Ammonium is produced by decomposing organic nitrogen compounds in anaerobic sludge stabilisation, commonly applied in waste water treatment plants > 20,000 population equivalents. Anaerobic digestion leads to the formation of ammonium (NH4+) as a result of ammonification of organic nitrogen. Due to its high solubility, the major proportion of ammonium is present in the liquid phase of the digested sludge, wherefore high ammonium contents are present in the effluents after mechanical sludge dewatering (sludge liquor [1]). Typical concentrations are in the range of 250-1,500 mg NH4+ L−1, sometimes even higher [2], [3] depending on the process parameters and whether co-substrates are fermented or not.
The further treatment of sludge liquor is usually done by returning the liquid phase to the aerobic nitrification of the waste water treatment plant (mainstream treatment). The recirculation can cause a nitrogen back-loading of up to 20%, depending on the amount of nitrogen in the waste water feed [4], [5], as well as increasing energy costs (e.g., for fan operation). Moreover, the design capacity for NH4+-removal of many sewage plants is currently approached and will further be exceeded [3]. The selective treatment of sludge liquor by additional alternative processes for ammonium removal (side-stream treatment) offers an opportunity to reduce nitrogen back loading and avoids the cost-intensive enlargements of the aeration basin. In addition to relieving the main flow, these side-stream processes usually require less space than the co-treatment of sludge liquor in the mainstream treatment [4].
Several biological processes for side-stream treatment of sludge liquor are currently applied (e.g., Sequencing Batch Reactor, Partial Nitrification, Anammox), but none of them facilitates a selective nitrogen recovery. Conventional stripping processes or the ANAStrip® process, which produce concentrated NH4+-containing solutions, were not installed extensively at waste water treatment plants due to low ammonium concentrations in the sludge liquor and the high operation and investment costs [6], [7]. In addition, operating problems such as pressure losses or even blockages can occur due to the fouling of bio-solids in the stripping columns [8]. Only 8 conventional ammonia strippers have been installed until 2005 in large-scale treatment plants in Europe for the side-stream treatment of sludge liquor [5]. To date, the ANAStrip® process was installed at two biogas plants [9].
Struvite precipitation is another possibility for nitrogen recovery from anaerobically treated effluents [10]. It is a chemical-physical nitrogen removal from sludge liquor in which ammonium is converted into an insoluble form and removed by a solids separation. With regard to an additional recovery of phosphorus, this process is currently gaining in importance, although it has not yet been able to establish struvite precipitation for nitrogen elimination [5].
Natural zeolites, in particular clinoptilolite, are also attractive for wastewater treatment. As a natural mineral, clinoptilolite is easily available, inexpensive and comprises a high selectivity for the absorption of ammonium from aqueous solutions [11], [12]. Wang and Peng [11] provided a good overview of the research activities carried out so far with respect to ammonium sorption on clinoptilolite following the mechanism of ion exchange. Furthermore, the ammonium exchange capacity could be increased if clinoptilolite was treated with sodium (Na+) in advance, since Na+ and NH4+ have a high affinity for mutual exchange [11], [13]-[15]. The exchange of Na+ for NH4+ can be written as follows (Z describes the exchange material):
(1)
In general, exchangeable cations are only weakly bound to the inorganic framework structure [16]. This means that exchangeable cations can be easily removed or exchanged when the zeolite is flushed with a highly concentrated solution of another cation. Additional, the concentration of the cation to be exchanged in the solution can influence the position of the equilibrium. During the regeneration of zeolites, the amount of exchanged sorbate can be increased by simultaneously removing the exchanged ion from the regeneration solution, in case of ammonium, e.g., by stripping [17].
Sherman [17] described a combined process for ammonium recovery from waste water with an ion exchanger (clinoptilolite) and simultaneous stripping of the regeneration solution with a subsequent acid scrubber. In the 1970s, two large-scale plants for the treatment of municipal waste water with nitrogen levels lower than 50 mg N L−1 were put into operation [12].
As well Deng et al. [18], [19] carried out ammonium separation experiments with zeolite and subsequent regeneration with a Sodium chloride (NaCl)-solution. The ammonium exchange capacity of the applied zeolite decreased with increasing regeneration frequency, treating NH4Cl-solutions with input concentrations of 30 mg N L−1 [19].
The Ion exchanger Loop Stripping (ILS) process is an option for simultaneous nitrogen recovery directly from sludge liquor. ILS is a hybrid process based on a combination of a solid ion exchanger material with simultaneous stripping of the regeneration solution [20]. Fixed bed columns of natural zeolite (main mineral: clinoptilolite) are used for ammonium ion exchange, which are alternately loaded and regenerated. The zeolite is regenerated with simultaneously air stripped sodium hydroxide (NaOH)-solutions. Stripped ammonia is captured in a scrubber with sulfuric acid to form a nitrogen oxide (NOx)-removal agent for off-gas cleaning in industrial processes. A basic flow chart of the ILS can be found in Ellersdorfer [20].
