Periurban horticultural practices are one of the most important activities in Buenos Aires Metropolitan Area (AMBA), Argentina. In this periurban area, pesticides and fertilisers are used without any control to cover the farmers’ needs obtaining high crop yields fast and producing modifications in soil ecosystem and structure in the long term. As agrochemicals were specifically designed for interfering in metabolic processes, it is important to understand the fate of these compounds in the environment. Horticultural soils receive high quantities of pesticides during fumigation steps [1], inducing changes in both macro and micro ecosystems, differently from the extensive farming. Particularly, these soils have been intensively exploited uninterruptedly during at least 20 years, showing clear signs of deterioration and low quality [2]. In addition, agrochemical application is performed by non-trained farmers with few economical resources who are currently exposed to consequent damage to health [3].
Soil is a natural complex system in which microorganisms and plants play relevant roles in keeping the natural equilibrium. Consequently, soil-plant-microbe interactions are so complex that they will have direct influence on the plant health and crop productivity [4]. The interactions that occur in the rhizosphere play a pivotal role in transformation, mobilization and solubilisation of a limited nutrient pool in the soil and subsequently define the uptake from each crop [6].
Beneficial free-living soil bacteria isolated from the rhizosphere, that proved to improve plant health or increase crop yields, are usually referred to as Plant Growth-Promoting Rhizobacteria (PGPR) [7], [8]. The importance of studying PGPR lies on their potential to be used as biofertilisers due to their capacity of, for example, producing 1-Aminocyclopropane-1-Carboxylate (ACC) deaminase to reduce the level of ethylene in the roots of the developing plants and produce hormones (auxins) like Indole-3- Acetic Acid (IAA) and cytokinins which also indirectly influence positively in nematode suppression [9]. Additionally, some strains may fix atmospheric nitrogen, solubilise mineral phosphates and mineralize other nutrients [10]. On the other hand, it is known that PGPR strains may produce siderophores with high Fe-complexing capacity causing an antagonist action against phytopathogenic micro or macroorganisms [9], [11]. As example, bacteria have been found to assist in root colonization by Rhizobia suppressing possible actions of plant pathogens, especially Pseudomonas and Bacilli behaving as PGPR associated to the rhizosphere of various leguminous crops [12], [13].
In addition, fungal communities were reported as good candidates for biofertiliser formulations by proving the improvement of plant growth and crop yields in many soils [14]. Particularly, rhizospheric yeasts are common soil microorganisms which showed plant growth-promoting capacities [15]. Yeasts are relevant for soil quality by influencing on soil aggregation, nutrient cycling and interaction with plants and soil animals [16]. It has been reported that some yeast genera produce antimicrobial substances and other exudates which enhance plant growth thanks to aminoacids and sugars secreted by bacteria. In consequence, some yeasts including Rhodotorula sp. have been studied by its potential capacity for the promotion of plant growth [17].
The immediate response to soil inoculation with PGPR varies considerably depending on the microorganism, plant species, soil type, inoculum density, and environmental conditions. The inoculated microorganisms sometimes do not survive in soil when competing with better-adapted indigenous microbiota [18]. Therefore, when formulating a biofertiliser it is highly recommended to consider the use of native bacteria, since they are better adapted to environmental conditions and are more competitive than non-native strains [19]. In fact, during the last decade the application of formulates based on autochthonous plant beneficial microbial consortia is being accepted as agrochemical substitutes to improve soil quality by using sustainable alternatives [20], [21]. In this way, the aim of this work was to isolate indigenous strains from periurban horticultural units with pesticide degrading capacity or at least tolerance and evaluate their plant growth-promoting properties in order to design potential biofertilisers to be applied in the restoration of these exploited soils.
The experimental work was performed following the methodology detailed below.
Commercial pesticides were used as culture medium supplements and formulates were selected according to each farmer application protocol:
Deltamethrin: DECIS®FORTE (Bayer Crop Science S.A.) 10% (m/v), CAS Number 52918-63-5. Active compound: [(S)-cyano-(3-phenoxyphenyl)methyl] (1R,3R)-3-(2,2-dibromoethenyl)-2,2-dimethylcyclopropane-1-carboxylate;
λ- Cyhalothrin: KARATE 5CS con tecnología ZEON® (Syngenta Agribusiness S.A.) 5% (m/v), CAS Number 68085-85-8. Active compound: [cyano-(3-phenoxyphenyl)methyl]3-[(Z)-2-chloro-3,3,3-trifluoroprop-1-enyl]-2,2-dimethylcyclopropane-1-carboxylate);
Iprodione: ROVRAL 50WP® (Bayer Crop Science S.A.) 50% (m/m), CAS Number 36734-19-7. Active compound: [3-(3, 5-dichlorophenyl)-2,4-dioxo-N-propan-2-ylimidazolidine-1-carboxamide];
Abacmectin: VERTIMEC® 018 EC (Syngenta Crop Protection AG), CAS Number: 71751-41-2. Active compound: mixture of ≥ 80% (2aE,4E,8E)-(5′S, 6S, 6′R, 7S, 11R, 13S,15S,17aR,20R,20aR,20bS)-6′-[(S)-sec-butyl]-5′,6,6′,7,10,11,14,15,17a,20,20a,20b-dodecahydro-20,20b-dihydroxy-5′,6,8,19-tetramethyl-17-oxospiro[11,15-methano-2H, 13H,17H-furo[4,3,2-pq] [2,6]benzodioxacyclooctadecin-13,2′-[2H]pyran]-7-yl2,6-dideoxy-4-O-(2,6-dideoxy-3-O-methyl-α-L-arabino-hexopyranosyl)-3-O-methyl-α-L-arabino-hexopyranoside and ≤ 20% (2aE,4E,8E)-(5′S,6S,6′R,7S,11R,13S,15S,17aR,20R,20aR,20bS)-5′,6,6′,7,10,11,14,15,17a,20,20a,20b-dodecahydro-20,20b-dihydroxy-6′-isopropyl-5′,6,8,19-tetramethyl-17-oxospiro[11,15-methano-2H,13H,17H-furo[4,3,2-pq][2,6]benzodioxacyclooctadecin-13,2′-[2H]pyran]-7-yl2,6-dideoxy-4-O-(2,6-dideoxy-3-O-methyl-α-L-arabino-hexopyranosyl)-3-O-methyl-α-L-arabino-hexopyranoside.
