Healthcare waste (HW) is any non-reusable and discarded medical waste generated after clinical, healthcare, and self-care activities such as diagnosis, therapeutic, and inoculation of humans and animals [1], [2]. Management of healthcare waste (MHW) globally and particularly in developing countries has become a major concern because of increasing volume of HW produced [3]. This could be due to the development in medical technologies, increasing world population [4], easy access to the health-cares facilities [5], and non-reusability and thus unsustainability of most of the medical devices [6].
World health organization (WHO) estimated that around 75%–90% of the total waste generated by the health care activities worldwide is non-toxic or general HW (gHW) while only 10%–25% could be considered as hazardous healthcare waste (hHW) [7]. This estimation is subjected to change and depends on the type of healthcare facility, regulations, the effectiveness of waste segregation, awareness of waste handling staff, storage of the different types of waste in their respective bins.
Diaz et al. summarized the waste generation data from several healthcare facilities in Ulaanbaatar, Mongolia and estimated the medical waste to be from 14% to 38% of the total waste stream generated by these facilities [8]. The medical waste from forensic lab (up to 98%) and from health care consultation facilities seemingly represent two extremes of the same end [8]. It is important to note that the amount of waste generated by healthcare facilities in developed countries is quite large (1.2 to 200+ times) as compared to the developing countries, yet the infectious waste percentage in developed countries is about 51% compared to 63% in developing countries [8]. Additionally, specific regulations associated with different facilities such as labs, hospitals and workspaces in different countries can define the scope of “healthcare waste” differently. To illustrate, a tissue used to cover the mouth while coughing and sneezing is generally segregated as a general waste, however in the current COVID-19 pandemic, such tissues can be treated as infectious waste depending on the healthcare facility (as observed during visits in UAE hospitals and testing centres dealing specifically with COVID-19 testing) [9].
HW shares a high proportion of total waste being produced worldwide. In 1991, the estimated generation of HW in USA was over 3 billion kg while China produced around 700 million kg in 2005 [10], [11]. Li et al. evaluated the chemical and physical composition of the HW from National Taiwan University Hospital (NTUH) [12]. It was reported that 99.02 wt.% of HW was combustible. This implies that, HW could be used to heat recovery option as the reported average heat value was 14225.6 kJ/kg. Bujak assessed the heat recovery potentials of HW [13]. This was done through thermal treatment in an incinerator coupled with heat recovery system (HRSG). Average thermal efficiency of HRSG was reported as over 80%.
HW composition varies greatly from source to source. It contains organic constituents that could either be replaced with recyclable precursors or if that is not possible, then it may be reused after recycling such as paper and plastics. Besides, organic parts of the HW have the potential to produce biofuels and other valuable chemicals. This could be done after segregation from both gHW and hHW which is neither reusable nor can be used to produce valuable chemicals. This sustainable approach of recycling, reusing and biofuels production would not only reduce environmental burden but also produce valuable fuels which could be used in diverse applications.
Manegdeg et al. investigated the electricity generation potentials of HW via pyrolyzer-rankine cycle [14]. Compositions of the biofuels produced from HW, including infectious healthcare waste (iHW), were as: synthetic gas (94.1%), biochar and bio-oil (5.9%). H2 (48.2%) and CO (22.6%) were the major constituents of the synthetic gas. Meanwhile, the reported efficiencies for the system (pyrolyzer-rankine cycle) and Rankine cycle power plants were 17.8% and 35.5%, respectively. It is worth noting that the authors compared microware, autoclave, plasma, gasification, incineration, bio-digestion, liquefaction, and pyrolysis. Manegdeg et al. [14] report the highest score for pyrolysis for medical waste treatment.
Hazardous healthcare wastes (hHW) may possess, one or more of, toxic, infectious, radioactive, corrosive, combustive, irritating, reactive chemicals and other hazardous constituents posing health and safety threats to occupational as well as general population. Underdeveloped countries are producing as much as 0.2 kg of infectious clinical waste per bed per day [15]. Unfortunately, due to the improper segregation practices and mixing of the hHW with gHW, the overall quantity of urban hHW raised significantly [16] .
Harhay et al. hypothesized that more than half of the world’s population is living under health risks due to inappropriate HW management [17]. Moreover, the threats of pandemics and other viral diseases are not only looming but also are swallowing thousands of lives every year [18]. A recent yet major such outbreak of highly resistant corona virus (COVID-19) affected over seventy five million people across the globe (as of December, 2020) while it caused 1.66 million deaths along with huge economic and financial losses [19]. To avoid such major and unprecedented epidemics, there is a strong need for efficient management and treatment of hHW before disposal.
Meanwhile, given the COVID-19 pandemic, it is imperative for low-resource countries to adopt the strict waste disinfection and safe disposal practices [20]. Infectious waste generated from hospitals, municipal and households activities where COVID-19 patients are either being treated or in quarantine need special attentions [21], [22]. It was speculated that improper disinfection and slight ignorance in handling of the iHW waste could prolong outbreak and cause its episodic recurrence [23], [24]. It is reported that on plastic materials and steel surfaces, COVID-19 virus could stay over 72 h [25].
The objective of this work is to review sustainable management practices of healthcare waste (SMHW) and recent treatment technologies. This might help environmental professionals and policy makers in taking informed decisions about how to select suitable yet efficient and sustainable HW treatment methods at on-site or off-site facilities. To keep the discussion coherent and simple, the term healthcare waste (HW) is used to refer to all types of healthcare wastes (HWs) whereas general and hazardous healthcare wastes are denoted by gHW and hHW, respectively, throughout the manuscript. The term infectious healthcare waste (iHW) is used to put emphasize only on potential infection containing and/or spreading HW.
Over the past few decades, there has been substantial advances in modern medicine and healthcare that span from substantial reductions in deaths from malaria, tuberculosis, HIV/Aids and vaccine-preventable diseases to early detections of diseases such as brain tumours, Parkinson’s and cancer [26], [27]. It is an undeniable fact that health care facilities and health care workers strive for better life for mankind (in general) and patients (in particular). There are several causes of health problems including, but not limited to, unhealthy living style, unhealthy environment, unintended contact with pathogens and ageing. However, it is important to note that the major cause of health problems and environmentally mediated higher mortality rate is ambient air pollution (1 in 8 deaths globally) [28]. Studies have revealed that the healthcare facilities are, ironically, one of the major contributors to environmental pollution and carbon-footprints representing around 3–10% of the total national CO2 equivalent emissions in England, Australia, and USA [29]. HW is the refuse produced in healthcare and diagnostic activities including, but not limited to, hospitals, pharmaceuticals, clinics, dentists, physicians, and laboratories. Nature of HW, origin, and risk associated with HW are listed in Table 1.
SMHW is a complex process, and it is imperative to implement cradle-to-grave approach to understand, control, and eliminate (or minimize) the environmental and economic burdens of such waste on the society. Careful planning from stakeholders, thorough research (both qualitatively and quantitatively) and substantial efforts are needed for sustainable HW management. The details and responsibilities of key stakeholders have already been discussed in details in the literature [30], [31]. SMHW involves a well-developed waste management approach also known as waste management hierarchy (Figure 1) which includes pollution prevention (P2), reduction, reuse, recycle, recovery, treatment, and disposal in order of preference, with P2 being the most desirable and disposal being the least desirable.
