-based optimisation approach with energy modelling. It identifies techno-economic pathways for the realisation of normative climate target in Germany. The following comparison with this paper's case study has three reasons:
To assess limitations of the conventional approach;
To demonstrate the value of qualitative scenario development;
To indicate how this paper's case study would change applying a conventional approach.
Despite major methodological differences, both studies have a similar thematic focus, which makes a comparison interesting (Table 3). Both studies cover the decarbonisation of the German energy system focusing on the energy infrastructure and H2 as a low-carbon energy carrier. In contrast to this paper's case study, Robinius et al. [9] aim to identify the most cost-effective CO2 reduction strategies for the energy sector in line with Germany's climate goals in 2050. Two CO2 reduction scenarios are at the centre of assessment, namely scenario 80 and scenario 95. The scenarios are subject to a German emission reduction target of 80% and 95%, respectively. The criterion of cost-effectiveness determines the optimal transformation pathways to realise each scenario. Robinius et at. [9] also acknowledge complexity and uncertainty in different ways than this paper's case study. To analyse complexity and cross-sector optimisation, a model family combines different energy models. The model family assesses the energy demand, Power-to-X (PtX) pathways and simulates the future energy market, which leads to a high level of detail on a regional level. Contrary, this paper's scenario stays on a rather abstract level. Robinius et al. [9] emphasize that future development is characterised by uncertainty. However, by concentrating only on data uncertainty related to future energy cost, they bypass the problem of fundamental uncertainty.
Comparison of alternative approaches
Robinius et al. [9] (2019) | Hoffart et al. (2020) | |
---|---|---|
Aim | Identification of cost-effective CO2 reduction strategies | Identification of key factors and dynamics for an energy system transformation with H2/CCS |
Field of application | Scenario 80 by 2050 |
3 H2/CCS gas infrastructure options |
Time horizon | 2050 | 2035 |
Type of analysis | Techno-economic | Socio-technical |
Criteria | Cost-effectiveness | Political feasibility |
Method | CBA-based optimisation, energy system modelling | Qualitative scenario development |
Focus | Policy identification | Policy implementation |
Approach | Forecast, mainly quantitative | Foresight, mainly qualitative |
Sectors | Gas, electricity, mobility, industry, building | Gas, electricity, mobility, heating, industry, politics |
Context | National (Germany), international | |
Results | 2 robust transformation pathways, 8 recommendations | 6 socio-technical scenarios as a policy evaluation framework |
Level of detail | Regional, concrete | National, abstract |
Robinius et al. [9] conclude that both scenarios are technically and economically feasible. They provide policy advice in form of eight core results and related recommendations, which build the core of the transformation strategy for both scenarios. For the scenario 80 and the scenario 95, the transformation strategies have two phases. An expansion of the energy system and renewable energies is needed, which is due to increased energy demand resulting from massive electrification (core result 2). In the first phase, immediate measures support the installation of new wind power and photovoltaic plant by 2035 (core result 3). An improvement of energy efficiency in all sectors should take place in parallel (core result 4). In the next phase, fossil-based technologies used in the industry for transport and construction need to be converted to bioenergy or electrification. Furthermore, hydrogen became a key energy carrier. Due to extensive incentive programs, heat pumps become the main heating technology (core result 5). New mobility concepts will promote a shift towards more rail transportation, but also increase hydrogen and electro mobility (core result 8). Consequently, hydrogen demand is expected to increase significantly, which requires new hydrogen production and infrastructure solutions as well as business models (core result 6). An extension of the underground hydrogen storage secures the energy supply. Energy imports are expected to decrease significantly, while the international energy markets will also trade hydrogen and synthetic fuels (core result 7). The scenarios' strategies share similarity but also differ. In the scenario 95, hydrogen demand is expected to increase to 12 Mio. tons in 2050. Domestic production satisfies over 50% of the demand, while energy imports are necessary [9].
The two cost-effective CO2 reduction strategies show major similarities with scenario 2 and scenario 3. Whereas scenario 80 is comparable to the technology-open scenario 2, scenario 95 is similar to scenario 3.
CBA-based approaches have several limitations. The paper's first point of critique refers to the policy assessment, i.e., the identification of the costs and the benefits, which is problematic. It is hardly possible to determine the correct monetary value for all economic costs and benefits. Besides the question what costs and benefits should be considered, monetarization is difficult. This is due to the fact that benefits occur in the future, which is characterized by uncertainty, making it hardly possible to predict upcoming costs and benefits. Especially in complex contexts like climate change, neoclassical models show flaws in coping with non-linear dynamics and incremental change over time. Such models assume rational agents and define the economy as a closed system. There is the false belief that economic systems are predictable in a similar way as mechanical ones.
In the study of Robinius et al. [9], measures for reducing CO2 emissions lead to savings of fossil energy carriers. The resulting reduction of total energy costs represents the benefits. To achieve the climate goals, investments in energy infrastructure, renewable energy production and energy efficiency are necessary, and display the costs. The expected total investment costs are substantially higher for the scenario 95 (192 billion EUR per year) compared to scenario 80 (102 billion EUR per year). The expected saved energy costs are lower in the scenario 80 (49 billion EUR) than in the scenario 95 (128 billion EUR). Robinius et al. [9] conclude that both scenarios are technically and economically feasible.