The advantage of simultaneous stripping with continuous circulation of the regeneration solution is a constantly low NH4+-concentration in the regeneration solution and a continuously high driving force for the ion exchange in accordance with the law of mass action. This leads to a significant reduction of the liquid volumes used for regeneration, without having to accept losses in the regeneration performance. Compact stripper and scrubber columns have a positive effect on the investment costs and the energy requirement for pumps and fan [21]. Additionally, the heating of the stripping solution is correspondingly low.
Lab scale experiments with the ILS process showed that ammonium recovery from ammonium sulfate [(NH4)2SO4]-solutions as well as real sludge liquor samples was possible. The results indicate the formation of a stable ion exchange equilibrium after several loading and regeneration cycles with a constant basic loading of the zeolite of around 3 mg NH4+ g−1 and ammonium removal rates between 44.7 and 60.2% [22].
In the present study, the results of pilot-scale experiments conducted on a real waste water treatment plant are presented. The special focus is on the zeolite stability, the technical feasibility of the ILS process and the achievable removal rates of ammonium after numerous loading and regeneration cycles. Experiments were performed with a mobile pilot plant at a municipal sewage plant in Austria using sludge liquor after mechanical dewatering.
Pilot scale experiments for the recovery of ammonium were done with a mobile plant (Figure 1), completely assembled in a 20‘ storage container, which can be operated at various locations.
Three ion exchanger columns (diameter ca. 450 mm) are the core units of the plant and can be operated individually or in series. Each column is filled with approximately 100 kg of ion exchange material (granular zeolite). The zeolite bed is placed between two sieve trays. The lower sieve tray rests on a perforated plate, which is attached at a height of 200 mm above the conical bottom of the column to ensure a homogenous liquid distribution in the column. Liquid flow through the column is from bottom to top to ensure a complete submersion of the whole ion exchanger material in the loading and regeneration solutions.
The plant is equipped with a stripper and a scrubber column for zeolite regeneration. Both columns are identical in construction and equipped with RPP SP 250 (RVT Process Equipment) packings, over a height of 1.2 m. The columns are mounted on sumps filled with regeneration and scrubbing solution. The regeneration solution is pumped from the stripper sump through one of the three ion exchanger columns back into the stripper via a separate piping system equipped with two heat exchangers and a continuous flow heater (max. 80 °C). The scrubbing solution is pumped from the sump to the head of the scrubber column. The plant has two other piping systems for flushing the ion exchanger columns with tab water and discharging the ion exchanger columns.
Interior view of the pilot plant for testing the ILS process (20’ container)
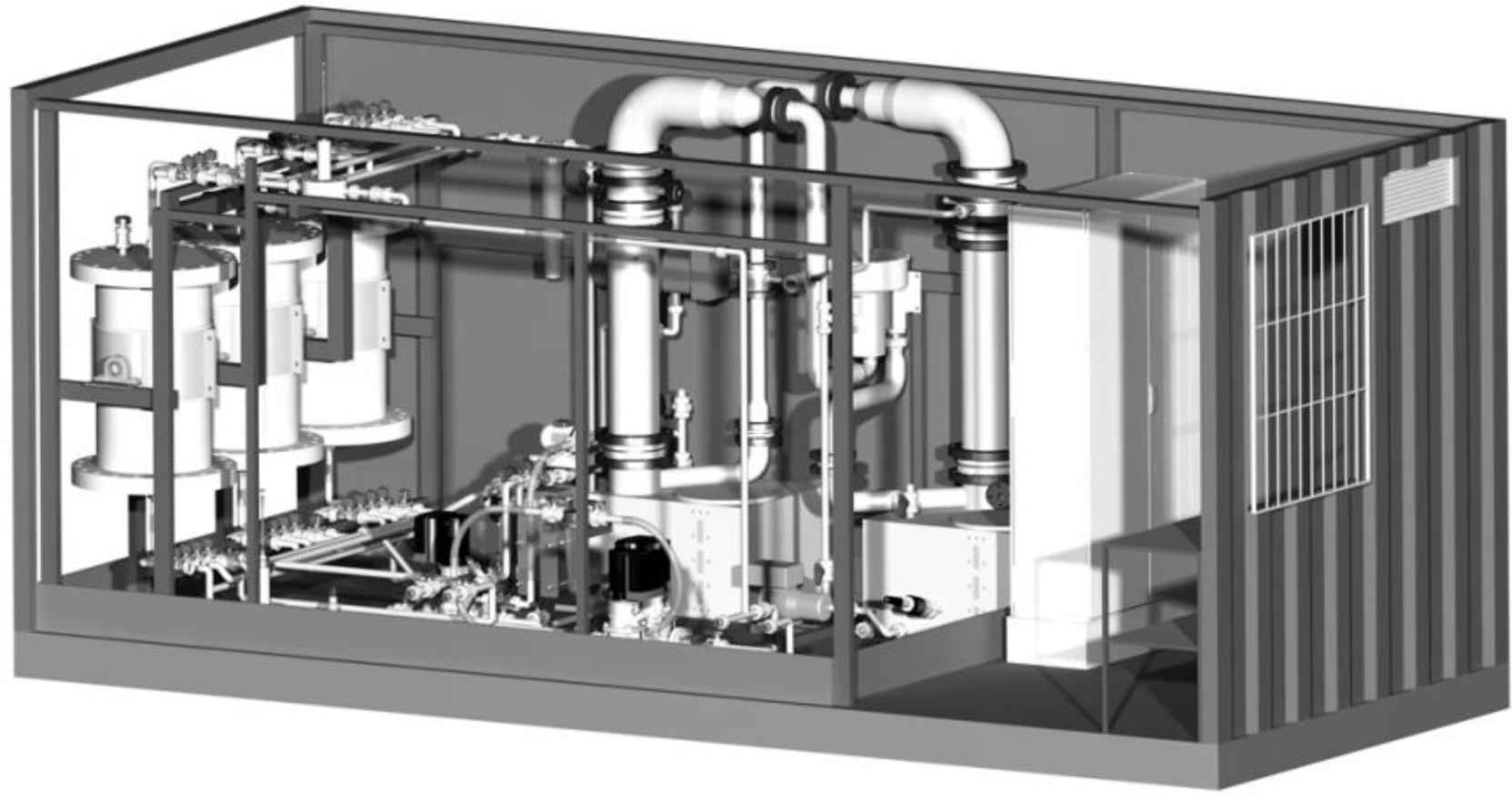
Natural zeolite (main mineral: clinoptilolite) with a particle size of 1-2.5 mm was used for the pilot-scale experiments. 100 kg of dry zeolite was manually washed with tap water to remove fines (approx. 1-2 m%). Finally, the washed zeolite was filled into one of the ion exchanger columns.