Samples were taken from two farms located in Cuartel V, Moreno District, Buenos Aires, Argentina (Figure 1) during spring 2014 ‒ spring 2016. Sites were selected according to the land use and 11 samples were taken from the Horticultural unit 1:T (tomato crop plot) and Y (plot destined to diverse crops as cabbages, leek and green onion) both from Mr. Alejandro Yucra farm (34° 34’ 54.58” S 58° 49ʼ 26.79” W and 34° 34ʼ 53.32” S 58° 49ʼ 24.60” W, respectively), from the Horticultural unit 2:S (Mr. Severino Choque farm, 34° 34ʼ 33.69” S 58° 48ʼ 49.71” W) was initially a strawberry crop plot, P (Mr. Severino Choque farm, 34° 34ʼ 34.96” S 58° 48ʼ 48.90” W) was the pesticide formulation preparation area and R (34° 34ʼ 39.31” S 58° 48ʼ 44.38” W), the reference grassland close to the productive plots which was not used for production and there was no evidence of anthropogenic perturbations for almost 20 years. In all campaigns, random samples of each site until 10 cm depth were taken and the resulting soil composite was preserved in polyethylene bags.
A physicochemical characterization of soil (Order Mollisols, Suborder Udolls, Great Group Argiudolls, Subgroup Vertic Argiudolls) was previously performed. Main differences were found in Organic Matter-C:N values, conductivity and copper concentration. Both sampled R soils, spring 2014 and fall 2015, showed low conductivities with highest Organic Matter-C:N values. S and Y spring 2014 soils evidenced high copper concentration related to the utilization of Cu-based agrochemicals as the fungicide Cotacuatro®, which decreased in fall 2015 samples studies [22].
None of the sampling sites was located in protected areas. Sampling permissions were obtained from each farm owner: Mr. Severino Choque at S, P and R soils and Mr. Alejandro Yucra at Y and T soils. For access to sampling sites, special permission was obtained from Instituto Municipal de Desarrollo Económico Local (IMDEL) and Moreno District municipality.
Sample site locations of Cuartel V, Moreno, Buenos Aires Metropolitan Area: Horticultural unit 1 with T and Y plots and Horticultural unit 2 with S, P and reference plots
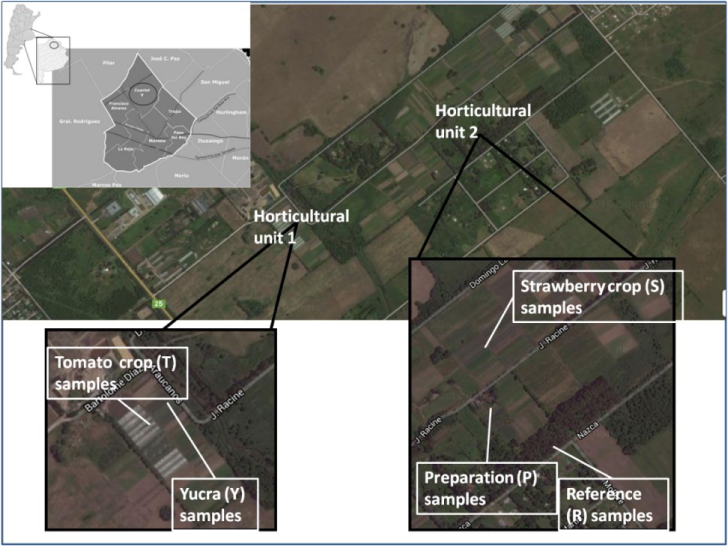
Soil (1 g) was suspended in 10 mL of physiological solution and 0.1 mL of serial 1:10 dilutions of the suspension (10−1 to 10−5) were spread by duplicates in Soil agar (soil 125 g/L, glucose 1% m/v, agar 15.2 g/L, mix of pesticides 0.1% v/v) or in Plate Count agar (PCA, g/L: tryptone 5, glucose 1, yeast extract 2.5, agar 12). The different pesticide mixtures were prepared according to the declared use by farmers in each field for production. For the samples of Horticultural unit 1, the mix was composed by deltamethrin and λ-cyhalothrin (P2) and for Horticultural unit 2 soils a mix of deltamethrin, abamectin and iprodione was used (P1). Plates were incubated up to seven days at 32 °C. Counts were expressed as CFU/g soil with the corresponding standard deviation calculation. Colonies grown in presence of pesticides were selected and purified in PCA twice at 32 °C for testing plant growth-promoting characteristics.
Nitrogen fixing bacteria were counted in soil samples as follows [23]: soil (1 g) was suspended in 10 mL of physiological solution and 0.1 mL of serial 1:10 dilutions of the suspensions were spread by duplicates in Burk’s agar, which composition per l is: K2HPO4 0.8 g, KH2PO4 0.2 g, MgSO4·7H2O 0.2 g, NaCl 0.2 g, CaSO4 0.01 g, Fe-Mo 1 mL (FeCl3·6H2O 1.45 g, Na2MoO4·2H2O 0.253 g in 100 mL H2O), sucrose 20 g, H3BO3 100 µg, ZnSO4·7H2O 100 µg, MnSO4·4H2O 10 µg, CuSO4·5H2O 3.0 µg, KI 1.0 µg, agar 20 g. Plates were incubated at 32 °C during seven days and CFU/g soil were calculated with the corresponding standard deviation.