This example again illustrated how much the result depends on the value of costs and benefits that are compared. What costs and benefits are considered determines the results. In the same vein, Robinius et al. [9] emphasise that the saved energy costs strongly depend on the future development of energy costs. If those change, the results change as well. Furthermore, the study is mainly based on economic aspects. The result would be different if other costs and benefits are included. If achieving the climate goals leads to no other benefits than energy costs savings is questionable. An expansion of renewable energies can, for example, decrease dependencies on fossil fuels or results in protest and environmental damages. Especially non-economic health and environmental benefits are related to massive emission reduction, which is the main reason for emission reduction. The choice of the costs and benefits, though, implies an indirect normative statement. To not include an economic or non-economic aspect in the CBA indicates that the aspect is either not important or that its value equals zero. Considering that most expected costs refer to environmental damage or loss of lives associated with climate change, it is questionable why Robinius et al. [9] do not include non-economic aspects. However, when monetizing non-economic aspects, the problem of pricing the priceless' [56] and problem of incommensurability' arises [57]**. Additionally, expected future values need to be adjusted to the present, because costs and benefits often occur at different times. The controversially questions of the adequate' discount rate arises. It does also apply for the benefits of energy cost savings that according to the study occur in the future. Discounting is especially relevant for climate change mitigation, where benefits relate to the future, i.e., future generations [58]. Consequently, setting a discount rate has a normative dimension and should be discussed openly and carefully. In the case of Robinius et al. [9], no information about the discount rate is available.
The result of a CBA strongly depends on the considered costs and benefits and the accuracy of future estimates. If different costs and benefits are considered or the expected future development does not occur, the result might change. The CBA of Robinius et al. [9] is thus problematic in different aspects. To change one value might imply that the proposed pathway might become economical unfeasible. Even when assuming that the costs and benefits are complete and the adequate discount rate is known, the question about how the future might develop remains. This is especially true for the energy price and related future savings. Consequently, the proposed optimal pathway might become economical unfeasible.
Second, even if it would be theoretically possible to determine the correct value for all economic and non-economic costs and benefits, the question of policy choice arises. It neither implies that the researcher's advice will be chosen by decision-makers nor that it will be successfully implemented. CBA approach such as the analysis of Robinius et al. [9] stay within a narrow context and focus on a discipline specific perspective. The implementation side and related aspects remain untouched. This paper's different socio-technical scenarios show that it is possible to acknowledge a wide range of aspects and developments. In contrast, the discussion of Robinius et al. [9] remains within only two of these scenarios, namely the two best-case options. The other possible developments require individual optimal strategies each. To say it differently, in all other cases, which are represented exemplarily by the six scenarios in this paper, the identified optimal transition pathway will most likely not be pursued. If policy advice is solely derived from CBA thinking, it is one-sided and of little relevance for decision-makers.
From a complexity economics perspective, socio-economic systems are complex adaptive systems that can be described as a network of heterogenous agents. These agents adjust their behaviour according to the interaction with other agents. In complex adaptive systems, it is hardly possible to implement optimal policies through central planning. There is the risk that the decision made by a central planner based on a CBA, will be interrupted due to protest by negatively affected stakeholder groups [51]. This is due to one-sided perspective which neglects political feasibility. Aspects of implementation are neglected, which lead to open questions for decision-makers.
Contrary to the paper's scenarios, Rubinius et al. [9] mainly leave political developments out. Except for the nuclear phase-out and the coal phase-out, the political perspective remains untouched. Similarly, other non-economic and non-technical factors, such as issues of acceptance, are not covered or only mentioned as a side remark. A lot of open questions remain for decision-makers needed to choose a strategy. Conventional approaches hardly provide answers and mainly focus on economic and technical aspects.
Their results show, for example, that the strategies to realise each scenario differ considerably. Measures needed to achieve an 80% target are not necessary required for the scenario 95%. Some measures are even counterproductive and not compatible with the other scenario. Consequently, it is not clear for decision-makers which policy they should imply. For them, the question of which policy is more realistic to be successfully implemented is important. These kinds of questions are not considered in the study's recommendation. After deciding on one strategy, policy-makers want to know about fostering and hindering factors for a successful implementation. In both scenarios, a significant increase of wind power and photovoltaics is seen as the backbone of the future energy system. Since the success of the Energy Transition mainly depends on the related energy system expansion, information about main drivers is crucial. Especially stakeholder acceptance is essential for an energy system expansion. Robinius et al. [9] point to possible acceptance issues for onshore wind energy. Instead of thinking about options how to avoid conflicts, offshore wind plants are recommended as an alternative, which are way more expensive. Despite these considerations, acceptance issues play no role. How to deal with changing conditions and future developments is also not part of the analysis but important for policy implementation.
The third point of critique refers to the policy advice of conventional approaches which is tailored to fit to one specific future development. If the future that is expected to be the most likely, does not occur, the policy advice is hardly useful. It shows little robustness to changing conditions which represents a major limitation.
The transformation strategies for scenario 95 and scenario 80 are fragile and little robust to changing conditions. Referring to key measures, expected future development and unexpected extremes demonstrate this point of critique. The failure of the strategies' key pillar can risk or stop the whole transformation strategy as the following example highlights. In the first phase of the strategy implementation, construction projects are essential. The installation of new wind and photovoltaic plants and related infrastructure expansion for renewables (energy network) and hydrogen (gas network) are required. Related construction work builds the prerequisite for many other strategies' components. Without this critical infrastructure, a broad electrification and use of hydrogen applications is hardly possible. However, especially those infrastructure and energy plant projects can be stopped or delayed due to protests. Public acceptance issues or regulatory issues might arise and hinder the project's implementation. If one of the first phase's key measures cannot be realised, the second phase might not even start. Thus, an early strategy failure is possible. Other critical elements such as the supply of hydrogen or investments in hydrogen applications might have similar effects.