Major emphasis needs to be given to the prevention and reduction of HW generation at first place as it would ultimately reduce HW [32]. Though it is unrealistic to suggest complete prevention of waste generation, several methods can be implemented to reduce the waste, particularly hazardous waste generation. Waste reduction, in a sustainable way, can be realized with the help of healthcare staff members; it requires a whole paradigm shift of healthcare practices. For instance, purchasing supplies that can be consumed less comparatively and are more environment friendly. Mercury-free thermometers and use of digital x-rays are just few examples in such cases.
SMHW is a comprehensive term and involves a much more in-depth approach than mentioned above. The segregation of wastes into different categories based on their nature and treatment methods is a crucial part of this system. General guidelines, bags colour codes, and temporary storing of HW are given in Table 2.
The enormity of these processes could be imagined by the fact that around 50% hHW is originally general waste. That implies it could be managed along with municipal waste after due classification and segregation. Doing this would significantly reduce the cost and environmental burden due to overall reduction and thus unnecessary treatment of the hHW.
Types of Healthcare wastes and associated risks
Nature of HW |
Origin |
Associated risks |
Sharps |
Syringes and surgical instruments |
Risks of injury and puncture |
Radioactive |
Nuclear medicines, radiology unit wastes |
Hazards associated with radioactive elements |
Pressurized vessels |
Gas cylinders and aerosol containers |
Leak, burst and explosion |
Contagious |
Blood, body fluids and secretions |
Contamination risks |
Anatomical |
Human or animal body parts and tissues |
Contamination risks |
Infectious |
Cultures and wastes from infected patients |
Risk of infection propagation |
Pharmaceutical |
Partially used medicines, discarded, and expired drugs |
Environmental risks |
Cytotoxic |
Cytotoxic drugs |
Health and environmental risks |
Heavy metals |
Batteries and mercury waste from broken instruments (i.e. thermometers and manometers) |
Safety, health, and environmental risks |
Chemicals |
Chemical substances (i.e. disinfectants and solvents) |
Reactivity and safety risks |
Waste management hierarchy – applicable to healthcare waste management, prevention being the most preferable; https://www.epa.gov/smm/sustainable-materials-management-non-hazardous-materials-and-waste-management-hierarchy
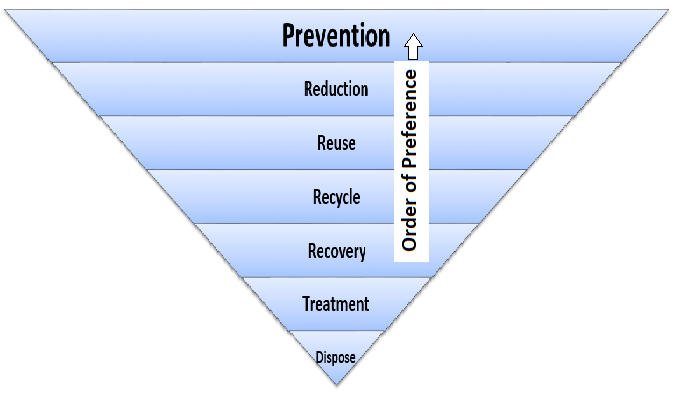
Adu et al. further discussed the sorting and management of HW [33]. The hazardous waste though is mostly around 5–15 % of total HW, if not properly segregated, will contaminate the entire waste which in turn will require special treatment and incur additional costs. Such hazardous waste has the potential, directly or indirectly, to cause large-scale environmental and health risks. For example, pyrolysis or incineration of hazardous waste containing heavy metals such as mercury will contaminate ground and water bodies.
A recent research survey conducted by Gill et al. (2020) reports that estimated combustible waste from ear, nose, and throat (ENT) surgeries in UK alone is around 100,000 kg/year [34], [35]. This could produce huge carbon footprint upon incineration. To reduce the carbon emissions and ecological imbalance owning to these surgeries, biomedical staff need to re-think on their strategies and should consider sustainable surgical alternatives. Lately, efforts are being employed on sustainability of the health care equipment and devices. This is being done by shifting and replacing the medical equipment made of single use plastics to sustainable and reusable ones which may be reused after sterilization [36].
General guidelines in sorting and storing of Healthcare wastes
Types of waste |
Colour codes |
Type of bag/container |
General/Non-hazardous waste (gHW) for incineration |
Black |
Plastic bag |
Sharps |
Yellow |
Sharps container |
Infectious HW with chemicals contamination |
Yellow and a “highly infectious” mark |
Plastic bag/container which can be autoclaved |
Infectious waste without chemicals contamination |
Orange and a “highly infectious” mark |
Plastic bag/container which can be autoclaved |
Contagious |
Yellow |
Plastic bag/container |
Anatomical |
Yellow |
Plastic bag/container |
Chemical |
Brown and a “highly infectious” mark |
Plastic bag/container |
Pharmaceutical |
Brown and a “highly infectious” mark |
Plastic bag/container |
Recyclable waste |
Clear/blue |
Plastic bag/container |
Food/organic waste |
Brown |
Plastic bag/container |
It is important to realize that strong international and national legislations along with adequate policies (for healthcare centres) have been formulated, however their implementation is still a critical problem and a matter of great concern in developing countries. Unawareness coupled with mishandling of HW has brought forward evidence of mixing of health care waste with municipal waste, illegal recycling and reselling and open air burning of hazardous waste [37]. This dictates the severity and seriousness of the issues at hand and needs to be addressed with sustainable management techniques.
Heath highlighted the scope of the issues related to futility and waste generated within biomedicine industry [38]. These issues include production of unnecessary medicines, misuse of the natural resources, excessive costs to patient for clinical facilities, and biomedicine manufacturing corruptions. This issue is being monitored by Cochrane – an international charitable organization for sustainable healthcare development through medical research and counselling. In 2014, it was reported that 613 active pharmaceuticals were declared as non-suitable for ecosystem whereas international biomedical industry produce 55% more emissions than automobile industry [39].
Auditing and legal investigations of healthcare institutes, at least once a year, could be another step forward to bring attitudinal improvements in better utilization of medical resources and waste reduction [40]. British environmental agency took one such initiative and is holding dental and biomedical clinics accountable for their clinical practices including waste production and its management [40]. It is estimated that around 60% of waste generated in phacoemulsification surgeries has a potential to be recycled and recovered for reuse [41].
Treatment of HW is carried out with different processes and it depends on many variables. Details are given in a separate heading below. HWs after treatment are disposed in landfilling sites which are more common in third world countries. Poorly segregated waste destined to go to landfills could cost up to 185 USD per ton of waste [40]. Illegal landfilling is very common in poor and underdeveloped countries. Moreover, burgeoning intravenous drug users globally could render improper handling of syringes, needles and other drugs which could transfer deadly blood borne viruses such as human immunodeficiency viruses (HIV) and hepatitis B virus (HBV) [42].