For an enrichment step, 1 g of soil was suspended in 10 mL of physiological solution (150 mM NaCl) and an aliquot was incorporated to a minimal medium (g/L: K2HPO4 7.3, KH2PO4 3.0, NH4Cl 1.0, MgSO4 0.1220, CaCl2·2H2O 0.01 M) supplemented with 1% v/v of the corresponding pesticide mix (P1 or P2) as the only carbon source. After 15 days of incubation at 120 rpm and 32 °C, cultures were transferred to agar plates containing the same minimal medium supplemented with agar (15 g/L) and 0.1% v/v of the same mix of pesticides used in the enrichment step. Plates were incubated at 32 °C up to 30 days, obtaining isolated colonies which were finally purified in PCA plates.
Strains isolated from horticultural soils were evaluated for Plant Growth-Promoting (PGP) properties.
Isolated bacteria were tested by streaking in Burk’s agar, according to Stella and Suhaimi [23]. Plates were incubated at 32 °C seven days, if growth was observed, the result was considered positive for N2 fixation.
Phosphate solubilisation capacity of horticultural strains was evaluated. Growth and presence of solubilisation halo were evaluated after streaking strains in National Botanical Research Institute Phosphorus (NBRIP) agar (g/L: MgCl2·6H2O 5.0, MgSO4·7H2O 0.25, KCl 0.2, (NH4)2SO4 0.1, Ca3(PO4)2 5.0, glucose 10.0, agar 15.0) and incubating 48 h at 32 °C [24].
Production of IAA was quantified by spectrophotometric technique [25]. Strains were inoculated by duplicates in Tryptone Broth (TB, g/L: casein peptone 20.0, NaCl 5.0) and incubated during 7 days at 32 °C. After incubation, cultures were centrifuged at 7,000 g 15 min. Supernatants and Salkowski reagent (12 g/L FeCl3 in 7.9 M H2SO4) reacted during 30 min in darkness after mixing 1 mL of each. Absorbance was registered at 530 nm with a linear range of 0.5-25 μg IAA/mL.
On the other hand, IAA production kinetics was studied. Culture supernatants with high auxin levels were selected to monitor IAA production along microbial growth. Each preinoculum was carried out in TB and incubated overnight to be transferred to fresh TB (2 mL to 20 mL). During an incubation of seven days at 32 °C, supernatant samples were taken at 24, 96 and 144 h and IAA was determined following the above mentioned protocol. In all cases, DO at 600 nm was measured for biomass estimation.
In a deeper analysis, Y5 strain was incubated in TB during 22 days at 30 °C and 120 rpm. Samples were taken at 48, 168, 240 and 528 h. IAA quantification was performed as mentioned above.
Strains were inoculated in M9 medium (g/L: K2HPO4 7.3, KH2PO4 3.0, NH4Cl 1.0, MgSO4.7H2O 0.246, CaCl2 0.01, succinate 5.0, yeast extract 0.1) formulated with deionized water to ensure iron absence. After incubating at 32 °C seven days and centrifuging 15 min at 6,300 g, culture supernatants were placed in plates with CAS-agar. CAS-agar (100 mL) was prepared according to Schwyn and Neilands [26] with modifications [27]. 7.5 mL of 2 mM Chrome Azurol S were mixed with 1.5 mL iron solution (1 mM FeCl3.6H2O, 10 mM HCl). Under stirring, 6.0 mL of 10 mM cetyltrimethylammonium bromide (CTAB) solution were added and the resulting blue solution was kept at 50 °C. In addition, buffer solution was prepared using 0.6 g of piperazine-N, N′-bis(2-ethanesulfonic acid) (PIPES) dissolved in 85 mL in ultrapure water (18 MΩ cm, Millipore) adjusting a final pH to 6.8 using 6 M KOH. To this solution, 1.5 g agar-agar was added and then heated at 50 °C to dissolve it. The blue and the agar solutions were finally mixed, and Petri dishes (10 cm diameter) were prepared with 30 mL of CAS-agar each. After gelling, 5 mm diameter holes were made and dried 1 h at 32 °C. Each hole was filled with 75 µl culture supernatant. A non-inoculated medium as negative control and cell-free supernatant from Pseudomonas aeruginosa PA01 cultures [28], [29] were used as positive control. An orange colour halo appearance after 24 h at room temperature was considered positive for siderophore production.
Morphological, biochemical and molecular characterizations were performed for strains positive for IAA/siderophore production, N2 fixation or Ca3(PO4)2 solubilisation.
Biochemical characterization as a preliminary approach was made using API20E®, API20NE®, APICHB® and API 20A® kits (Bio Mérieux), as required for each isolate. Finally, molecular identification was achieved by analysis on sequences and confirmation of microorganism’s homogenic data using rDNA database (NCBI) after amplification of Bacteria or Fungi’s ribosomal RNA gene. PCR on 16S rDNA was performed using 27F and 1492R primers, and yielding of 1,300 bp or more sequencing data using internal primers 785F, 907R. Only one strain was characterized by analysis on 18S rDNA region sequences, with a guaranteed length greater than 1,600 bp (Macrogen Korea).
Selected strains Y1, Y5, Y8a, Y13b and P13b2 were evaluated for potential inhibitory interactions between them. First they were separately grown overnight in Nutrient Broth. Each culture was swabbed in PCA plates and after a few minutes, 50 µl of the each other culture were discharged over the swabbed surface. Plates were incubated at 32 °C for 48 h and bacterial growth was observed. In addition, S1-2, SP-3 and RP7 were tested in the same way and also inoculated over Y5-swabbed surface and treated as detailed above.
Main results of the screening are presented according the followed steps in the experimental design.
Results of the CFU/g soil are shown in Figure 2 for samples belonging to the first campaign in Soil agar and in PCA. Microbial CFU/g soil were 1.5 Log higher in plates without pesticides than in plates with the products (Figure 2), with no significant differences between soils. PCA counts were clearly 2 Log higher than the corresponding counts in Soil agar, an oligotrophic medium. In addition, a screening of N2-fixing microorganisms was carried out in soil samples collected recovering a range from 2.1 to 6% of PCA counts without significant differences among them, according to the overlapping of the respective confidence intervals.