The scenario specific strategies are designed to fit a specific future development and climate goal. Therefore, the success and usefulness of the measures depends on the future developments. Although different future developments are possible, the study of Robinius et al. [9] neglect these. Most assumptions about background conditions such as population growth or mobility demand are taken from Gerbert et al. [59]. Exemplary, a discussion of three common assumptions of scenario 80 and scenario 95 shows alternative developments. The study expects the development of an international energy market for hydrogen and synthetic fuel. A fully functioning free market is assumed. To establish such a market, new legal regulation and a cross-border hydrogen infrastructure are needed but do not yet exist. When building such an infrastructure, problems of acceptance might also play a role. Additionally, agreements on how to share the investment costs between the producing and importing countries might lead to a delay in time. Also, existing long-term contracts on fossil fuels export and import might hinder hydrogen trade. Therefore, it might be possible that Germany's demand for hydrogen imports cannot fully be satisfied. Furthermore, the household size is expected to decrease further, while people will live in bigger places. This need for more comfort points to a rather unsustainable lifestyle and contradicts the future shift to more public transport and low-carbon mobility. People's lifestyle can change both in positive and negative terms, which has a major influence on the success of low-carbon application and energy and thus the transformation strategies.
Unexpected extreme events, such as the current Corona crisis, might change the future development as well. An impending recession resulting from Germany's partly economic and societal lockdown, might lead to a recession or lower economic growth. Lower economic growth has a negative effect on the investment environment. The Corona crisis might also shift the Government's focus from climate goal realisation towards the economy's recovery. Subsidies that would be needed to accelerate a deep decarbonisation might be cut due to unexpected spending during and after the crisis. In the energy sector, companies need to recover from economic losses and might postpone investments in infrastructure and renewable energy projects. Similarly, households might postpone planned investments in low-carbon heating or electro and hydrogen vehicles. The avoidance of public transport during the crisis might continue after the lockdown and lead to a preference of individual over public mobility. Due to European and international border restrictions, a new focus on national issues hinders the development of a free hydrogen market. Thus, a slowdown of the low-carbon transformation is possible. Extreme events associated with level 4 uncertainties are not predicable and could also have positive effects on the low-carbon transformation. The Corona crisis shows how a single event can totally change the expected future and make policies designed for a specific situation useless.
This section shows how qualitative scenario development overcomes limitations of the conventional CBA-based approach. Due to a different way how to cope with uncertainty and complexity, the first and third limitations does not apply to scenario development. The section's focus is thus on how approach addresses the second limitations, namely open questions.
Conventional approaches have a common structure. An optimal pathway, which is based on a given starting point and directed towards given targets is identified. It consists of three elements that can be formalised for two different targets as follows:
Open questions can be related to the different elements and demonstrate the missing focus on policy implementation:
B I) Which policy, i.e., pathway, will be chosen (B1 or B2)?
Which policy should be implemented?
Which policy is more realistic to be successfully implemented?
B II) What can decision-makers do to enable a successful implementation?
Which factors foster or hinder a successful implementation?
How can conflicts be avoided and how to foster supportive factors?
A) What if the starting point, i.e., the assumptions on background conditions, change?
What can decision-makers do to enable the materialisation of assumptions?
What are alternative developments and what do they imply for initial policy?
C) What if the given targets change?
Can the policy still be implemented, or does it need to be adapted?
What are consequences of applying the optimal policy to a different future?
Qualitative scenario development covers these questions in different ways. The question B I basically deals with the policy decision-making. From a practical perspective and considering the urgency of climate change, the question of what policy should be implemented heavily depends on what policy is most realistic to be successfully implemented. To identify realistic policies, political feasibility as a suitable criterion to evaluate different policies. Figure 5 displays the concept of political feasibility that the authors developed for this purpose. It is based on the conceptual framework of the energy scenario analysis by Schubert et al. [15]. They argue that political and social feasibility matters in energy scenarios and hence follows a holistic approach.
Analysis framework to identify feasibly policies (authors' own contribution)
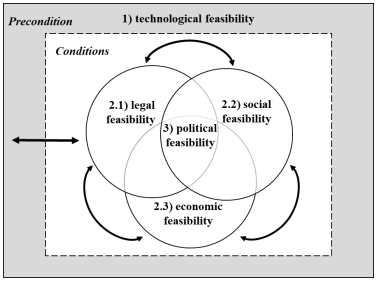
For this paper, the idea of identifying feasible scenarios is adapted and applied to the context of feasible policies. Technological feasibility' represents the first level, namely the necessary precondition for a feasible energy policy. If a measure is technologically not feasible, it cannot be implemented as an energy policy. Whether or not an energy policy is politically feasible exceeds, though, the technical dimension. This is why, the concept of political feasibility also includes legal feasibility', social feasibility' and economic feasibility' (level 2). While these level 2 conditions influence each other, there are also interdependencies between level 1 and level 2. Political will or economic incentives can support technological progress and enable technically feasible solutions.
The authors consider policies to be politically feasible if they have a high chance of being successfully implemented. Accordingly, two steps of feasible policies can be defined referring to a successful implementation: political process of decision-making, and political process of implementation. To initiate the process of policy implementation, political policy decision-making represents the first step. Political intention in the form of regulatory and legislative efforts for deep decarbonisation, i.e., through an energy system transformation, is key. In addition to the political will, political enforceability is required in order to find political majorities for the respective policy and re-design of laws. In a second step, the political process of policy implementation follows. To successfully implement the chosen policy, governmental resources, e.g., financial and personnel capacities, are required. When it comes to the final implementation of a policy, e.g., the building process of pipelines, governmental enforceability is important. Resisting veto-players who try to interrupt or stop the implementation is a main challenge.