Ammonium loading experiments were performed using effluents directly discharged after the screw press of the municipal waste water treatment plant in Knittelfeld/Austria. The extracted sludge liquor was transferred into a separate receiver tank (capacity ca. 2 m³) outside the container. The columns were operated with sludge liquor by a centrifugal pump via the receiver tank, to ensure a continuous supply of sludge liquor with stable ammonium concentrations during the loading cycles.
Sludge liquor samples were taken at the beginning, at the end and after defined intervals during the loading experiments. The ammonium concentration of the samples was determined with a Photometer DR3900 (Hach Lange) and standard test kits LCK 302 and 303 for different concentration ranges. According to the manufacturer, the photometric accuracy is 1% with an absorbance of 0.5-2.0.
Loading cycles in all experiments lasted 60 minutes over one column (experiments E-1 to E-62) and 180 minutes over two columns in series (experiments A, B and C). Starting time was defined with the first overflow of treated solution on the top of the (first) ion exchanger column. After treatment, the sludge liquor was returned to the main sludge liquor flow of the waste water treatment plant.
After each loading cycle, the zeolite bed was flushed upstream with tap water to minimize carryover of sludge liquor residues into the regeneration solution. The regeneration was started after discharging the remaining washing solution from the buttom of the column.
Sodium hydroxide solution was pumped from bottom to top over the zeolite bed for regeneration. The solution was simultaneously stripped at a temperature of 60 °C. Sodium hydroxide solution (30%) was added to maintain pH-values > 11, depending on the measured pH-value in the stripping sump.
The circulated stripping gas was cleaned from ammonia in the subsequent scrubber with a H2SO4-solution (pH < 2) to produce a concentrated (NH4)2SO4-solution. The increase of the pH-value above 2 in the scrubbing solution was prevented by adding the sulphuric acid solution (50%), depending on the measured pH-value in the scrubber sump.
After regeneration, the remaining regeneration solution in the ion exchanger column was pumped back to the stripper sump. Then, the zeolite bed was again flushed upstream with tap water before the next loading cycle was started. Flushing and discharging of the zeolite bed was performed after each loading/regeneration cycle. The loading and regeneration conditions during the experiments are summarized in Table 1.
Loading and regeneration conditions during the experiments
|
E-1 to E-62 |
E-A, B, C |
---|---|---|
Number columns [-] |
1 |
2 in series |
Zeolite mass in column(s) [kg] |
98 |
196 |
Bed volume [m3] |
0.123 |
0.245 |
Flow rate [L h−1] |
500 |
|
Flow rate [BVa h−1] |
4.07 |
2.04 |
Loading |
||
Solution |
Sludge liquor |
|
L/Z-ratiob [mL g−1] |
5.1 |
2.6 |
Duration [min] |
60 |
180 |
Regeneration |
||
Solution |
NaOH |
|
pH [-] |
> 11 |
|
Temperature [°C] |
60 |
|
Total volume [L] |
150-200 |
|
L/Z-ratiob [mL g−1] |
1.5-2.0 |
0.8-1.0 |
Duration [min] |
90 |
120 (C1), 90 (C2) |
Bed volume
Ratio of the volumetric amount of loading/regeneration fluid of the mass of zeolite in the column
The NH4+-removal rates (A [%]), calculated with the trapeze integral of the difference between the initial and treated sludge liquor concentrations normalized to the initial concentration, are used for the analysis and evaluation of the loading cycles:
(2)
where A [%] is NH4+-removal rates over the experimental duration based on the initial sludge liquor concentration, c0,LOAD [mg NH4+ L−1] is initial NH4+-concentration of the sludge liquor, ci,LOAD [mg NH4+ L−1] is NH4+-concentration of the treated sludge liquor at defined times (i) and t is loading time.