About 59 strains were isolated from both reference and horticultural soils after the enrichment step: 15 strains from Y, 19 from P, 5 from S, 8 from T and 9 from R.
A total of 14 colonies from plates with pesticides were selected from soil agar. From Burk’s agar, 13 strains were isolated: 4 from T, 3 from S, 2 from Y and 4 from R, while no strains were isolated from P.
Bacterial counts (CFU/g soil) of samples (S, P, T and R) from 2014 campaign in different culture media and presence of pesticides regularly applied in farms: bars indicate confidence intervals according to the calculated standard deviation
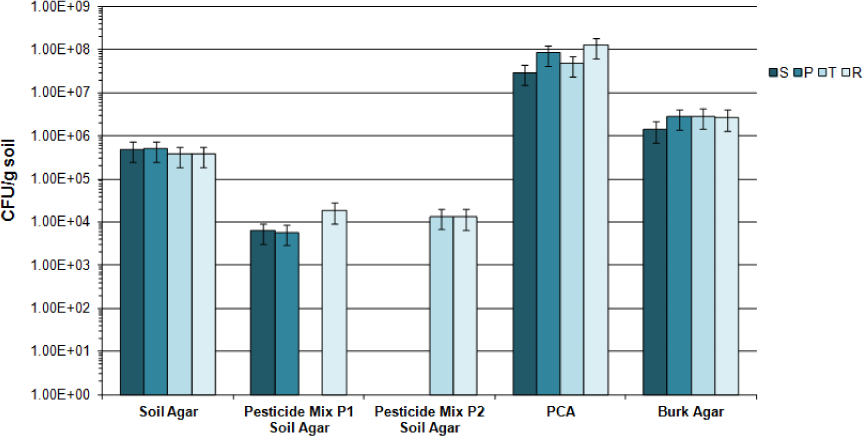
Once the strains were adapted to pesticide presence, they were tested in Burk’s agar to evaluate N2 fixation. Thirty six strains isolated with an enrichment step were able to grow, the major proportion coming from Y and P (13 and 14, respectively). On the other hand, isolates from soil agar for strains named S1-2 and SP-10 (from S, R and P, respectively) were positive for N2 fixation. Regarding phosphate solubilisation, Figure 3 illustrates the results of a considerable halo formation. Eight positive strains were detected from soil agar isolates: three from S, two from P and four from R. Between the isolates from the enrichment media, 26 were able to solubilise calcium phosphate. All microorganisms from Y samples were positive for this assay, being only one strain negative. Regarding Burk’s agar isolates, five came from R, 3 from T, 1 from S and 1 from Y. Taking Pseudomonas aeruginosa PA01 as positive control for siderophores in CAS agar, horticultural bacteria were tested. For all isolates, production of siderophores was negative, except for SP-3, RP7 and SP-10. For IAA production tests, isolates were specifically selected according to previous results: the combination of positives for Burk’s agar growth, Ca3(PO4)2 solubilisation, siderophore biosynthesis and culturability. A summary of these results was compiled in Table 1, which shows the maximal IAA concentrations obtained in batch cultures.
Screening of calcium phosphate solubilisation capacity by halo formation in NBRIP medium
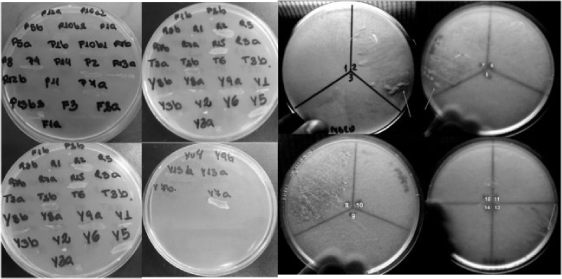
Regarding IAA production kinetics, results are shown in Figure 4. Nine strains were selected for these assays for being best IAA producers (see Table 1), with different behaviours in growth. IAA production was closely related to microbial growth with an exponential increase during the experiment. Relevant IAA concentrations were detected for Y5 (0.981 µg/mL), Y8a (0.961 µg/mL), Y13b (1.942 µg/mL), P13b2 (1.335 µg/mL), S1-2 (7.733 µg/mL), SP-3 (2.969 µg/mL), RP7 (2.824 µg/mL) and SP-10 (3.283 µg/mL). Particularly in the case of Y8a, maximum IAA synthesis was registered between 24 and 96 h (0.968 µg/mL), decreasing up to 0.758 µg/mL in 144 h despite the increasing bacterial growth. Finally, Y1 showed a poor growth with a detectable concentration of 0.794 µg IAA/mL at 96 h, finally decreasing to values comparable to control experiments.
Analysis of plant growth-promoting properties performed on microbial isolates
Strain |
Burk’s agar |
Ca3(PO4)2 solubilisation |
Siderophore production |
Maximal IAA [µg/mL] |
---|---|---|---|---|
S1-2 |
+ |
+ |
- |
7.733 |
SP-3 |
- |
+ |
+ |
2.969 |
RP7 |
- |
+ |
+ |
2.824 |
SP-10 |
+ |
+ |
+ |
3.283 |
Y5 |
- |
+ |
- |
5.217 |
Y8a |
+ |
+ |
- |
1.361 |
P13b2 |
- |
+ |
- |
1.335 |
Y13b |
+ |
+ |
- |
2.419 |
Y1 |
+ |
+ |
- |
3.130 |
IAA production kinetics assay of agrochemical tolerant isolates: Y1, Y5, Y8a, Y13b and P13b2 were obtained after the enrichment step, while S1-2, SP-3, RP7 and SP-10 resulted from Soil agar cultures
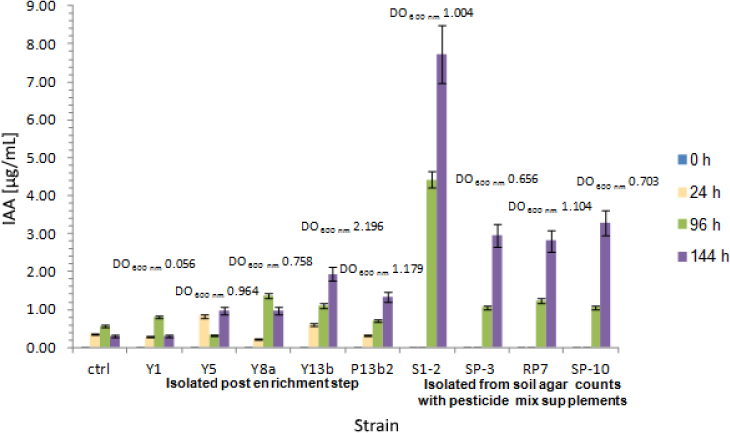
Focusing on IAA production by the yeast Y5, an increase in IAA concentration during 528 hours of a complementary analysis was observed, reaching a maximum of 13.21 µg IAA/mL at the end of the experiment after 22 days of growth consistent with the stationary phase (Figure 5).