Hence, qualitative scenario development addresses the question of which policy will be chosen (B I) by incorporating the reality of policy decision-making into scientific policy evaluation. This proposes a new, more holistic criterion for policy evaluation, i.e., an analysis framework for feasible policy recommendations that transcend discipline-specific criteria.
As the above understanding of political feasibility implies, knowing and addressing possible hurdles is essential for a successful implementation. Question B II is related to the process of policy implementation. Qualitative scenario development addresses type B II questions by identifying factors that foster or hinder a successful implementation in the form of key factors. These factors are not limited to but include all levels of political feasibility which exceeds the conventional techno-economic focus. For the case study example, 23 key factors were identified. Table 4 lists the 23 key factors and displays them in six categories to identify main drivers.
Key factors and categories for a gas infrastructure modification
Categories | Key factors |
---|---|
Outcomes | Realisation of national climate goals |
Stakeholders | Private investors in gas sector |
Measures | Phase out & phase in: fossil & renewable gas |
Sector-specific developments | Fuel of road traffic |
Infrastructure developments | Electricity network expansion |
Energy-related developments | Electricity production |
Decision-makers can use such a list of key factors as a base for identifying measures to avoid conflicts and strengthen supportive factors. There are shared key factors with Robinius et al. [9] such as energy prices' (electricity and gas), lignite energy phase out', electricity network expansion' or fuel of road traffic'. Despite these similarities, the list of key factors also comprises additional aspects such as political activities like governmental support for transformation technologies' or the phase out of fossil-based gas/phase in of renewable gas', which share the level of planning certainty.
Contrary to the techno-economic study, the socio-technical scenarios entail stakeholders as important key factors. Stakeholders represent an own category and comprise 5 key factors which refer to the following stakeholder groups:
Political decision-makers;
Citizens & society;
Public interest groups;
Economic lobby groups;
Investors in gas sectors (see Table 5).
Stakeholders and key factors
Stakeholder groups | Key factors |
---|---|
Political decision-makers | Character of public policy |
Citizens & society | Behaviour and public acceptance |
Public interest groups | Power of public interest groups |
Economic lobby groups | Power of lobbyism |
Investors in gas sector | Private investors in gas sector |
The evaluation of each initial influence factor according to their interconnection with the whole system functions as a verification or a falsification of the initial literature-based intuition. Accordingly, the key factor technological progress & market maturity' was identified as a systemic indicator. It implies that this factor displays changes of the whole system but has only little influence on the whole system itself (low activity, high passivity). This interpretation is consistent with the understanding of political feasibility that concludes technical feasibility on the lowest level as a necessary precondition. In this sense, scenario development enables decision-makers to identify crucial factors for a successful policy implementation that are neglected in conventional approaches, such as relevant stakeholders or hidden factors with systemic relevance.
These key factors represent a central element of qualitative scenario development and address type A questions, which focus on consequences of changing assumptions. Contrary to forecasting of key aspects as Robinius et al. [9] mostly do, scenario development aims to foresight all possible developments without assigning probabilities. Alternative pathways of development are expressed in the form of future projections. To adequately deal with a high level of uncertainty, scenario development allows to identify alternative developments in the form of consistent scenarios. The six socio-economic scenarios presented before can be used as an evaluation framework related to C I questions. In the case of a high level of uncertainty, the conventional way of defining first best policy options is highly risky. The better a policy is tailored to fit an expected future setting, the more difficult the implementation will be when this future does not materialize. The higher the level of complexity and uncertainty, the higher the chance that the future will develop differently than forecasted. In other words, it implies that the policy was designed to fit the wrong future, which might have severe consequences. Qualitative scenario analysis allows to address these issues in two ways:
First, the robustness of a specific policy can be analysed in the context of different scenarios. Different policies, in the paper's case infrastructure options, can be evaluated in a next step, which exceeds the scope of this paper. Each infrastructure option can be tested according to its compatibility with different scenarios. An option that does not fit the reality of a specific scenario, cannot be a useful policy recommendation. Consequences, acceptance problems or costs, that are related to implementing the optimal policy to a wrong' future can be analysed. A new understanding of robust and feasible policies is related to such an assessment;
Second, scenario analysis is a useful method to determine robust policies that fit all possible scenarios in a minimum way. In the sense of a lowest common denominator, such policies would decrease the risk of stranded assets and unprofitable investment while increasing the chances of a successful implementation. This does also mean that in the end, it might not be the first, second or even third best option that will be implemented. On the one hand, this might imply less-ambitious policy. On the other hand, it increases the chance of a successful implementation and might also set incentives to shape the overall setting by considering relevant stakeholders. Instead of identifying specific pathway, scenario development provides a framework to analyse policies that fit in a future space of possible windows of opportunities.
The aim of the paper was to show that foresight methods such as qualitative scenario development are a promising alternative to traditional CBA-based optimisation approach. This section summarises the added value of qualitative scenario development and discusses the methods' challenges and chances.
Referring to concrete examples, the result section demonstrated that qualitative scenario development is a promising alternative to conventional CBA-based forecasts. Table 6 distils the added value of qualitative scenario development in a more general way.