The averaged NH4+-concentration of the sludge liquor at the beginning and at the end of the loading cycles was used for the calculation of the inlet NH4+-concentration. The NH4+-concentration of the treated sludge liquor was determined after 5, 10, 15, 30 and 60 minutes and, in the case of serial experiments, additionally after 120 and 180 minutes.
The results and discussion are divided into three sections. At the beginning, the first four loading cycles with fresh ion exchanger material are presented, followed by the results over 62 loading cycles. Finally, the results of a serial operation of two ion exchanger columns are presented.
The results of the first four loading cycles (E-1 to E-4) revealed a significant increase of the NH4+-removal rates after the first NaOH regeneration. A removal rate of 66.0% was achieved in the first loading cycle (E-1), which increased to average removal rates of 87.1 ±1.1% over the following three loading cycles (E-2 to E-4), when sludge liquor with 1,035 ±33 mg NH4+ L−1 was used under similar conditions (Figure 2).
Removal rates of ammonium of the first four loading cycles of the ion exchanger column (c0 = feed concentration of sludge liquor of the respective cycle)
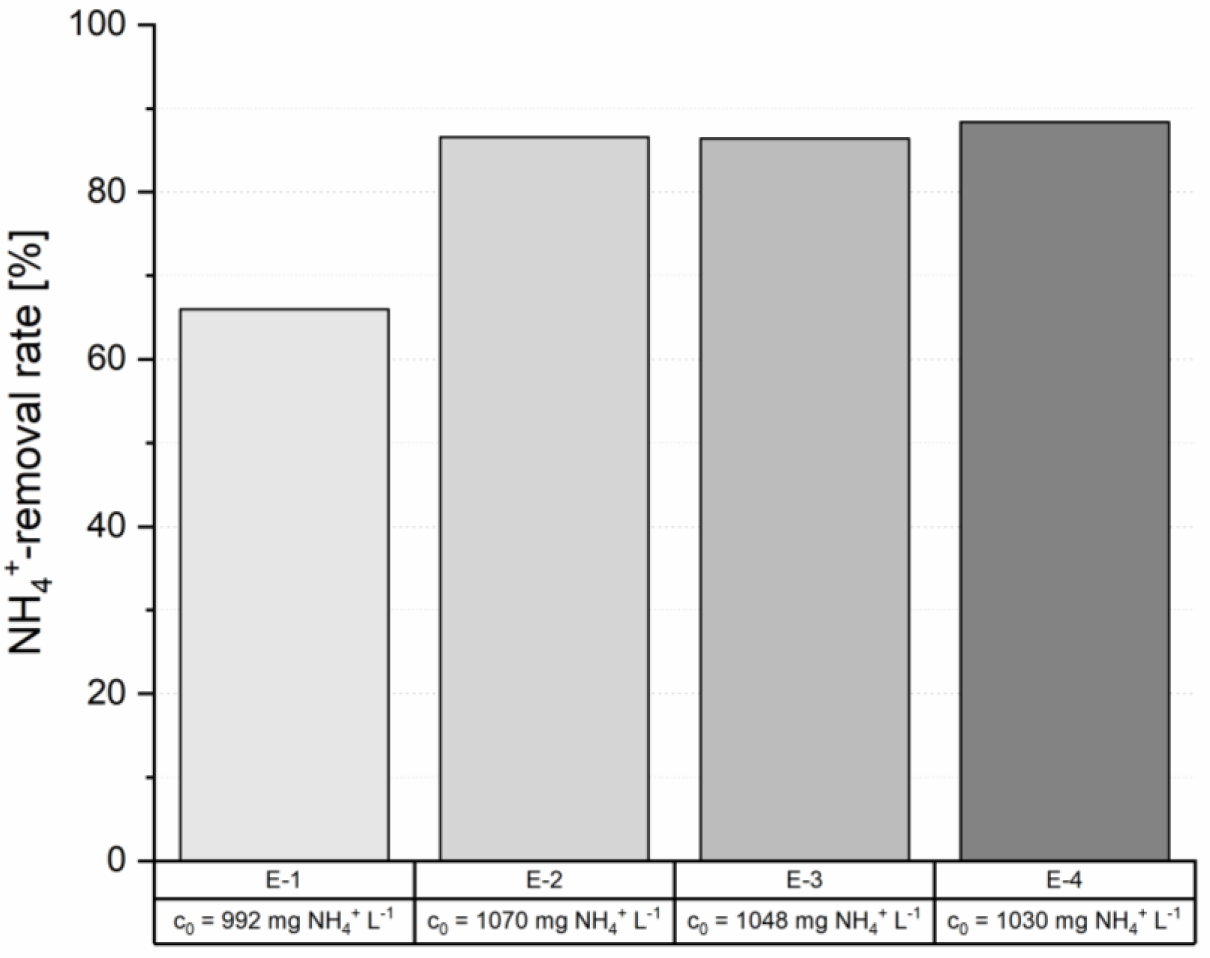
This increase derives from the exchange of the natural cation composition of the zeolite (Ca2+, K+, Na+, Mg2+) by Na+ from the NaOH regeneration solution which facilitates favorable ion exchange kinetics [23].