Eight strains were finally selected for identification and future studies, according to their PGP properties and better culturability. Although the data obtained from the biochemical characterization performed with API® tests was absolutely preliminary and could not give an identity beyond the genera, they contributed to complement the information of the 16S/18S rRNA gene sequences. In fact, results obtained matched the biochemical characteristics of the strains. Isolates from Soil agar SP-3 and SP-10 were identified as Sphingobium yanoikuyae (NCBI GenBank Accession Number MN215404), S1-2 as Pseudomonas migulae (DDBJ Accession Number LC494531) and strain RP7 as Leucobacter aridicollis (NCBI GenBank Accession Number MN212958). Regarding the isolates obtained after the enrichment step, Y5 was identified as Rhodotorula mucilaginosa (NCBI GenBank Accession Number MN215307), Y13b as Bacillus toyonensis (NCBI GenBank Accession Number MN215308), P13b2 as Bacillus megaterium (NCBI GenBank Accession Number MN215309) and Y8a as Bacillus safensis (NCBI GenBank Accession Number MN215310).
Kinetics of IAA production for Rhodotorula mucilaginosa Y5 extended to 22 incubation days
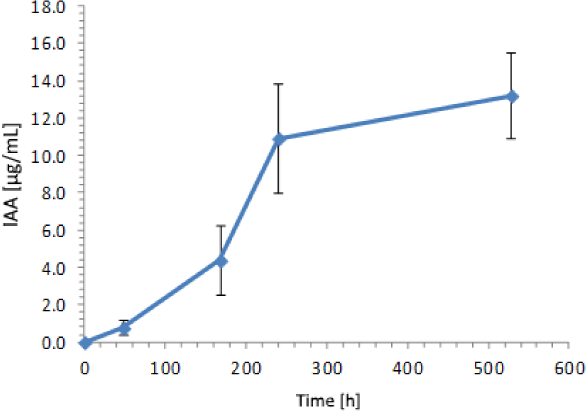
To evaluate potential mixed culture formulations, the results showed that there was a negative interaction since Y1 did not grow in presence of the other strains. Only in the plate where Y13b was swabbed, Y1 grew in a minimal proportion. An inhibition halo around Y8a culture drops was detected against P13b2 grass. There were no appreciable growth interferences between P13b2-Y13b-Y5, Y8a-Y13b-Y5 and S1-2, SP-3, RP7 growth over Y5 surface. In this last case, the evaluation was made only using strain Y5 because of being a yeast with typical inhibitory characteristics against other microorganisms, as already described in literature [30], [31].
References about environmental isolates, with evident combination of PGP properties as in this study, are insufficient. Even though there are reports about the diverse capacities and functions of the strains in environment, they are not focused on the direct applications of PGP microorganisms on horticultural soils as in this case [32], [33].
PCA and Soil agar counts were significantly different, evidencing the nutritional conditions to which microorganisms were exposed at. PCA is a rich culture medium allowing growth to culturable copiotrophs microorganisms while Soil agar reproduced the natural environment selecting culturable oligotrophic microorganisms. Hence, different culturable soil populations were selected according to their requirements, but not necessarily represent the majority of the microbial community members. The ‘great plate count anomaly’ should be considered when microbial communities belonging to natural samples are evaluated by this method, since only approximately 0.1-1.0% of the total bacteria can be enumerated by the plating procedure. In fact, the maximum recovery of heterotrophic bacteria is 1% of the total direct count using plating procedures from a variety of oligotrophic to mesotrophic aquatic habitats, including soils as in this case [34]. Despite these limitations, the enumeration of heterotrophs still contributed to the soil status information. For example, CFU/g obtained in PCA counts (ca. 108) are in agreement to other responses found in literature for polluted soils [35]. Additionally, CFU/g registered in Soil agar evidenced the susceptibility of a fraction of the culturable population towards pesticide exposition, regardless the soil sample.
Interestingly, the enrichment broth constituted a selective step for the isolation of pesticide degrading/tolerant microorganisms. In fact, four strains were selected from horticultural soils by an enrichment step in two different mixes of pesticides: deltamethrin, iprodione and abamectin on one hand, and deltamethrin and λ-cyhalotrin on the other. Strains were characterized as Bacillus toyonensis, Bacillus safensis, Bacillus megaterium and Rhodotorula mucilaginosa. Bacillus megaterium strain P13b2 was isolated using the first mix of pesticides while strains Y13b (B. toyonensis), Y8a (Bacillus safensis) and Y5 (Rhodotorula mucilaginosa) grew in presence of the other mix. The fact that three of the strains were characterized as Bacillus sp. (Phylum Firmicutes) is consistent with the results described by Kumar et al. [36], who reported that this genus has an advantage over the non-spore formers such as Pseudomonas, due to spores being more robust and resistant to elevated temperatures and high concentrations of chemicals. In reference to this study, the presence of spores could facilitate the development of new biofertilisers in order to improve horticultural practices. On the other hand, Rhodotorula yeasts, in particular Rhodotorula mucilaginosa, found in extreme environments, were reported to be able to grow using different carbon sources [37]. These yeasts could be the responsible of pesticide metabolization for example, since it is known that some genera as Rhodotorula and Candida can use phenolic compounds as unique source of carbon. Strains were tested according to their capacity for producing IAA, solubilising calcium phosphate and fixing atmospheric nitrogen. P13b2, Y3b and Y8a were positive in all experiments and Y5 was only negative for nitrogen fixation, consistent with its belonging to Eukarya Domain.