The value of qualitative scenario development
Limits CBA-based optimisation approaches | Advantages qualitative scenario analysis |
---|---|
Policy assessment | |
Quantification of costs and benefits: |
No quantification necessary: |
Policy choice | |
Technical, economic feasible policies: |
Political feasible policies: |
Policy advice | |
Policy advice fits a specific future pathway: |
Policy advice fits different future scenarios: |
The limitations of traditional methods are threefold. CBA-based forecast approaches revealed to be problematic (policy assessment), hardly relevant (policy choice) and of little robustness (policy advice) for an accelerated emission reduction. Identifying the adequate monetary value for economic and non-economic costs and benefits today and in the future ? is problematic. Policy advice based on economic and technological feasibility or cost-effectiveness is hardly relevant for decision makers. It neglects aspects of implementation and political feasibility and leaves open questions. Since the recommendations are based on strong assumptions and tailored to fit one specific future development, they are only little robust to changing conditions.
Qualitative scenario development overcomes these limitations in different ways. Contrary to CBA-based optimisation approaches, no quantification or monetarization is required to develop qualitative scenarios. Instead, main drivers and different future pathways are developed in qualitative terms. Focusing of policy implementation within the process of scenario development, allows to cover untouched aspects of techno-economic analyses. Open questions on changing conditions, fostering or hindering factors and policy choice are addressed using the following tools: key factor analysis, system-relevance analysis, consistency analysis, stakeholder identification and participation. Political feasibility is analysed through considering the core features of:
Complexity;
Non-economic aspects;
Uncertainty;
Stakeholders.
Policies are designed to be flexible and applicable to a variety of possible future scenarios. Advice is based on common patterns and drivers arising from different scenarios.
Although scenario development offers advantages over traditional quantitative methods, there are also challenges and criticism. Qualitative scenarios are criticised to be unrealistic since they rely on the limited expertise of few experts and stakeholders [60]. Therefore, results are based on the subjective understanding of participants and not on objective scientific analysis [61] and might hence be biased [62]. In the same vein, it is argued that the development of qualitative scenarios lacks reproducibility, which is crucial for scientific credibility. Results of scenario development, such as storylines, are criticised to be hardly reproducible because they are based on a dynamic group process [63]. Compared to quantitative models, assumptions used in scenario approaches are not expressed in formal equations but intensively discussed in qualitative terms. The lack of a formal expression nourishes the impression of missing transparency. It is, though, questionable if assumptions expressed in mathematical terms are more transparent than a detailed qualitative description of those.
Additionally, the value of qualitative scenario development is questioned due to a lack of quantitative data. Although the exactness of quantitative scenarios is misleading and provides a wrong impression of what can be known about the future, decision-makers still prefer numerical data over narratives. A (false) feeling of control and objectivity is associated with quantitative data. Against this background, qualitative scenarios have a hard standing as they do not satisfy the demand of decision-makers for quantitative information.
For researchers, qualitative scenario development is a rather unattractive method. The process of scenario development is very time-consuming, and the results are difficult to publish. For the core team, scenario development is very time intensive since repetition of process phases are necessary which requires a lot of internal consultation and meetings. Especially visualization and storytelling, for which economists are normally not training, is often underestimated. Extra training can be required, also to design participatory elements and workshops. Time-intensive participation can be a constraining factor for external participating stakeholders. To keep the workshop or interview time as brief as possible, without risking leaving to little time for reflections requires experience and planning. Publishing research resulting from uncommon techniques, such as visualisation or storytelling, is difficult. This kind of research hardly fits the tradition of economic publication, which represents according to Kapeller [64] an obstacle for researchers.
Scientists who are using qualitative scenario development are aware of these challenges. They established different criteria for evaluating the quality of their work. For selecting scenarios in the last phase of the process, the literature proposes different criteria as guidance. Wilson [65] defines five criteria, according to which scenarios should be:
Plausible;
Different to each other;
Consistent;
Useful for decision-making;
A challenging mindset about the future.
Others add compatibility, stability and variability. There is also research on the improvement of stakeholder participation. Ernst et al. [7], for example, discusses on how to improve the design and conduct of different participatory method and on how to overcome related biases.
These challenges and critique can be pinned down to questions of philosophy of science. The point of critique reveals that different and divergent understandings of what counts as good' science are present. The general discussion about objectivity in science is also reflected in the critique towards scenario analyses and especially applies to difficulties associated with stakeholder participation. Whether qualitative methods generate valuable knowledge is related to the question of true knowledge in the tradition of the epistemic goal of science, which was a long tradition. Whether aspects of implementation should be considered by scientists or left to decision-makers is related to the scientists' understanding of the role of science in society.
Qualitative scenario analysis and foresight methods in general have the potential to contribute to the further development of the science of economics. Confronted with critique due to forecast failure in the last years, a shift within the science of economics towards more foresight might help to restore the profession's reputation [5]. Openness for new foresight approaches could raise the awareness for limits of forecasting-based policy advice. Applying foresight methods contributes to methodological plurality and pluralism in economics, which is criticized to be missing in mainstream economics [66]. Foresight methods could thus stimulate economists to enlarge their perspective. It is helpful for overcoming the "failure of collective imagination" of economists, which is seen the reason for not seeing the financial crisis coming [67]. In contrast to forecasting, foresight promises less in term of predictability. It is a step towards more humility in economics, which was long argued to be a missing character trait of economists [68].