Fluctuations during operation of the waste water treatment plant resulted in varying ammonium concentrations in the sludge liquor. Therefore, experiments with similar feed concentrations were summarized, to compare the achieved removal rates (Table 2). 62 consecutive loading and regeneration cycles were carried out in total. 61 of them were used for the evaluations (E-38 was eliminated due to analytical errors). Loading E-1 was not taken into account in the calculation of the average removal rate in the concentration range of 800-1,100 mg NH4+ L−1, since no stable exchange equilibria were reached (Figure 2).
Average NH4+-concentrations and removal rates in the respective concentration range of the conducted loading cycles
Amount of operation cyclesa |
Concentration range [mg NH4+ L−1] |
Average feed concentration [mg NH4+ L−1] |
Removal rates [%] |
---|---|---|---|
5 |
500-800 |
671 ±111 |
83.6 ±7.1 |
14 |
800-1,100 |
1,026 ±69 |
85.8 ±6.3 |
36 |
1,100-1,500 |
1,302 ±116 |
83.6 ±5.2 |
5 |
> 1,500 |
1,975 ±256 |
71.8 ±11.9 |
Sum of evaluated operation cycles = 60 (run E-1 and E-38 discarded, cf. text)
Removal rates of 83.7 ±7.1% were obtained in the concentration range of 500-800 mg NH4+ L−1. The removal rates were slightly higher in the next concentration range (85.8 ±6.3% for 800-1,100 mg NH4+ L−1), provided that experiment E-1 (66.0%) is not considered due to the reasons mentioned above. Removal rates lower than 80% were observed in two experiments (E-46: 77.1% and E-47: 69.0%), whereas high values of 93.2 and 91.7% were achieved in the same concentration range as well [loading cycles E-5 and E-61 (Figure 3)].
The average removal rate is 83.6 ±5.2% in the concentration range of 1,100-1,500 mg NH4+ L−1, which comprises 36 loading and regeneration cycles in total. The lowest removal rate was obtained with 72.8% (E-48), while the highest removal rate is 91.9% (E-10).
An average removal rate of 71.8 ±11.9% was achieved in the five loadings in the highest concentration range above 1,500 mg NH4+ L−1. The loadings E-49 and E-50 showed exceptionally low values of 62.7 and 64.7%, respectively.
Removal rates of ammonium after 60 minutes and feed concentration of 61 loading cycles over one ion exchanger column (E-1 to E-62 without E-38) separated in four different concentration ranges: c0 = 500-800 (a); c0 = 800-1,100 (b); c0 = 1,100-1,500 (c) and c0 > 1,500 mg NH4+ L−1 (d)
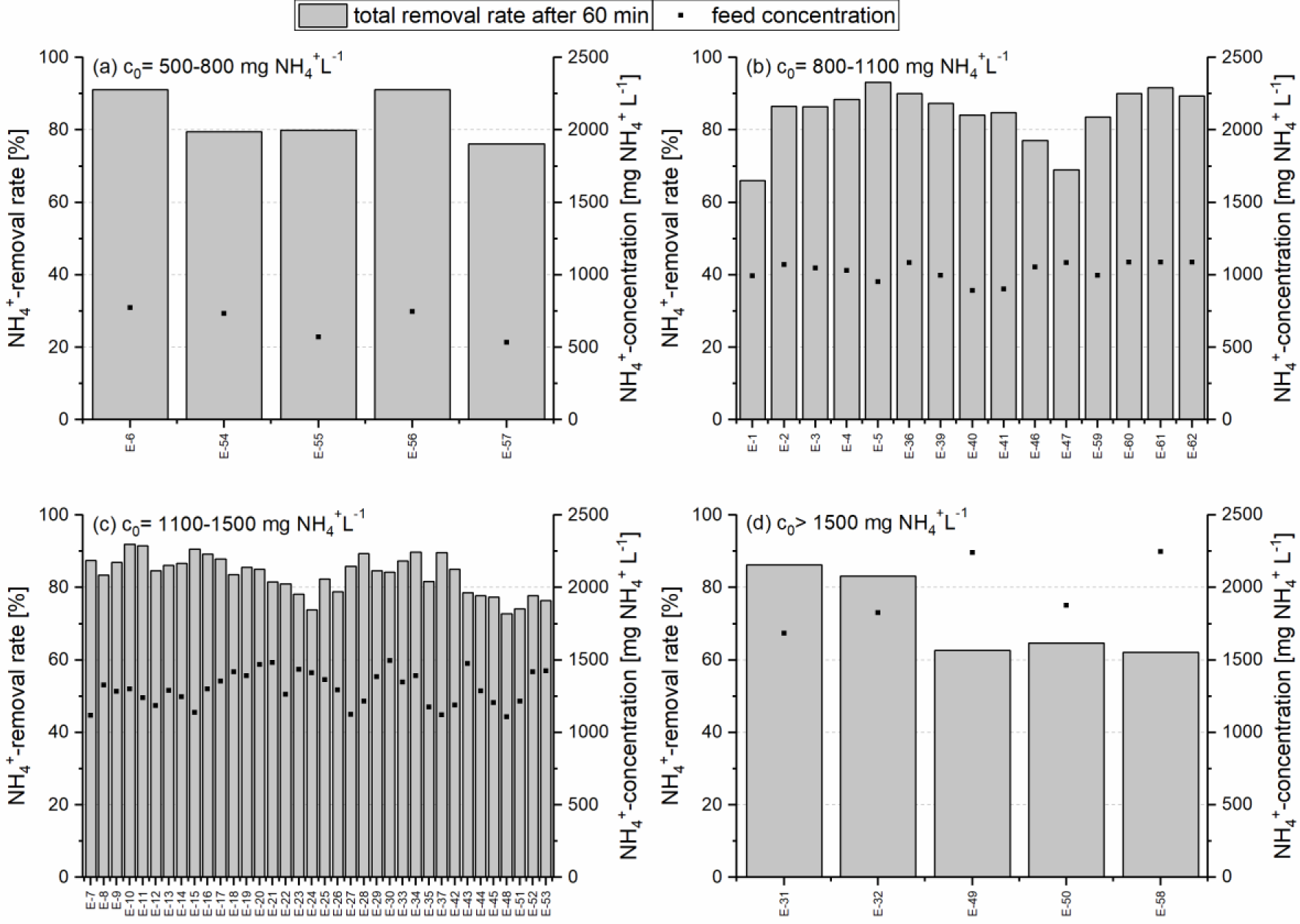