Collection of HW should start from the most hygienically sensitive areas, i.e., intensive care units (ICUs), operation theatres (OTs) and emergency wards, etc. Special care must be taken in classification, sorting, and collection of iHW. Fines may be imposed on inappropriate segregation of HW.
Sorted HW must be kept away from patients, visitors, and other unauthorized hospital staffs. It must never be left unattended at any time in any open place. In case of non-availability of special utility rooms for temporary storage, it can be stored in closed containers which should be carefully labelled and be placed away from common reach. For HW, if generated in huge quantities, designated central waste storage areas should be installed and used. From there, it should be either sent to onsite- or may be transported to off-site treatment facilities. Taghipour et al. conducted a study on the challenge of deciding whether a HW needed to be treated on-site or it would be required to send off-site for this purpose [43].
General requirements for central HW storage area are as follows: (a) impermeable floor with water supply and drainage system, for easy cleaning; (b) separate storage facilities for gHW and hHW; (c) easily accessible to waste handling and collection vehicles; (d) must be away from food storage and food processing areas; (e) marked as an unauthorized area for common people; (f) out of reach of animals, birds or insects; (g) have enough storage capacity to handle all the waste generated from the facility for the specified period; (h) equipped with first aid and (i) must be kept clean and ventilated at all times.
Transportation of HW is an important management step. Care must be practiced while transporting hHW within the facility or away from the waste producing facility. Within the facility, separate trolleys, with marked labels, must be used for gHW and hHW. In medical facilities, usually, black, and yellow painted trolleys are used for gHW and iHW, respectively. Trolleys should be easy to clean and they should not be too high to restrict visibility of the handling staff and should have appropriate waste carrying capacity. There should not be any sharp edges on the trolley to avoid injury and/or leaks during transportation.
HW offsite transportation, maybe, for treatment is a risky step, especially in case of hHW [44]. It is very crucial to transport gHW and hHW, separately. This step involves carrying of HW from central storage areas of healthcare facilities and its transportation to treatment plants where it may be incinerated, disposed, discarded, dumped, or destroyed by different treatment methods. Treatment of the waste is discussed in the subsequent sections. Transportation to off-site treatment facility requires expertise and special skills. Therefore, logistic staffs must have training and knowledge of safe handling, basic risk and hazard management in case of emergency during its transportation [7]. Taslimi et al. modelled the risk associated with the transportation and storage of medical waste to offsite treatment [44].
To keep the transportation process smooth and environmentally friendly, specially designed HW handling containers and vehicles must be used. These vehicles should meet the following criteria: (a) equipped with spill kits, protective clothes, empty plastic bags, small containers, and other cleaning tools to handle any emergency; (b) easily cleanable from inside and free from any sharp edges and (c) easily recognizable and mounted with visible emergency contact numbers.
Thermal, chemical, irradiation, and biological methods are being employed in the treatment of HW. Waste effluents from hospitals could be treated with ozone [45], ultraviolet (UV) irradiation [46], chlorine dioxide (ClO2) [47], chlorine (Cl2) [48], and sodium hypochlorite (NaClO) [49]. In this section, different technologies employed in HW treatment, their specified applications and limitations are discussed.
Thermal technologies are among the most common methods used in HW treatment because they could accommodate a vast range of generated waste [50]. Thermal technologies are further classified as high-heat and low-heat treatment technologies. Incineration of waste usually carried out at high temperatures thus it is classified as a high-heat technology. It is one of the most widely used HW treatment methods and it is estimated that around 60% regulated HWs are treated by this method [51]. Incineration of HW is done at the temperature range between 700 to 1200 ℃. Its primary purpose is to eliminate all the hazards and reduce volume of the waste. It could reduce the waste volume up to 95% [51], [52]. HW is typically incinerated in three different types of incineration units, starved air incinerators, excess air incinerators and rotary kiln.
Starved/controlled air incinerators. HW treatment in starved/controlled incinerators, is carried out in two chambers. Initially, waste is sent to the first (lower) chamber where heat is applied either without oxygen (i.e. to pyrolyze) or with small amount of oxygen (i.e. to gasify in lower amount of stoichiometric oxygen than required for complete combustion). In either case, organic parts of HW produce combustible gases. Produced gases help in raising the temperature of the incineration process and thus improve the overall efficiency of the process. Temperature in the first chamber can range between 760 and 985 ℃ [53].
The gases along with the left-over waste from the first chamber are sent to the second (upper) chamber. There, complete combustion of the waste is done with excess oxygen. The temperature in this chamber typically can range from 980 to 1,095 ℃. Average HW treatment capacity for this type of incinerators is typically ranged between 0.6 and 50 kg/min. Trinh et al. used the air-flow-controlled incinerator to treat the solid HW [54]. To increase the efficiency of the process and better heating, a drying chamber (before carbonization chamber) was added to the incinerator. This chamber operates between 70 to 200 ℃ and significantly reduces the moisture content of the HW. Air velocity positively affects the efficiency of the incinerator. As the velocity increased from 0.8 to 1.1 m/s, combustion time is decreased up to 15 minutes.
Excess air incinerators. These incinerators typically come in small units containing two main chambers with several small internal chambers and baffles. It consists of two chambers, primary and secondary. Each chamber is equipped with one or two burners which provide the required heat and maintain the operating temperature (typically 870 to 980 ℃). Waste is incinerated in two stages. In the first step, HW is sent to the primary chamber where it is combusted. In the secondary chamber, enough residence time and temperature are provided to ensure the complete combustion of unburnt organics and gases from the first chamber. Excess air incinerators or batch incinerators typically operate between 60 and 200% excess air with the HW feed capacity of 3.8 kg/min [55].
Rotary kilns. HW rotary kilns incinerators typically have two chambers i.e. primary and secondary. Primary chamber normally consists of rotating round shaped kiln which is often placed horizontally with a slight inclination. On the contrary, secondary chamber is a vertical hopper shaped compartment which is connected to the primary chamber to pass produced gases.
Waste is fed to the primary chamber where it is combusted, volatiles and other gases are produced due to the combustion reactions. It is equipped with auxiliary burner(s) to initiate combustion and maintain the desired temperature. Inclination along with the rotary motion moves the burnt waste towards the end of the primary chamber where ash is collected. Sometimes baffles are added to the inner circumference of the kiln to improve the burning.
Emission from the kiln is sent to the secondary combustion chamber. Unburnt particulate matters and incomplete combusted volatiles in the flue gases from first chamber are burnt thoroughly with excess air. Secondary chamber temperature can reach as high as 1315 ℃ [53]. Rotary kilns are often continuous system. Kilns can be manufactured in different sizes, depending upon the volume of waste to be treated.
Low-heat thermal processes. Autoclaving and steam sterilization for HW treatment are usually operated at temperatures between 93 to 177 ℃ in a tightly sealed chamber [51]. Wet and dry heat treatments are two different types of low-heat thermal processes. In wet-heating, steam is used to kill pathogens. However, the effectiveness of HW treatment by steam autoclave is questionable. Tiller et al. evaluated the steam autoclaving for sterilization of HW . Out of 22 samples studied, bacterial regrowth was reported for 18 samples; this implies the autoclave treatment is effective for 18% of the cases only. Ineffectiveness was attributed to the inability of the steam to reach the far depth of the iHW but comparatively with higher residence time; thus, shredder and mechanical mixing could improve the autoclave disinfection.