Nonetheless, this paper does not argue that economists should refrain from forecasting. In general, these methods are valuable but have their limitations. The decision between forecasting and foresight is no question of either-or. Both methods have their legitimacy for specific purposes but also boundaries. For guiding decision-making, foresight methods should be acknowledged as promising answers to limitations of quantitative forecasting. Similarly, qualitative scenario development is no strict decision with qualitative and quantitative research. It can be useful to quantify some aspects for the interpretation of the scenarios, e.g., hydrogen mobility. Complementing quantification might help to get a better understanding of the possible development or satisfies decision-makers' demand for data. Insights of scenario development could also be used as a modelling foundation, such as agent-based modelling.
Facing a high level of uncertainty and complexity, it has to be elaborated with care what aspects can be quantified. To combine qualitative and quantitative approaches can also yield to new knowledge that would not have been accessible with a single method's approach. McDowall [69] applies a hybrid approach to explore transition pathways for hydrogen energy. His work on "bringing narrative socio-technical storylines into dialogue' with quantitative energy systems modelling" underlines the potential of methodological improving by applying new methods.
This paper argues that economic energy policy research can contribute more to the acceleration of deep decarbonisation by emphasizing the implementation side of policies. It implies to identify feasible instead of optimal polices and requires including four core features:
Complexity;
Non-economic aspects;
Uncertainty;
Stakeholders.
Furthermore, it argues that optimal policy recommendations based on traditional economic optimisation analyses and forecasts are optimal only from a narrow perspective of economic theory. Thus, they have little chances of being successfully implemented and can be misleading for two reasons: they are tailored to fit a future that was expected as the most likely development but did not occur, and they face unexpected political and societal opposition and do not find political majorities. While the former is related to complexity and fundamentally uncertainty of social systems which makes economic mid- and long-term forecasts quite unreliable, the latter relates to the neglect of non-economic aspects in economic policy analyses. The authors argue that complexity and fundamental uncertainty of energy systems are serious challenges for traditional policy analysis that call for a rethinking of economic energy policy analyses.
The example of the paper's case study showed that qualitative scenario analysis is a promising alternative to conventional approaches. It allows to include the four core features and addresses limitations of the traditional optimisation approach. A comparison with a CBA-based optimisation study that identifies techno-economic pathways for given climate targets revealed limits of traditional approaches. It leaves at least three types of open questions, namely on the implications of wrong forecasts (A), concerning policy choice (B) and changing climate targets (C). By developing socio-technical scenarios for an assessment of gas infrastructure strategies, it was demonstrated that scenario analysis addresses these questions in different ways. First, it incorporates the reality of policy-choice into scientific analysis by proposing political feasibility as an evaluation framework to identify feasible policies. Second, scenario analysis acknowledge uncertainty related to the future development in the sense of allowing key factors to develop in different pathways that result in consistent and plausible scenarios. Third, the key factor analysis helped to identify hurdles of implementation and underlined the importance of stakeholder dynamics for the success of the policy implementation. The six qualitative scenarios suggest that major investments in the German gas infrastructure and more general in an energy system transformation are more likely in some scenarios than in others. Finally, feasible and robust policies can be developed based on these scenarios, which is beyond the scope of the methodological paper and implies a need for more research.
Adapting to uncertainty and accepting related unpredictability in the context of decarbonising, the energy sector does not have to be a disadvantage for decision-makers and scientists providing policy advice. Implementing robust and feasible policies that fit different scenarios of future development, allows decision-makers to benefit from windows of opportunities. Furthermore, they avoid being locked in measures that are only optimal in a single situation but not in others. In the field of energy policy research, scientists can provide plausible scenarios for the development of energy systems and related conditions to support decision-makers. Despite the unpredictability related to social systems, scientists can make a valuable contribution to understand transitions better and thus accelerate deep decarbonisation.
Sociotechnical Transitions for Deep Decarbonization ,Science , Vol. 357 (6357),pp 1242-1244 , 2017, https://doi.org/https://doi.org/10.1126/science.aao3760