The removal rates of the individual loading cycles are directly related to the quality of the previous regeneration, which is in turn influenced by the equilibrium in the stripper as well as the pH value and the Na+ concentration of the regeneration solution. To keep the influence of the regeneration on the loading cycles as low as possible, the pH value of the regeneration and scrubbing solution was monitored and kept above 11 and below 2, respectively. Due to the complexity and the interaction of these process parameters, phases with lower removal rates can still occur. One or more consecutive insufficient regenerations may adversely affect the subsequent loading cycles, for example in the loading cycles E-47 to E-50. Besides these process-related variabilities, the removal rates remained at a constant level during the conducted experiments.
At sludge liquor concentrations above 1,500 mg NH4+ L−1, the removal rates are slightly lower compared to the other concentration ranges due to zeolite exhaustion. Significant increases in the removal rates can be achieved by increasing the amount of zeolite in the ion exchanger columns or – on the other hand, shortening of the loading times.
A significant increase of the removal rates was achieved in three loading cycles with a serial connection of two multiply loaded and regenerated ion exchanger columns (Figure 4). A NH4+-removal rate of 76.2% was obtained after 60 minutes after the first column, which increased up to 96.5% at the outlet of the second column in the loading cycle with a feed concentration of 534 mg NH4+ L−1 (E-A). After 120 minutes of loading NH4+-removal rates of 56.0% (C1) and 93.6% (C1 + C2) were achieved and after 180 minutes 44.2% (C1) and 84.1% (C1 + C2), respectively. This revealed an increase of the removal efficiency through the serial connection by 67.1% (120 minutes) and 90.3% (180 minutes).
The experiment series using sludge liquor with a feed concentration of 996 mg NH4+ L−1 (E-B) revealed similar increases in the NH4+-removal rates. In experiment B, the NH4+ removal rates increases from 55.2% after 180 minutes loading time after the first column to 90.1% through the serial connection.
Due to the high feed concentration of 2,246 mg NH4+ L−1 experiment C resulted in lower removal rates. Nevertheless, an increase of the removal efficiency by 48.2% (60 minutes) and 82.2% (180 minutes) was achieved.