According to some reports, the penetration of steam can be improved and pathogens can reduce up to 99% if residence time between 15 to 45 min is given along with steam temperature of 121 ℃ [52]. Some reports also mentioned the widespread use of steam autoclave treatment and reported that around 20% onsite HW disinfection is done through this process [10]. Autoclave and steam sterilization processes are categorized in wet-heating treatment of HW. Typically, autoclaves in clinical facilities are used to sterilize the recyclable medical instruments items such as paper, plastics and lab trash [56].
In dry heat treatment processes, the disinfection of iHW is done by heating it in a water/moisture free environment. Heating is done by thermal radiations, i.e., infrared and/or resistance heaters [31].
Limitations of thermal technology. HW contains significantly higher poly-composites (20-25% by weight) than municipal waste (USA municipal waste estimated plastic components = 9.4% by weight) which upon incineration could cause severe environmental and health concerns [57], [58]. Toxic emissions of polychlorinated dibenzo-p-dioxins (dioxins), polychlorinated dibenzofurans (furans) (PCDD/Fs), hydrochloric acid (HCl), sulphur dioxide (SO2), nitrogen oxides (NOx), carbon monoxide (CO), particulate matter, and heavy metals such as cadmium (Cd), mercury (Hg), and lead (Pb) are major environmental concerns [59], [60]. Domingo et al. reviewed the hazardous effects of toxic emissions from incinerators on the population living in their vicinity. It was reported that long term human exposure to such emission containing heavy metals, polychlorinated dibenzo-p-dioxins and dibenzofurans (PCDD/Fs) could significantly increase cancer and cancer mortality [61]. Mariné-Barjoan et al. reported similar results [62]. Moreover, toxic ash handling is another serious challenge. Rotary kiln incinerators could produce emissions with comparatively high particulate matters because of rotary motion of the combustion chamber.
Limitations of steam sterilization. These are following: (a) slow and uneven heating; (b) waste size reduction requirement (i.e., mechanical assistance) and (c) small handling capacities. Moreover, waste treated with autoclave may not be completely free of pathogens because of incomplete penetration of steam into the HW load and consequently micro-organisms could regrow and render post-disposal problems . Operation limitations of thermal treatments include high capital cost for incinerators. Furthermore, anatomical, radioactive, volatile organic compounds (VOCs), laboratory chemicals, and chemotherapy wastes cannot be treated by autoclave sterilization [51].
Chemical treatment methods are used principally for treating liquid iHW such as blood, body fluids, and hospital sewage [63]. However, medical waste in solid form can also be treated after reducing its size (i.e. by shredding) [64] .
Commonly used chemicals for liquid iHW are listed in Table 3 [7]. Although formaldehyde, glutaraldehyde and ethylene oxide are very effective chemicals to treat iHW, they are not taken into considerations because of their extreme toxicity and noxious emissions. Some solid iHW such as tissues, anatomical parts, and animal carcasses are treated by dissolving them in heated alkali solvents [7]. Iron(III) chloride (FeCl3) and calcium chloride (CaCl2) could be used to treat chemical oxygen demand (COD) [63]. Up to 70% COD removal can be done with 200 mg/L FeCl3. Meanwhile, this removal efficiency increased to 98% when coagulant such as aluminium sulphate (Al2(SO4)3) and/or FeCl3 were added to the effluent. CaCl2 not only reduces COD concentration but also kills microorganisms [63].
Liquid and solid iHW such as blood, other body fluids, sharps, and needles, etc., are also treated by encapsulation treatment method, in place of chemical treatment, where they are packed in rigid special containers (i.e. with concrete mixture) and later dumped at designated landfill sites [7].
Commonly used chemicals for liquid infectious healthcare waste
Acids (Peracetic acid) |
Alcohols (ethyl alcohol and isopropyl alcohol) |
Heavy metal compounds (silver nitrate, mercurochrome) |
Chlorine and chlorine compounds (Sodium hypochlorite, calcium hypochlorite) |
Iodophors (povidone-iodine) |
Hydrogen peroxide, lime solution |
Ozone gas |
Calcium oxide powder (dry inorganic powder) |
Quaternary Ammonium Compounds (didecyl dimethyl ammonium bromide, dialkyl dimethyl ammonium chloride) |
Limitations of chemical treatment method. Some resistant microorganisms such as bacterial spores and hydrophilic viruses could escape chemical treatment and render doubts on the thoroughness of the disinfection process. To ensure the efficiency of the disinfection process, high chemical concentrations are required. This could make the process unecological and uneconomical. Furthermore, relatively large retention time is required to improve the effectiveness of the process which would increase the cost.
Operation specific limitation includes size reduction requirement for solid iHW. Waste with smaller particle size would be treated effectively, however this incurs additional cost. Radioactive, hazardous chemical wastes, VOCs, and waste generated from chemotherapy cannot be treated by chemical treatment methods [65]. Moreover, toxic chemicals, aerosols, and particulate matter release during disinfection process necessitate the use of air-pollution control systems.
Irradiation of iHW is very effective, swift, and novel. Electron beams irradiation [66] and germicidal gamma radiations (i.e., radioactive cobalt-60) [67] are often employed to kill the pathogens and disinfect the iHW. These beams kill microorganisms by rapturing their cell walls and destroy the organic matters by dissociating them.
Electron-beam irradiation apparatus could have different operation schemes depending upon the manufacturer. Nutek Corporation, USA, developed electron-beam irradiation apparatus which generates electron-beams using a filament [66]. Apparatus capacity is around 180 kg/h. General HW (gHW) including plastics, glass, paper, and other trash can be treated using this apparatus. Generated electron beams are bombarded on the HW using high-voltage electric field electron accelerator. Disinfection is carried out in vacuum chamber. To make the process continuous, waste is driven by an automatic conveyor.
During the process, the temperature does not rise more than 15 ℃ while 0.0175 kWh energy is consumed per pound of HW disinfected. Due to low temperature operation condition, it produces only a few toxic emissions such as ozone gas which can be removed by catalytic destruction. Moreover, treated waste can be handled immediately after the treatment process because of low temperature. Residual radiations stops as soon as the operation shuts down [66]. Gamma rays and e-beams have the potential to penetrate through the plastic bags, making the technique effective for the disinfection process [68]. Moreover, waste can be treated within the bags.
Microwaves are also employed for the disinfection of iHW. In microwave disinfection techniques, magnetron is used to produce microwaves using electrical energy [69]. Microwaves transfer a huge number of produced waves into the waste which cause the very fast vibratory motion. To keep the molecular vibration synchronized with the bombarded waves the water molecules in the wastes vibrate. Microwaves turns moisture in the wastes into steam due to this vibrational energy [70]. The heat generation in this process destroys virus, fungus, yeast, bacteria, and spores. The steam is produced within the waste unlike autoclave operation which transfers heat from the surrounding to the waste. In microwave treatment process moisture must be present in the waste otherwise it needs to be added into the wastes. The produced steam due to microwave action acts as a disinfectant and de-contaminate iHW. Microwave treatment is not actually the irradiation technology however sometimes it is referred as one.