, Energy Futures: Diversity and the Need for Assessment ,Futures , Vol. 43 (8),pp 820-830 , 2011, https://doi.org/https://doi.org/10.1016/j.futures.2011.05.024
, More Foresight, Less Forecasting in Macroeconomics ,Working Paper Series ,pp 1-21 , 2019
, - , , Embracing Complexity: Strategic Perspectives for an Age of Turbulence, 2015
- , , Complexity and the Economy, 2015
Long-Term Impacts of a Coal Phase-Out in Germany as Part of a Greenhouse Gas Mitigation Strategy ,Applied Energy , Vol. 192 ,pp 234-246 , 2017, https://doi.org/https://doi.org/10.1016/j.apenergy.2017.01.065
, Benefits and Challenges of Participatory Methods in Qualitative Energy Scenario Development ,Technological Forecasting and Social Change , Vol. 127 ,pp 245-257 , 2018, https://doi.org/https://doi.org/10.1016/j.techfore.2017.09.026
, Shared Socio-Economic Pathways of the Energy Sector Quantifying the Narratives ,Global Environmental Change , Vol. 42 ,pp 316-330 , 2017, https://doi.org/https://doi.org/10.1016/j.gloenvcha.2016.07.006
, - , , Paths for the Energy Transition, Cost-Efficient and Climate-Friendly Transformation Strategies for the German Energy System Up to the Year 2050 (in German), 2019
A Review of Scenario Planning ,Futures , Vol. 46 ,pp 23-40 , 2013, https://doi.org/https://doi.org/10.1016/j.futures.2012.10.003
, The Roads Ahead: Narratives for Shared Socioeconomic Pathways Describing World Futures in the 21st Century ,Global Environmental Change , Vol. 42 ,pp 169-180 , 2017, https://doi.org/https://doi.org/10.1016/j.gloenvcha.2015.01.004
, Context Scenarios and Their Usage for the Construction of Socio-Technical Energy Scenarios ,Energy , Vol. 111 ,pp 956-970 , 2016, https://doi.org/https://doi.org/10.1016/j.energy.2016.05.073
, Socio-Technical Scenarios as a Methodological Tool to Explore Social and Political Feasibility in Low-Carbon Transitions: Bridging Computer Models and the Multi-Level Perspective in UK Electricity Generation (2010-2050) ,Technological Forecasting and Social Change , Vol. 151 ,pp 119258 , 2018, https://doi.org/https://doi.org/10.1016/j.techfore.2018.04.001
, Integrating Techno-Economic, Socio-Technical and Political Perspectives on National Energy Transitions: A Meta-Theoretical Framework ,Energy Research & Social Science , Vol. 37 ,pp 175-190 , 2018, https://doi.org/https://doi.org/10.1016/j.erss.2017.09.015
, Does Political and Social Feasibility Matter in Energy Scenarios? ,Energy Research & Social Science , Vol. 7 ,pp 43-54 , 2015, https://doi.org/https://doi.org/10.1016/j.erss.2015.03.003
, Typology of Sociotechnical Transition Pathways ,Research Policy , Vol. 36 (3),pp 399-417 , 2007, https://doi.org/https://doi.org/10.1016/j.respol.2007.01.003
, Studying Transitions: Past, Present, and Future ,Research Policy , Vol. 48 (9),pp 1-16 , 2019, https://doi.org/https://doi.org/10.1016/j.respol.2019.04.012
, Engaging With the Politics of Sustainability Transitions ,Environmental Innovation and Societal Transitions , Vol. 1 (1),pp 70-75 , 2011, https://doi.org/https://doi.org/10.1016/j.eist.2011.02.003
, The Three Domains Structure of Energy-climate Transitions ,Technological Forecasting and Social Change , Vol. 98 ,pp 290-302 , 2015, https://doi.org/https://doi.org/10.1016/j.techfore.2015.05.009
, An Agenda for Sustainability Transitions Research: State of the Art and Future Directions ,Environmental Innovation and Societal Transitions , Vol. 31 ,pp 1-32 , 2019, https://doi.org/https://doi.org/10.1016/j.eist.2019.01.004
, - , , Allgemeine Wirtschaftspolitik, 3., Überarb. und Erw. Aufl., 2009
Improving the Way We Think About Projecting Future Energy Use and Emissions of Carbon Dioxide ,Climatic Change , Vol. 90 (3),pp 189-215 , 2008, https://doi.org/https://doi.org/10.1007/s10584-008-9458-1
, The Current State of Scenario Development: An Overview of Techniques ,Foresight , Vol. 9 (1),pp 5-25 , 2007, https://doi.org/https://doi.org/10.1108/14636680710727516
, The Origins and Evolution of Scenario Techniques in Long Range Business Planning ,Futures , Vol. 37 (8),pp 795-812 , 2005, https://doi.org/https://doi.org/10.1016/j.futures.2005.01.003
, Scenario Management: An Approach to Develop Future Potentials ,Technological Forecasting and Social Change , Vol. 59 (2),pp 111-130 , 1998, https://doi.org/https://doi.org/10.1016/S0040-1625(97)00166-2
, - , , Methods of Future and Scenario Analysis, Overview, Assessment, and Selection Criteria, DIE Re-search Project Development Policy: Questions for the Future, 2008
- , , Technikfolgenabschätzung ‒ Eine Einführung, 2002
- , , Scenarios, An Explorers Guide, Exploring the Future, 2003
Scenario Techniques for Energy and Environmental Research: An Overview of Recent Developments to Broaden the Capacity to Deal With Complexity and Uncertainty ,Environmental Modelling & Software , Vol. 97 ,pp 201-210 , 2017, https://doi.org/https://doi.org/10.1016/j.envsoft.2017.07.017
, - , , Cloudy Crystal Balls, An Assessment of Recent European and Global Scenario Studies and Models, Experts Corner Report, Prospects and Scenarios No. 4, 2000
- , , Szenariotechnik: Vom Umgang mit der Zukunft, 2006
- , , Thinking in Alternative Futures (in German), Internationale Politik, No.12/2006-Zukunftsfragen, 2006
, European Foundation for the Improvement of Living and Working Conditions , 2003
, Scenario Types and Techniques: Towards a Users Guide ,Futures , Vol. 38 (7),pp 723-739 , 2006, https://doi.org/https://doi.org/10.1016/j.futures.2005.12.002