Removal rates of ammonium after 60, 120 and 180 minutes over one (C1) and two serial (C1 + C2) ion exchanger columns with three different feed concentrations
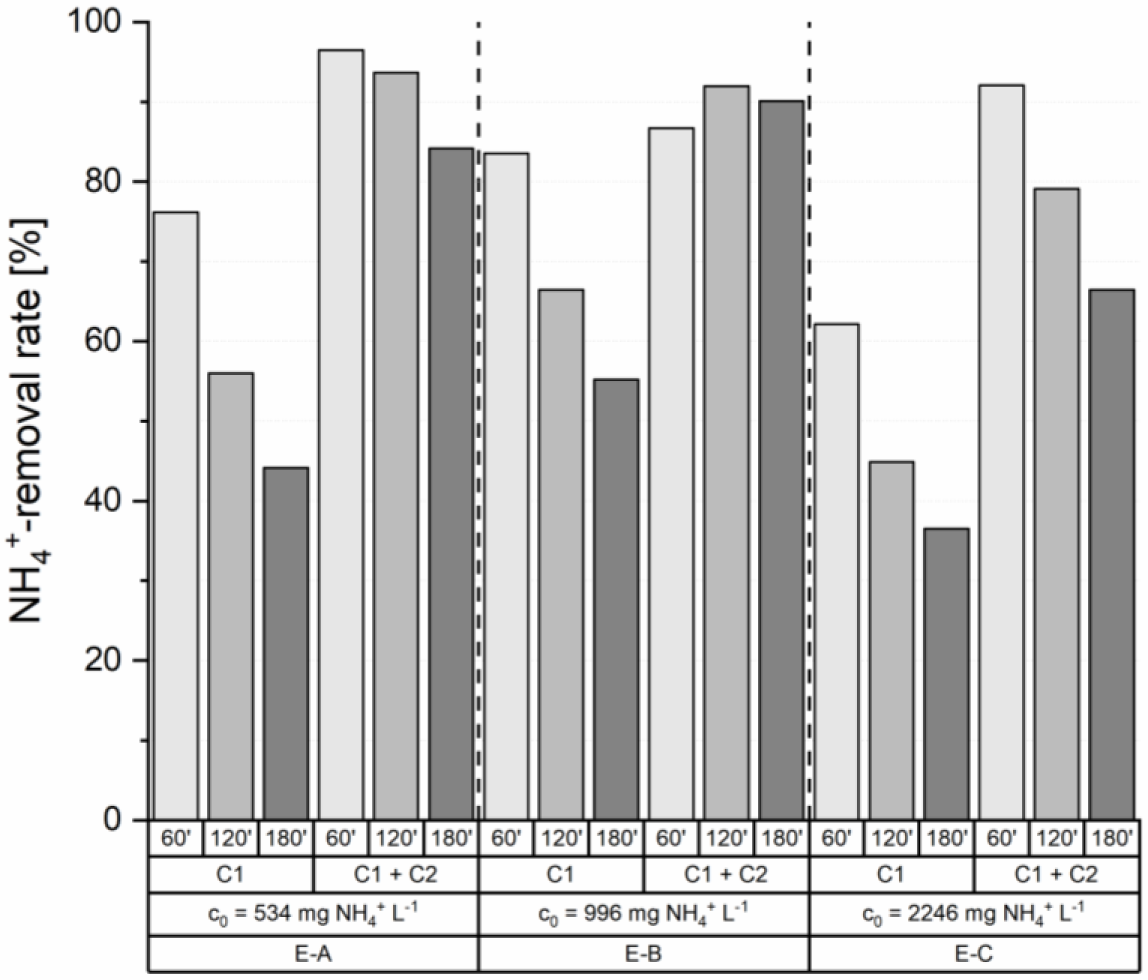
The results of these experiments (A, B and C) show that a serial connection of two ion exchanger columns and a doubling of the mass of ion exchanger material allows for a tripling of the loading time without reduction of the achievable NH4+ removal rates.
Pilot scale experiments with the ILS process showed that ammonium recovery with natural zeolite (clinoptilolite) from sludge liquor at the waste water treatment plant in Knittelfeld/Austria was successful. Ammonium removal rates increased significantly after the first NaOH regeneration and were stable in the following loading and regeneration cycles. Removal rates between 69-94% were achieved after 60 minutes of loading in a concentration range of 500-1,500 mg NH4+ L−1. Phases with higher and lower removal rates alternated during the experiments (standard deviation of ±7.4% in 62 experiments), which depended in particular on the feed concentration and process conditions during zeolite regeneration. Nevertheless, no ongoing decline in the removal rates was observed over 62 loading and regeneration cycles. The experiments revealed that over a wide concentration range of 500-1,500 mg NH4+ L−1 consistently high removal rates can be obtained (around 80%).
A serial connection of two ion exchanger columns showed that sludge liquor with concentrations above 2,000 mg NH4+ L−1 can be treated with removal rates > 80% after 60 minutes of loading. In addition, loading times can be tripled without observing a decrease in the ammonium removal rates.
The stability of the NH4+-exchange capacity of the applied zeolite was affirmed for numerous loading and regeneration cycles. This is a decisive criterion for a large-scale implementation of the ILS process and recommends the process for a wide range of applications.
Detailed energy and mass balancing is not possible with the current experimental setup of the pilot plant. To determine a detailed energy and chemical consumption, as well as performing cost calculation, further investigations are required.
The next steps will be to determine under which conditions the ILS process can be implemented for an economic nitrogen recovery. In addition, other biological waste waters like biogas manure, pig manure, landfill leachates or industrial waste waters, which potentially contain significant amounts of ammonium, will be investigated in order to expand the application field of the ILS process in future.
This work was conducted in part within the research project ‘ReNOx’ (FFG project 843673). Financial support from the Austrian Research Promotion Agency (FFG) is gratefully acknowledged. The authors would also like to thank the industrial partner Christof Industries and Lafarge Zementwerke GmbH for supporting the project.
Andreas Lechleitner, Eva Gerold, Sandro Pesendorfer and Francesca Capó Tous are thanked for experimental support.