Vela et al. reported that microwave disinfection action works efficiently in the presence of water and at 2450 MHz [71]. Microwave treatment for iHW is novel, inexpensive, eco-friendly, and simple. In microwave technology, thermal energy could be controlled and targeted to the specific surface area of the waste using waveguide. This would reduce treatment time and make it more effective and environmentally friendly. HW is typically microwaved for 20–30 minutes and during this process the temperature could go up to 100 ℃. Unlike autoclave, in microwave treatment, no airtight operation and steam jackets are necessary. Operational cost for microwave treatment (i.e. USD 0.13/kg) is considerably lower than autoclave waste treatment (i.e. 0.14–0.33 USD/kg) [70]. Moreover, due to least toxic emissions, environmental concerns are almost negligible.
Soares et al. compared microwave and autoclave as disinfection techniques, for healthcare waste treatment using life cycle assessment (LCA) and cost analysis to evaluate the environmental impact and performance of these techniques. The results indicated that microwave has around 4 times lower environmental impact (12.64 Pt) and 10 times lower costs (0.12 USD/kg) as compared to the environmental impact (48.46 Pt) and costs (1.10 USD/kg) associated with autoclaving [72]. The results of microwave technique for HW treatment are quite encouraging and showed the potential to be a feasible sustainable alternative to current treatment technologies for iHW treatment. This technique is being applied for HW disinfection by Bertin medical waste company [73], [74].
Hospitals and health care facilities are rarely equipped with the on-site waste processing technologies which implies that HW treatment often requires off-site treatment facilities. Interim storage of hHW within the facility and off-site transportation are not environmentally friendly and viable options. Therefore, treatment at the point of generation using technologies such as microwave and other irradiations technologies are important and need more considerations.
Limitations of irradiations. High capital cost associated with the procurement of sophisticated equipment such as nuclear reactor and electron beam accelerators are the major drawbacks for gamma radiations and generation of e-beam/X-ray, respectively. Meanwhile, strict operation precaution must be practiced to main occupational safety. Maintenance cost of the equipment is also very high. One of the operation specific limitations is that gamma radiations inhibit the treatment of waste containing polyvinyl chloride (PVC), acetyl and polytetrafluoroethylene (PTFE) plastics due to their sensitivity to radiations. Although microwave technology is comparatively economical but waste containing metallic parts cannot be treated using microwave technology.
Waste-to-energy (WTE) conversion is another approach to sustainable mitigation of waste. Depending upon the state and nature of the waste, different WTE technologies could be utilized. In WTE process, energy generated could be used for any desirable purpose however, usually it is used to produce steam which then is used to produce electricity [75]. HW contains over 99% combustible constituents hence WTE is an interesting alternative to treatment and landfilling. In the US, WTE is a classified renewable energy industry under Energy Policy Act of 2005 (EPA). It is a grown industry with operating years of over three decades [76]. This testifies the potentials of WTE as an alternative and reliable industry for HW treatment.
Liquid HW is generally hazardous and contains blood, body fluids, discarded vaccines, mercury, formaldehyde, saliva, urine, etc. [77]. Treatment of liquid HW is usually done either through autoclaving or chemical disinfection. Autoclave mainly remove the pathogens. Chemical disinfection although changes the nature of the waste but usually increases the volume of the disposables. Therefore, in both of these treatments waste disposal remains the problem. WTE treatment using the anaerobic digestion process (ADP) or electrochemical fuel cell are other interesting areas for research. Conventional anaerobic digestor (AD) could be used to produce methane and other combustible gases using biological enzymatic catalyst. Meanwhile, an improved digestion technique is anaerobic membrane bioreactors which could not only reduce the capital cost but usually has higher methane production potentials [78]. WTE through electrochemical process was conducted by Acuri et al. in enzymatic fuel cell (EFC) [79]. The study highlighted the potentials of dentistry special waste such as blood and saliva to be converted into electricity. Kabbashi et al. conducted a similar study to convert waste blood into methane using anaerobic digestion [80].
Amid novel corona virus (SARS-CoV-2), researchers are rethinking the strategies to contain HW. The outspread of the virus through direct contact and respiration is well known [81], [82], [83], [84]. However, it is hypothesized that it could escape through infected human stools and hence waste-streams containing virus or its particles could potentially become a secondary infecting source [85], [86], [87], [88]. Since, waste streams from municipal, industrial and clinical sources are transported together in either urban piping networks or through canals (in comparatively low gross domestic product (GDP) countries), it is possible that inefficiently disinfected waste-streams could cause virus spread at relatively large scales [89]. Therefore, it is of enormous importance to thoroughly disinfect potential virus carrying waste-streams at their origins [90].
Hospital waste stream can be processed with biological waste treatment methods. These methods include activated sludge, biological contact oxidation, membrane bioreactor and biological aerated filters [48]. These methods often incorporate the use of micro-organisms to treat organic HW.
Mechanical methods are used to reduce the volume of disinfected HW from autoclave and other disinfection sources [65]. Mechanical destruction methods (i.e. use of shredders, grinders, and mixers) are not essentially the disinfection treatment, instead, these methods are combined with other disinfection techniques for the improvement of the overall process in terms of better mixing and distribution of heat to effectively kill harmful micro-organisms [7].
Air- and waterborne diseases associated with landfilling, and poor incineration practice of hHW and vulnerability of epidemic outbreak in case of poor management of the HW, warrant the urgent need for safe, novel, and better treatment technologies [6]. Commonly practiced medical waste treatment technologies (incineration and landfills) are not completely efficient and could produce undesirable by-products during waste handling [4]. Therefore, novel technologies such as plasma arc [91], superheated steam sterilization [56], irradiations and ozone technologies need to be developed for large scale operations to treat hHW [7]. Among the technologies, irradiation technologies are of particular interest and have the potential to provide viable alternative to existing technologies owning to their nitrogen and sulphur dioxide free emissions [67].
Sustainable assessment of technologies (SAT) is a set of methods which helps in evaluating and assessing the sustainability of a treatment method by taking into considerations its effectiveness in terms of environment friendliness, technical and economical suitability, social acceptability, and long-term operational capabilities. The method intervenes both at strategic and operational levels. Specifics of the method can be found elsewhere [92].
Makan and Fadili used the ‘preference ranking organization method for enrichment evaluations’ (PROMETHEE) to assess the sustainability of HW treatment methods [94]. Ten treatment technologies including high and low heat treatment technologies and landfill were selected for this purpose. These technologies are assessed against four major criterions i.e. environmental, financial/economic, social, and technical. There were several factors considered under these criterions. According to PROMETHEE II – complete ranking through the calculation of the net outranking flow – rotary kiln turns out to be the most sustainable treatment method. Waste landfilling got the least rating and ranked the lowest among considered technologies. Further details on PROMETHEE method could be found in the literature [93].