Focusing on the strains isolated from Soil agar, three species that belonged to quite different taxonomical classification than the group obtained through the enrichment step were identified. All of them were able to solubilise calcium phosphate and to produce IAA, promissory PGP capacities in which were not reported up to now. On one hand, Sphingobium sp. (Phylum Proteobacteria, Class α-Proteobacteria) is a strain described as characteristic in terms of degradation of piretroids and isoproturon [38]. On the other hand, although Leucobacter sp. (Phylum Actinobacteria) has been associated with remediation of soil and wastewater contaminated with chrome and nickel [39], Liu et al. [40] have proposed alternative ways for biodegradation of triazines. On a third side, Pseudomonas migulae is often found in soil isolates, particularly from polluted environments related to mine areas [41]. Leucobacter aridicollis RP7, which was isolated from Soil agar in presence of pesticides, came from the R samples. It is interesting to mention that although this strain was not originally adapted to pesticides, it has shown a positive growth in their presence. Beyond this, as it was expected P and Y samples have more isolates with resistance to the mix of pesticides.
Respecting to Y5 analysis, it is known that yeasts improve crops because of a fast phosphorus solubilisation, and phytohormones production [42]. So, the efficient rates of IAA production found in this study ‒ 13.2 µg/mL as maximal concentration achieved in 22 days ‒ are consistent with other reports. In addition, as already mentioned above Rhodotorula genus is reportedly capable of metabolizing phenolic compounds [43], so Rhodotorula mucilaginosa Y5 represents an important tool to complement bioremediation with biofertilisation techniques in these horticultural soils.
Inhibitory activity was evaluated to know whether the selected strains could coexist in mixed cultures. Results suggest that isolates from S, Y, P and R soils may be good alternatives to work on the development of mixed bioinoculants. Particularly, Y1 strain would not be considered because of its growth inhibition in presence of the other strains. In consequence, the proposed mixed cultures:
Bacillus megaterium P13b2, Bacillus toyonensis Y13b, Rhodotorula mucilaginosa Y5;
Bacillus safensis Y8a, Bacillus toyonensis Y13b, Rhodotorula mucilaginosa Y5;
Pseudomonas migulae S1-2, Sphingobium yanoikuyae SP-3, Leucobacter aridicollis RP7, Rhodotorula mucilaginosa Y5 would be the chosen candidates for the potential restoration of pesticide contaminated soils by bioaugmentation procedures.
After the screening, a total of eight strains were isolated from different horticultural unit soils. Microorganisms were identified as Sphingobium yanoikuyae, Pseudomonas migulae, Leucobacter aridicollis, Bacillus toyonensis, Bacillus megaterium, Bacillus safensis and the yeast Rhodotorula mucilaginosa. They demonstrated capacity to produce IAA, to solubilise calcium phosphate, to fix nitrogen (except the yeast), three of them to secrete siderophores and, on the other hand, were able to resist/metabolize a mix of pesticides that normally are used for horticultural production. By observing the positive behaviour of them in mixed cultures, there would be an alternative for development of biofertilisers according to type of soil and activity. Next steps will include the evaluation of different biodegradable materials as cell immobilisation matrices. Bioinoculants formulated with these immobilised native bacteria will contribute to the quality restoration of these exploited soils and will allow applying sustainable horticultural practices improving productivity with the avoidance of an environmental damage.
This work was supported by the Universidad Nacional de General Sarmiento, Universidad de Buenos Aires, Consejo Nacional de Investigaciones Científicas y Tecnológicas (CONICET) and grant PIO CONICET FYPF2014-2015 Nº13320130100204CO.
Abbreviations |
|
ACC |
1-Aminocyclopropane-1-Carboxylate |
AMBA |
Buenos Aires Metropolitan Area |
CFU/g soil |
Colony Forming Units per g Soil |
CTAB |
Cetyltrimethylammonium Bromide |
IAA |
Indole-3- Acetic Acid |
IMDEL |
Local Economic Development Municipal Institute (Instituto Municipal de Desarrollo Económico Local) |
NBRIP |
National Botanical Research Institute Phosphorus |
P1 |
Deltamethrin, Abamectin and Iprodione Mixture |
P2 |
Deltamethrin and λ-cyhalothrin Mixture |
P |
Pesticide Formulation Preparation Area of Mr. Severino Choque Farm |
PCA |
Plate Count Agar |
PCR |
Polymerase Chain Reaction |
PGP |
Plant Growth-promoting |
PGPR |
Plant Growth-Promoting Rhizobacteria |
PIPES |
Piperazine-N,N′-bis(2-ethanesulfonic Acid) |
R |
Reference Grassland Close to the Productive Plots Which Was Not Used for Production and There Was no Evidence of Anthropogenic Perturbations for Almost 20 Years |
S |