- , Technology Evaluation of Digestate Treatment and Utilization Concepts (1st ed.) (in German), In-house Publication of the University of Natural Resources and Life Sciences Vienna, Tulln, Austria, 2010
Nitrogen Removal from Sludge Digester Liquids by Nitrification/Denitrification or Partial Nitritation/Anammox: Environmental and Economical Considerations ,Water Science & Technology , Vol. 50 (10),pp 19-26 , 2004
, Ammonium Removal from Digested Sludge Liquors Using Ion Exchange ,Water Research , Vol. 41 (2),pp 433-439 , 2007, https://doi.org/https://doi.org/10.1016/j.watres.2006.10.021
, - , , Anarobic Technology (2nd ed.) (in German), Springer-Verlag, Berlin, Germany, 2005
- Back-Contamination from Sludge Treatment ‒ Procedures for Sludge Liquor Treatment (in German), 2005, http://www.araconsult.at/download/literature/atv_jardin_wurrzburg.pdf, [Accessed: 25-June-2020]
Ammonia Stripping on a Thermophilic Biogas Plant ‒ Technical and Economic Evaluation (in German) ,Chemistry Engineer Technology , Vol. 81 (7),pp 921-932 , 2009, https://doi.org/https://doi.org/10.1002/cite.200900033
, - , Nitrogen Separation with the ANAStrip-process System GNS (in German), Fachagentur Nachwachsende Rohstoffe e.V., Gülzow-Prüzen, Germany, 2009
- , Possibilities of Nutrient Recovery and Fertilizer Production from Waste Waters (in German), Ostfalia University of Applied Sciences, Braunschweig/Wolfenbüttel, Germany, 2009
- , Adaptation and Development of the ANAStrip-process for Nitrogen Separation in Sludge Liquor from Waste Water Treatment Plants (in German), FFG-Project Nr. 831102, Final Report, Halle (Saale), Germany, 2013
Struvite Precipitation for Ammonium Removal from Anaerobically Treated Effluents ,Journal of Environmental Chemical Engineering , Vol. 3 (1),pp 413-419 , 2015, https://doi.org/https://doi.org/10.1016/j.jece.2015.01.004
, Natural Zeolites as Effective Adsorbents in Water and Wastewater Treatment ,Chemical Engineering Journal , Vol. 156 (1),pp 11-24 , 2010, https://doi.org/https://doi.org/10.1016/j.cej.2009.10.029
, - , Ion Exchange Separations with Molecular Sieve Zeolites, Zeolites: Science and Technology, Springer, Dordrecht, The Netherlands, 1984
Ammonium Sorption from Aqueous Solutions by the Natural Zeolite Transcarpathian Clinoptilolite Studied Under Dynamic Conditions ,Journal of Colloid and Interface Science , Vol. 284 (2),pp 408-415 , 2005, https://doi.org/https://doi.org/10.1016/j.jcis.2004.10.058
, Hydrothermal Modification of Natural Zeolites to Improve Uptake of Ammonium Ions ,Journal of Chemical Technology and Biotechnology , Vol. 80 (4),pp 376-380 , 2005, https://doi.org/https://doi.org/10.1002/jctb.1224
, - , Natural Zeolites in Water Treatment – How Effective is Their Use, Water Treatment, InTech, London, UK, 2013
Cation-Exchange Properties of Natural Zeolites ,Natural Zeolites: Occurrence, Properties, Applications , Vol. 45 (1),pp 453-518 , 2001, https://doi.org/https://doi.org/10.2138/rmg.2001.45.14
, Ion Exchange Separations with Molecular Sieve Zeolites ,Adsorption and Ion Exchange Separations, American Institute of Chemical Engineers, New York, USA , Vol. 74 (179),pp 98-116 , 1978
, Ammonium Nitrogen Removal from the Permeates of Anaerobic Membrane Bioreactors: Economic Regeneration of Exhausted Zeolite ,Environmental Technology , Vol. 35 (13-16),pp 2008-2017 , 2014, https://doi.org/https://doi.org/10.1080/09593330.2014.889759
, Simultaneous Regeneration of Exhausted Zeolite and Nitrogen Recovery Using an Air Stripping Method at Alkaline pH ,Water Quality Research Journal of Canada , Vol. 51 (4),pp 321-330 , 2016, https://doi.org/https://doi.org/10.2166/wqrjc.2016.007
, The Ion-Exchanger-Loop-Stripping Process: Ammonium Recovery from Sludge Liquor Using NaCl-Treated Clinoptilolite and Simultaneous Air Stripping ,Water Science & Technology , Vol. 77 (3),pp 695-705 , 2017, https://doi.org/https://doi.org/10.2166/wst.2017.561
, - , Integrated Production and Utilisation of Biogas in Cement Works (in German), Ph.D. Thesis, Montanuniversität Leoben, Leoben, Austria, 2012
Ammonium Recovery from Model Solutions and Sludge Liquor with a Combined Ion Exchange and Air Stripping Process ,Journal of Water Process Engineering , Vol. 32 ,pp 100909 , 2019, https://doi.org/https://doi.org/10.1016/j.jwpe.2019.100909
, Adsorption Mechanisms of High-levels of Ammonium onto Natural and NaCl-modified Zeolites ,Separation and Purification Technology , Vol. 103 ,pp 15-20 , 2013, https://doi.org/https://doi.org/10.1016/j.seppur.2012.10.005
,