Rahmani et al. conducted the SAT analysis to categorize the best alternative technology for iHW generated from hospitals in Ardabil city of Iran [94]. In the study, four environmental health experts, four infection control expert and two nurses were invited to answer yes/no questionnaire for treatment technologies (shredder, chemical treatment, hydroclave, microwave, chem-clave, and central incineration) that were selected. The analysis was conducted under the set of national environmental laws and international policies set by WHO. Based on the assessment, autoclave with a shredder was reported as a best treatment technology to treat iHW while chemical method stood fifth. The selection was made after considering hospital and environmental specific conditions, and evaluating social, technical, and economic factors. Other techniques, in the order of their evaluation, were: hydroclave, autoclave, central incineration, and chemical treatment.
Similar ranking has been reported by Rafiee et al. [95]. SAT method of reaching out for best treatment technology is based on the highest standing in all aspects i.e. environmental, technical, economic, and social. However, SAT method assessment of the treatment technologies relies on the opinions of the experts. Moreover, experts from different countries or even different regions within the same country could have different opinions based on the ratios of the hHW and gHW and other such factors.
Selection of appropriate method for the treatment of HW is very important. Following factors should be considered to select sustainable treatment methods: (a) types, and volume of the HW; (b) disinfection capabilities of the process; (c) treatment site availability (on-site versus off-site); (d) availability of resources (i.e. funds, technology and human resources for waste treatment); (e ) health, environmental and safety considerations; (f) treated waste disposal requirements and (g) operation, maintenance and other regulatory costs of the treatment facility, etc. [7].
Regulation of HW at national level requires specific waste handling legislations and their enforcement. These legislations motivate public and private sector stakeholders to take actions, implement and monitor waste regulations. Typical stakeholders are, ministry of health, ministry of environment, ministry of human resource, ministry of transportation, ministry of finance, and ministry of waste management, etc. [30]. Based on the type of wastes (i.e. solid, liquid or gaseous emissions) generated in the process of HW treatment, regulations exist in all fronts for removal of the pollutants or wastes.
Flue gases from incineration processes of HW carry a number of toxic components such as dioxins, heavy metals, furans, organic compounds, etc. [7]. Due to stringent international legal limits for toxic emissions, existing medical waste incinerators need retrofitting of emission control equipment to curb flue gases and meet the international permissible limits [96], [97]. Although air-pollution control devices could significantly reduce the toxic emissions, the overall removal efficiencies are inadequate which suggests that there is a need for better alternatives such as microwave and other irradiation technologies [98].
Moreover, treatment of the flue gases includes air pollution control for removal of fly ash (i.e. particulate filters), halides and oxides (i.e., scrubbing with alkaline substance). Vavva et al. discussed the treatment method of fly ash produced from incineration of HW [50]. Electrostatic forces and physical filters such as electrostatic precipitators (ESPs) and fabric filters (FFs) respectively, are used for removal of heavy metals. Heavy metals removal efficiency by ESPs is remarkable and remove arsenic (As), Barium (Ba), Cadmium (Cd), Chromium (Cr), Cobalt (Co), Copper (Cu), Manganese (Mn), and Vanadium (V) to over 99 % [99]. Dry and semi-dry scrubbing processes with calcium-based sorbents such as limestone (CaCO3), lime (CaO), or hydrated lime (Ca(OH)2) are used to solidify acids such as HCl and hydrofluoric acid (HF) and acid precursors such as SO2 [100]. NOx produced during the combustion could be reduced by controlling the excess air or reducing the combustion temperature [100].
Selective catalytic reduction (SCR) is a more efficient method for reducing NOx (i.e. it reduces up to 90 % NOx) [101]. Selective non-catalytic reduction (SNCR) in the presence of oxygen by reaction with ammonia (NH3) and/or urea (CO(NH2)2) are used to convert NOx to N2 [101]. Furans and dioxins producing reactions during HW incineration could be controlled by adjusting reaction condition (i.e. temperature) [4]. Ash and sludge are generated in the incineration of solid HW and clinical wastewater treatment, respectively, which are considered as hazardous. Fly ash needs special attentions if the incineration conditions are not managed properly. Hence, to avoid hazards handling of treated leftovers such as dumping in designated landfill sites must be practiced carefully [7].
Moreover, ash could be used in different applications other than sending it to landfills if it is produced from gHW. Deng et al. report production of ceramics from ash [102]. Treatment of HW fly ash from incinerators and removing contaminants such as heavy metals from it could enable applications in mortar mixtures [103]. Zaldívar et al. [104] and Chuang et al. [105] proposed applications in calcium aluminate cement (CAC) mortars (as insoluble carbonates) and lightweight aggregates, respectively. Nevertheless, finding further applications of fly ash and its better management requires more research.
Handling and disposal of the treated waste is another huge challenge because of the various stages involved in the step, i.e., from suitable selection of the landfills to post-closure monitoring and other associated complexities. Disposal of the treated waste must be carried out under safe environmental practices and enacted laws [106]. The waste that is discarded in landfills should be monitored subsequently [107].
Thorough and comprehensive measures must be taken by hospitals and clinics in HW management and treatment methods. Properly segregated hHW could reduce the cost significantly since only about 10–25 % of HW is infectious. Clinics and hospitals must promote excellent segregation practices, improve working skills of the staff by education, training, and incentives. An efficient medical waste management coupled with new technologies such as autoclave with shredder could yield better environmentally friendly and cost-effective results. In the future, it is expected that conventional incinerators running on non-renewable energy sources, will be replaced with modern incinerators running on sustainable and renewable energy sources such as biofuels.
Meanwhile, efforts should be employed to incorporate SAT method in the evaluation of the sustainability of the available methods. At advanced scale, SAT assessment might also be done on the assessment of two or more HW treatment methods together. This would evaluate the synergistic effects and might improve the overall effectiveness of the treatment technologies. SAT methods to this point revealed that in often cases, autoclave assisted with shredder could be a better alternative for the treatment of hHW. Furthermore, efforts should be directed towards developing more reliable ways of assessing the sustainability of the treatment techniques. We anticipate, this contribution could play an important role in proper management of the HW and keeping public as well as medical staff, nurses, doctors, and hospitals safe from prevailing pandemic diseases such as coronavirus (COVID-19). This work is dedicated to all the medical staff, nurses and doctors who work tirelessly around the globe in keeping the public safe from spread of epidemic diseases.
The authors would like to thank American University of Sharjah for the support of Faculty Research Grant (FRG19-S-E102 Project) by OCRO.