Strawberry Crop Plot of Mr. Severino Choque Farm |
T |
Tomato Crop Plot of Mr. Alejandro Yucra Farm |
TB |
Tryptone Broth |
Y |
Plot Destined to Diverse Crops as Cabbages, Leek and Green Onion of Mr. Alejandro Yucra Farm |
Environmental Pesticide Distribution in Horticultural and Floricultural Periurban Production Units ,Chemosphere , Vol. 87 (5),pp 566-572 , 2012, https://doi.org/https://doi.org/10.1016/j.chemosphere.2011.12.074
, Environmental Fate of Trifluralin, Procymidone and Chlorpyrifos in Small Horticultural Production Units in Argentina ,Water, Air, and Soil Pollut. , Vol. 225 , 2014, https://doi.org/https://doi.org/10.1007/s11270-014-1952-7
, Potential Dermal Exposure to Deltamethrin and Risk Assessment for Manual Sprayers: Influence of Crop Type ,Sci. Total Environ. , Vol. 391 (1),pp 34-40 , 2008, https://doi.org/https://doi.org/10.1016/j.scitotenv.2007.09.034
, Extending Thermotolerance to Tomato Seedlings by Inoculation with SA1 Isolate of Bacillus Cereus and Comparison with Exogenous Humic Acid Application ,PLoS ONE , Vol. 15 (4), 2020, https://doi.org/https://doi.org/10.1371/journal.pone.0232228
, Inoculation with Indole-3-Acetic Acid-Producing Rhizospheric Rhodobacter Sphaeroides KE149 Augments Growth of Adzuki Bean Plants Under Water Stress ,J. Microbiol. Biotechnol. , Vol. 30 (5),pp 717-725 , 2020, https://doi.org/https://doi.org/10.4014/jmb.1911.1106
, The Contribution of Arbuscular Mycorrhizal Fungi in Sustainable Maintenance of Plant Health and Soil Fertility ,Biol. Fertil. Soils , Vol. 37 (1),pp 1-16 , 2003, https://doi.org/https://doi.org/10.1007/s00374-002-0546-5
, Biofertilizers with Beneficial Rhizobacteria Improved Plant Growth and Yield in Chili (Capsicum Annuum L.) ,World J. Microbiol. Biotechnol. , Vol. 36 (6), 2020, https://doi.org/https://doi.org/10.1007/s11274-020-02863-w
, Screening and Assessment of Potential Plant Growth-promoting Bacteria Associated with Allium Cepa Linn ,Microbes Environ. , Vol. 35 (2), 2020, https://doi.org/https://doi.org/10.1264/jsme2.ME19147
, Plant Growth Promoting Rhizobacteria (PGPR): A Potential Alternative Tool for Nematodes Bio-Control ,Biocatal. Agric. Biotechnol. , Vol. 17 ,pp 119-128 , 2019, https://doi.org/https://doi.org/10.1016/j.bcab.2018.11.009
, Potential PGPR Properties of Cellulolytic, Nitrogen-Fixing, Phosphate-Solubilizing Bacteria in Rehabilitated Tropical Forest Soil ,Microorganisms , Vol. 8 (3), 2020, https://doi.org/https://doi.org/10.3390/microorganisms8030442
, Growth Promotion and Yield Enhancement of Peanut (Arachis Hypogaea L.) by Application of Plant Growth-Promoting Rhizobacteria ,Microbiol. Res. , Vol. 159 (4),pp 371-394 , 2004, https://doi.org/https://doi.org/10.1016/j.micres.2004.08.004
, Effect of Specific Plant-Growth-Promoting Rhizobacteria (PGPR) on Growth and Uptake of Neonicotinoid Insecticide Thiamethoxam in Corn (Zea Mays L.) Seedlings ,Pest Manag. Sci. , Vol. 71 (9),pp 1258-1266 , 2015, https://doi.org/https://doi.org/10.1002/ps.3919
, Bacillus Telluris sp. nov. Isolated from Greenhouse Soil in Beijing, China ,Microorganisms , Vol. 8 (5), 2020, https://doi.org/https://doi.org/10.3390/microorganisms8050702
, Soil Fungal Communities Differ Between Shaded and Sun-Intensive Coffee Plantations in El Salvador ,PLoS ONE , Vol. 15 (4), 2020, https://doi.org/https://doi.org/10.1371/journal.pone.0231875
, Effect of Inoculation with Soil Yeasts on Mycorrhizal Symbiosis of Maize ,Pedobiologia , Vol. 50 (4),pp 341-345 , 2006, https://doi.org/https://doi.org/10.1016/j.pedobi.2006.06.002
, Assessment of Yeast Diversity in Soils Under Different Management Regimes ,Fungal Ecol. , Vol. 5 (1),pp 24-35 , 2012, https://doi.org/https://doi.org/10.1016/j.funeco.2011.07.004
, Effect of Soil Amendment with Yeasts as Bio-Fertilizers on the Growth and Productivity of Sugar Beet ,Afr. J. Agric. Res. , Vol. 8 (1),pp 46-56 , 2013, https://doi.org/https://doi.org/10.5897/AJAR12.1989
, Arachis Hypogaea PGPR Isolated from Argentine Soil Modifies its Lipids Components in Response to Temperature and Salinity ,Microbiol. Res. , Vol. 173 ,pp 1-9 , 2015, https://doi.org/https://doi.org/10.1016/j.micres.2014.12.012
, Perticari, A. and Correa, O. S., Genotypic Characterization of Azotobacteria Isolated from Argentinean Soils and Plant-Growth-Promoting Traits of Selected Strains with Prospects for Biofertilizer Production ,Sci. World J. , Vol. 2013 , 2013, https://doi.org/https://doi.org/10.1155/2013/519603
, From Isolation of Phosphate Solubilizing Microbes to Their Formulation and Use as Biofertilizers: Status and Needs ,Front. Bioeng. Biotechnol. , Vol. 7 (425), 2020, https://doi.org/https://doi.org/10.3389/fbioe.2019.00425
, Formulation of Microbial Inoculants by Encapsulation in Natural Polysaccharides: Focus on Beneficial Properties of Carrier Additives and Derivatives ,Front. Plant Sci. , Vol. 11 (270), 2020, https://doi.org/https://doi.org/10.3389/fpls.2020.00270