, Scenario Planning: A Tool for Strategic Thinking ,Sloan Management Review , Vol. 36 (2),pp 25-40 , 1995
, Research Needs and Challenges Faced in Supporting Scenario Design in Sustainability Science: A Literature Review ,Sustainability Science , Vol. 11 (2),pp 331-347 , 2016, https://doi.org/https://doi.org/10.1007/s11625-015-0340-6
, , The Art of the Long View: Paths to Strategic Insight for Yourself and Your Company (Planning for the Future in an Uncertain World) , 1996
, , Szenario Techniques , 1988
, Energy Efficiency Road Mapping in Three Future Scenarios for Lao PDR ,J. Sustain. Dev. Energy Water Environ. Syst. , Vol. 1 (3),pp 172-186 , 2013, https://doi.org/https://doi.org/10.13044/j.sdewes.2013.01.0013
, Futures Under Glass: A Recipe for People Who Hate to Predict ,Futures , Vol. 22 (8),pp 820-842 , 1990, https://doi.org/https://doi.org/10.1016/0016-3287(90)90018-D
, , Scenarios: The Art of Strategic Conversation , 2005
, , German Case Study: Final Design and First Results, Project Report , 2019
, ELEGANCY: The Interdisciplinary Approach of the German Case Study to Enable a Low Carbon Economy by Hydrogen and CCS ,Energy Procedia , Vol. 158 ,pp 3709-3714 , 2019, https://doi.org/https://doi.org/10.1016/j.egypro.2019.01.887
, Complexity and the Economics of Climate Change: A Survey and a Look Forward ,Ecological Economics , Vol. 138 ,pp 252-265 , 2017, https://doi.org/https://doi.org/10.1016/j.ecolecon.2017.03.032
, Information Visualisation for Science and Policy: Engaging Users and Avoiding Bias ,Trends Ecol. Evol. (Amst) , Vol. 29 (3),pp 148-157 , 2014, https://doi.org/https://doi.org/10.1016/j.tree.2014.01.003
, Guidance for Transport Planning and Policymaking in the Face of an Uncertain Future ,Transportation Research Part A: Policy and Practice , Vol. 88 ,pp 104-116 , 2016, https://doi.org/https://doi.org/10.1016/j.tra.2016.03.012
, Addressing Deep Uncertainty Using Adaptive Policies: Introduction to Section 2 ,Technological Forecasting and Social Change , Vol. 77 (6),pp 917-923 , 2010, https://doi.org/https://doi.org/10.1016/j.techfore.2010.04.004
, Defining Uncertainty: A Conceptual Basis for Uncertainty Management in Model-Based Decision Support ,Integrated Assessment , Vol. 4 (1),pp 5-17 , 2003
, , Optimal Adaptive Policymaking Under Deep Uncertainty? Yes We Can! , 2012
, When and How to Use Scenario Planning: A Heuristic Approach With Illustration ,J. Forecast. , Vol. 10 (6),pp 549-564 , 1991, https://doi.org/https://doi.org/10.1002/for.3980100602
, Complexity Economics and its Implications for Economic Policy (in German) ,Perspektiven der Wirtschaftspolitik , Vol. 16 (4),pp 379-392 , 2015, https://doi.org/https://doi.org/10.1515/pwp-2015-0024
, Towards a Low-Carbon Society via Hydrogen and Carbon Capture and Storage: Social Acceptance from a Stakeholder Perspective ,J. Sustain. Dev. Energy Water Environ. Syst. , Vol. 9 (1),pp 1-16 , 2020
, A Rationale for Science-Policy Interfaces ,Futures , Vol. 39 (7),pp 807-826 , 2007, https://doi.org/https://doi.org/10.1016/j.futures.2006.12.004
, A Review of Socio-Technical Energy Transition (STET) Models ,Technological Forecasting and Social Change , Vol. 100 ,pp 290-305 , 2015, https://doi.org/https://doi.org/10.1016/j.techfore.2015.07.017
, Scenario Methodology: New Developments in Theory and Practice ,Technological Forecasting and Social Change , Vol. 80 (4),pp 561-565 , 2013, https://doi.org/https://doi.org/10.1016/j.techfore.2012.11.011
, - , , Critique of Cost-Benefit Analysis, and Alternative Approaches to Decision-Making, A Report to Friends of the Earth England, 2008
Cost-Benefit Analysis, Incommensurability and Rough Equality ,Environmental Values , Vol. 11 (1),pp 27-47 , 2002
, - , , Towards the Ethics of a Green Future: The Theory and Practice of Human Rights for Future People, 2018
- , , Climate Paths for Germany (in German), 2018
The Allure of Energy Visions: Are Some Visions Better Than Others? ,Energy Strategy Reviews , Vol. 2 (3-4),pp 211-219 , 2014, https://doi.org/https://doi.org/10.1016/j.esr.2013.10.001
, Bridging Analytical Approaches for Low-Carbon Transitions ,Nature Clim. Change , Vol. 6 (6),pp 576-583 , 2016, https://doi.org/https://doi.org/10.1038/nclimate2980
, Stakeholder Involvement in Sustainability ScienceA Critical View ,Energy Research & Social Science , Vol. 17 ,pp 71-81 , 2016, https://doi.org/https://doi.org/10.1016/j.erss.2016.04.001
, - , , Environmental Futures: The Practice of Environmental Scenario Analysis, 2008
Some Critical Notes on Citation Metrics and Heterodox Economics ,Review of Radical Political Economics , Vol. 42 (3),pp 330-337 , 2010, https://doi.org/https://doi.org/10.1177/0486613410377855
, - , , Learning from the Future: Competitive Foresight Scenario, 1998
- , , Climate Economics: A Call for More Pluralism and Responsibility, 2020
- , , The Global Financial Crisis Why Didnt Anybody Notice?, 2009
Macroeconomics After the Crisis: Time to Deal With the Pretense-Of-Knowledge Syndrome ,Journal of Economic Perspectives , Vol. 24 (4),pp 85-102 , 2010, https://doi.org/https://doi.org/10.1257/jep.24.4.85
, Exploring Possible Transition Pathways for Hydrogen Energy: A Hybrid Approach Using Socio-Technical Scenarios and Energy System Modelling ,Futures , Vol. 63 ,pp 1-14 , 2014, https://doi.org/https://doi.org/10.1016/j.futures.2014.07.004
,