Abbreviations |
|
Ca(OH)2 |
Hydrated Lime |
CO(NH2)2 |
Urea |
AD |
Anaerobic Digester |
ADP |
Anaerobic Digestion Process |
Al2(SO4)3 |
Aluminium Sulphate |
As |
Arsenic |
Ba |
Barium |
CAC |
Calcium Aluminate Cement |
CaCl2 |
Calcium Chloride |
CaCO3 |
Limestone |
CaO |
Lime |
Cd |
Cadmium |
Cl2 |
Chlorine |
ClO2 |
Chlorine Dioxide |
CO |
Carbon Monoxide |
Co |
Cobalt |
COD |
Chemical Oxygen Demand |
COVID-19 |
Corona Virus |
Cr |
Chromium |
Cu |
Copper |
Dioxins |
Polychlorinated Dibenzo-p-dioxins |
e-beam |
Electron Beam |
EFC |
Enzymatic Fuel Cell |
ENT |
Ear, Nose, and Throat |
EPA |
Energy Policy Act |
ESPs |
Electrostatic Precipitators |
FeCl3 |
Iron(III) Chloride |
FFs |
Fabric Filters |
Furans |
Polychlorinated Dibenzofurans |
GDP |
Gross Domestic Product |
gHW |
General Healthcare Waste |
HBV |
Hepatitis B Virus |
HCl |
Hydrochloric Acid |
HF |
Hydrofluoric Acid |
Hg |
Mercury |
hHW |
Hazardous Healthcare Waste |
HIV |
Human Immunodeficiency Viruses |
HRSG |
Heat Recovery System |
HW |
Healthcare Waste |
ICUs |
Intensive Care Units |
iHW |
Infectious Health Waste |
MHW |
Management of healthcare waste |
Mn |
Manganese |
N2 |
Nitrogen |
NaClO |
Sodium Hypochlorite |
NH3 |
Ammonia |
NOx |
Nitrogen Oxides |
NTUH |
National Taiwan University Hospital |
OTs |
Operation Theatres |
Pb |
Lead |
PCDD/Fs |
Polychlorinated Dibenzo-p-dioxins and Polychlorinated Dibenzofurans |
PROMETHEE |
Preference Ranking Organization Method for Enrichment Evaluations |
PTFE |
Acetyl and Polytetrafluoroethylene |
PVC |
Polyvinyl Chloride |
SARS-CoV-2 |
Severe Acute Respiratory Syndrome Coronavirus 2 |
SAT |
Sustainable Assessment of Technologies |
SCR |
Selective Catalytic Reduction |
SMHW |
Sustainable Management of Healthcare Waste |
SNCR |
Selective Non-Catalytic Reduction |
SO2 |
Sulphur Dioxide |
USD |
United States Dollar |
UV |
Ultraviolet |
V |
Vanadium |
VOCs |
Volatile Organic Compounds |
WHO |
World Health Organization |
WTE |
Waste-to-energy |
Hospital waste management in El-Beheira Governorate, Egypt ,Journal of Environmental Management , Vol. 91 (3),pp 618-629 , 2010, https://doi.org/https://doi.org/10.1016/j.jenvman.2009.08.012
, Medical waste management and control ,Journal of Environmental Protection , Vol. 3 (12),pp 1625 , 2012
, A reverse supply chain for medical waste: A case study in Babol healthcare sector ,Waste Management , Vol. 113 ,pp 197-209 , 2020, https://doi.org/https://doi.org/10.1016/j.wasman.2020.05.052
, Medical waste management – A review ,Journal of Environmental Management , Vol. 163 ,pp 98-108 , 2015, https://doi.org/https://doi.org/10.1016/j.jenvman.2015.08.013
, Healthcare waste management: Current practices in selected healthcare facilities, Botswana ,Waste Management , Vol. 28 (1),pp 226-233 , 2008, https://doi.org/https://doi.org/10.1016/j.wasman.2006.12.019
, Safe and sustainable waste management of self care products ,bmj , Vol. 365 , 2019
, - , , Safe management of wastes from health-care activities, 2014
Characteristics of healthcare wastes ,Waste Management , Vol. 28 (7),pp 1219-1226 , 2008, https://doi.org/https://doi.org/10.1016/j.wasman.2007.04.010
, - Healthcare waste Institute, , https://cdn.ymaws.com/wasterecycling.org/resource/resmgr/hwi_minutes/HWI_COVID-2019_FAQs.pdf
Medical Waste Disposal ,Air & Waste , Vol. 44 (10),pp 1176-1179 , 1994, https://doi.org/10.1080/10473289.1994.10467311
, - World Health Organization, Status of Health-Care Waste Management in Selected Countries of the Western Pacific Region, , https://apps.who.int/iris/handle/10665/208230
Physical and chemical composition of hospital waste ,Infection Control & Hospital Epidemiology , Vol. 14 (3),pp 145-150 , 1993
, Heat recovery from thermal treatment of medical waste ,Energy , Vol. 90 ,pp 1721-1732 , 2015, https://doi.org/https://doi.org/10.1016/j.energy.2015.06.124
, Medical waste treatment and electricity generation using pyrolyzer-rankine cycle for specialty hospitals in Quezon City, Philippines ,E&ES , Vol. 463 (1),pp 12180 , 2020
, - World Health Orginzation, Health Care Waste,, , https://www.who.int/news-room/fact-sheets/detail/health-care-waste
Medical wastes management in the south of Brazil ,Waste Management , Vol. 25 (6),pp 600-605 , 2005, https://doi.org/https://doi.org/10.1016/j.wasman.2004.03.002
, Health care waste management: a neglected and growing public health problem worldwide ,Tropical Medicine & International Health , Vol. 14 (11),pp 1414-1417 , 2009
, - The New York Times, Ebola Outbreak in Congo Hits Milestone, and Death Toll Rises, , https://www.nytimes.com/2019/08/30/world/africa/congo-ebola-deaths.html
- Worldometer, Coronavirus Cases, , https://www.worldometers.info/coronavirus/
Assessment of medical wastes management practice: A case study of the northern part of Jordan ,Waste Management , Vol. 27 (6),pp 746-759 , 2007, https://doi.org/https://doi.org/10.1016/j.wasman.2006.03.004
, Repercussions of COVID-19 pandemic on municipal solid waste management: Challenges and opportunities ,Science of The Total Environment , Vol. 743 ,pp 140693 , 2020, https://doi.org/https://doi.org/10.1016/j.scitotenv.2020.140693
, Minimization of spreading of SARS-CoV-2 via household waste produced by subjects affected by COVID-19 or in quarantine ,Science of The Total Environment , Vol. 743 ,pp 140803 , 2020, https://doi.org/https://doi.org/10.1016/j.scitotenv.2020.140803
, Disinfection technology of hospital wastes and wastewater: Suggestions for disinfection strategy during coronavirus Disease 2019 (COVID-19) pandemic in China ,Environmental Pollution , Vol. 262 ,pp 114665 , 2020, https://doi.org/https://doi.org/10.1016/j.envpol.2020.114665
, Can the human coronavirus epidemic also spread through solid waste? ,Waste Management & Research , Vol. 38 (5),pp 485-486 , 2020
, Aerosol and surface stability of SARS-CoV-2 as compared with SARS-CoV-1 ,New England Journal of Medicine , Vol. 382 (16),pp 1564-1567 , 2020
, - A vision for primary health care in the 21st century, Technical series on primary healthcare , 2018
- Improving health and wellbeing: new advances in medical care, , https://stfc.ukri.org/files/science-publications/improving-health-and-wellbeing/
The Green Print: Advancement of Environmental Sustainability in Healthcare ,Resources, Conservation and Recycling , Vol. 161 ,pp 104882 , 2020
, Life cycle environmental emissions and health damages from the Canadian healthcare system: an economic-environmental-epidemiological analysis ,PLoS medicine , Vol. 15 (7),pp e1002623 , 2018
, - Technical Brief: Sustainable Health Care Waste Management , 2020