, Biomarkers in Eisenia Andrei Exposed to Fruit and Vegetable Soils Treated with Chlorpyrifos, Copper Oxychloride and Myclobutanil (in Spanish) ,Acta Toxicológica Argentina , Vol. 23 ,pp 50 , 2015
, Selection of Suitable Growth Medium for Free-Living Diazotrophs Isolated from Compost ,J. Trop. Agric. and Fd. Sc. , Vol. 38 (2),pp 211-219 , 2010
, An Efficient Microbiological Growth Medium for Screening Phosphate Solubilizing Microorganisms ,FEMS Microbiol. Lett. , Vol. 170 (1),pp 265-270 , 1999, https://doi.org/https://doi.org/10.1111/j.1574-6968.1999.tb13383.x
, A Critical Examination of the Specificity of the Salkowski Reagent for Indolic Compounds Produced by Phytopathogenic Bacteria ,Appl. Environ. Microbiol. , Vol. 6 (2),pp 793-796 , 1995, https://doi.org/https://doi.org/10.1128/aem.61.2.793-796.1995
, Universal Chemical Assay for the Detection and Determination of Siderophores ,Anal. Biochem. , Vol. 160 (1),pp 47-56 , 1987, https://doi.org/https://doi.org/10.1016/0003-2697(87)90612-9
, Chemical Characterization and Ligand Behaviour of Pseudomonas Veronii 2E Siderophores ,World J. Microbiol. Biotechnol. , Vol. 34 (134), 2018, https://doi.org/https://doi.org/10.1007/s11274-018-2519-3
, Isolation of an Iron-Binding Compound from Pseudomonas Aeruginosa ,J. Bacteriol. , Vol. 137 (1),pp 357-364 , 1979, https://doi.org/https://doi.org/10.1128/jb.137.1.357-364.1979
, Metal Regulation of Siderophore Synthesis in Pseudomonas Aeruginosa and Functional Effects of Siderophore-Metal Complexes ,Appl. Environ. Microbiol. , Vol. 58 (9),pp 2886-2893 , 1992, https://doi.org/https://doi.org/0099-2240/92/092886-08$02.00/0
, Carotenoids from Rhodotorula and Phaffia: Yeasts of Biotechnological Importance ,J. Ind. Microbiol. Biotechnol. , Vol. 36 (2),pp 163-180 , 2009, https://doi.org/https://doi.org/10.1007/s10295-008-0492-9
, Biocontrol of Postharvest Gray and Blue Mold Decay of Apples with Rhodotorula Mucilaginosa and Possible Mechanisms of Action ,Int. J. Food Microbiol. , Vol. 146 (2),pp 151-156 , 2011, https://doi.org/https://doi.org/10.1016/j.ijfoodmicro.2011.02.015
, Evaluation of Heavy Metals Tolerant Bacterial Strains as Antioxidant Agents and Plant Growth Promoters ,Biocatal. Agric. Biotechnol. , Vol. 19 , 2019, https://doi.org/https://doi.org/10.1016/j.bcab.2019.101110
, Isolation and Identification of a Phosphate-Solubilizing Paenibacillus Polymyxa Strain GOL 0202 from Durum Wheat (Triticum Durum Desf.) Rhizosphere and its Effect on Some Seedlings Morphophysiological Parameters ,Biocatal. Agric. Biotechnol. , Vol. 19 , 2019, https://doi.org/https://doi.org/10.1016/j.bcab.2019.101087
, A Hidden Pitfall in the Preparation of Agar Media Undermines Microorganism Cultivability ,Appl. Environ. Microbiol. , Vol. 80 (24),pp 7659-7666 , 2014, https://doi.org/https://doi.org/10.1128/AEM.02741-14
, Bioremediation of Diesel Fuel Contaminated Soil: Comparison of Individual Compounds to Complex Mixtures ,Soil Sediment Contam. , Vol. 15 (3),pp 277-297 , 2006, https://doi.org/https://doi.org/10.1080/15320380600646332
, Bacillus Strains Isolated from Rhizosphere Showed Plant Growth Promoting and Antagonistic Activity Against Phytopathogens ,Microbiol. Res. , Vol. 167 (8),pp 493-499 , 2012, https://doi.org/https://doi.org/10.1016/j.micres.2012.05.002
, Carotenoids Production by the Yeast Rhodotorula Mucilaginosa: Use of Agricultural Wastes as a Carbon Source ,Process Biochem. , Vol. 40 (9),pp 2985-2991 , 2005, https://doi.org/https://doi.org/10.1016/j.procbio.2005.01.011
, Enhanced and Complete Removal of Phenylurea Herbicides by Combinational Transgenic Plant-Microbe Remediation ,Appl. Environ. Microbiol. , Vol. 84 (14), 2020, https://doi.org/https://doi.org/10.1128/AEM.00273-18
, Leucobacter Chromiireducens sp. nov., and Leucobacter Aridicollis sp. nov., Two New Species Isolated from a Chromium Contaminated Environment ,System Appl. Microbiol. , Vol. 27 (6),pp 646-652 , 2004, https://doi.org/https://doi.org/10.1078/0723202042369983
, Enhanced Degradation of Prometryn and Other S-Triazine Herbicides in Pure Cultures and Wastewater by Polyvinyl Alcohol-Sodium Alginate Immobilized Leucobacter sp. JW-1 ,Sci. Total Environ. , Vol. 615 ,pp 78-86 , 2018, https://doi.org/https://doi.org/10.1016/j.scitotenv.2017.09.208
, Draft Genome Sequence of Se(IV)-Reducing Bacterium Pseudomonas Migulae ES3-33 ,Genome Announcements , Vol. 3 (3), 2015, https://doi.org/https://doi.org/10.1128/genomeA.00406-15
, Exploration of Novel Rhizospheric Yeast Isolate as Fertilizing Soil Inoculant for Improvement of Maize Cultivation ,J. Sci. Food Agric. , Vol. 95 (7),pp 1491-1499 , 2015, https://doi.org/https://doi.org/10.1002/jsfa.6848
, Isolation and Characterization of Phenol Degrading Yeasts from Wastewater in the Coking Plant of Zarand, Kerman ,Braz. J. Microbiol. , Vol. 47 (1),pp 18-24 , 2016, https://doi.org/https://doi.org/10.1016/j.bjm.2015.11.032
,