- International Committee of the Red Cross, Medical Waste Management, , https://www.icrc.org/en/publication/4032-medical-waste-management
Medical Waste Management: Where Does the Solid Waste Go? ,Laboratory Medicine , Vol. 31 (3),pp 141-145 , 2000
, Medical Waste-Sorting and Management Practices in Five Hospitals in Ghana ,Journal of environmental and public health , Vol. 2020 , 2020
, - United Kindom without Incineration, Evaluation of the Climate Change Impacts of Waste Incineration in the United Kingdom, , https://ukwin.org.uk/files/pdf/UKWIN-2018-Incineration-Climate-Change-Report.pdf
Activism for health: green surgery ,BMJ: British Medical Journal (Online) , Vol. 368 , 2020
, Reducing Waste and Increasing Sustainability in Health Care Settings ,AJN The American Journal of Nursing , Vol. 120 (4),pp 45-48 , 2020
, Efficiency improvement for medical waste management ,Handbook of Clean Energy Systems ,pp 1-11 , 2015
, Boost for sustainable healthcare , 2020
, Carbon footprint of the global pharmaceutical industry and relative impact of its major players ,Journal of Cleaner Production , Vol. 214 ,pp 185-194 , 2019
, Environmental sustainability and waste within the dental practice ,British dental journal , Vol. 226 (8),pp 611-618 , 2019
, Waste production from phacoemulsification surgery ,Journal of Cataract & Refractive Surgery , Vol. 46 (2),pp 215-221 , 2020
, Mass needle stick injury in children from the Western cape ,Journal of tropical pediatrics , Vol. 52 (3),pp 192-196 , 2006
, On-site or off-site treatment of medical waste: a challenge ,Journal of Environmental Health Science and Engineering , Vol. 12 (1),pp 68 , 2014
, Medical waste collection considering transportation and storage risk ,Computers & Operations Research , Vol. 120 ,pp 104966 , 2020, https://doi.org/https://doi.org/10.1016/j.cor.2020.104966
, Disinfection of hospital wastewater by continuous ozonization ,Journal of Environmental Science and Health, Part A , Vol. 38 (12),pp 2895-2908 , 2003
, Human enteric viruses in a wastewater treatment plant: evaluation of activated sludge combined with UV disinfection process reveals different removal performances for viruses with different features ,Letters in applied microbiology , Vol. 66 (3),pp 215-221 , 2018
, Research on Simple Disinfection System for Medical Wastewater of Township Hospital. ,Asian Journal of Chemistry , Vol. 26 (11), 2014
, Application progress of hospital wastewater treatment methods ,Occupational Health , Vol. 26 ,pp 1180-1182 , 2010
, - , Comparison study on hospital wastewater disinfection technology, Advanced Materials Research, 2014
Chemical Stabilization of Fly Ash from Medical Waste Incinerators ,Environmental Processes ,pp 1-21 , 2020
, Alternatives for treatment and disposal cost reduction of regulated medical wastes ,Waste Management , Vol. 24 (2),pp 143-151 , 2004, https://doi.org/https://doi.org/10.1016/j.wasman.2003.10.008
, Medical waste management/incineration ,Journal of Hazardous Materials , Vol. 48 (1-3),pp 1-30 , 1996
, - Environmental Protection Agency, Locating and Estimating Air Emissions from Sources of Dioxins and Furans, , https://www3.epa.gov/ttnchie1/le/dioxin.pdf
Treatment of medical solid waste using an Air Flow controlled incinerator ,Polish Journal of Chemical Technology , Vol. 22 (1),pp 29-34 , 2020
, - Environmental Protection Agency, Medical waste incineration, , https://www3.epa.gov/ttnchie1/ap42/ch02/final/c02s03.pdf
Treatment of clinical solid waste using a steam autoclave as a possible alternative technology to incineration ,International journal of environmental research and public health , Vol. 9 (3),pp 855-867 , 2012
, Analyses of the recycling potential of medical plastic wastes ,Waste Management , Vol. 22 (5),pp 461-470 , 2002, https://doi.org/https://doi.org/10.1016/S0956-053X(02)00006-5
, Composition of municipal solid waste in the United States and implications for carbon sequestration and methane yield ,Journal of Environmental Engineering , Vol. 135 (10),pp 901-909 , 2009
, Heavy metal pollution from medical waste incineration at Islamabad and Rawalpindi, Pakistan ,Microchemical Journal , Vol. 90 (1),pp 77-81 , 2008, https://doi.org/https://doi.org/10.1016/j.microc.2008.03.010
, Dioxins from medical waste incineration: normal operation and transient conditions ,Waste Management & Research , Vol. 33 (7),pp 644-651 , 2015
, Adverse health effects for populations living near waste incinerators with special attention to hazardous waste incinerators. A review of the scientific literature ,Environmental Research , Vol. 187 ,pp 109631 , 2020, https://doi.org/https://doi.org/10.1016/j.envres.2020.109631
, Cancer Incidence in the vicinity of a waste incineration plant in the Nice area between 2005 and 2014 ,Environmental Research ,pp 109681 , 2020
, Occurrence, sources and conventional treatment techniques for various antibiotics present in hospital wastewaters: A critical review ,TrAC Trends in Analytical Chemistry , Vol. 129 ,pp 115921 , 2020, https://doi.org/https://doi.org/10.1016/j.trac.2020.115921
, - Pegex, Regulated Medical Waste Treatment Wethods, , https://www.hazardouswasteexperts.com/regulated-medical-waste-treatment-methods/
Alternatives for the treatment and disposal of healthcare wastes in developing countries ,Waste Management , Vol. 25 (6),pp 626-637 , 2005
, Improving medical waste disposal ,Mechanical Engineering-CIME , Vol. 116 (5),pp 56-60 , 1994
, Radiation technologies for waste treatment: a global perspective ,IAEA Bulletin , Vol. 36 (1),pp 11-15 , 1994
, - Malsparo, Medical Waste Management, Collection, Storage, and Treatment of Medical Waste, , https://www.malsparo.com/
- Health Care without Harm, Non-Incineration Medical Waste Treatment Technologies in Europe, , https://www.env-health.org/IMG/pdf/altech_Europe_updated_version_10_12_2004.pdf
- Omania Health, New horizons for medical waste treatment technology, , https://insights.omnia-health.com/hospital-management/new-horizons-medical-waste-treatment-technology
Mechanism of lethal action of 2,450-MHz radiation on microorganisms. ,Applied and Environmental Microbiology , Vol. 37 (3),pp 550-553 , 1979
, Applications of life cycle assessment and cost analysis in health care waste management ,Waste management , Vol. 33 (1),pp 175-183 , 2013
, - Microwave medical waste treatment, , https://www.bertin-medical-waste.com/microwave-medical-waste-treatment/
- Laboratory waste management, , https://www.bertin-medical-waste.com/laboratory-waste-management/
- Biomass explained, Waste-to-energy (Municipal Solid Waste), , https://www.eia.gov/energyexplained/biomass/waste-to-energy.php
- , , Woodhead Publishing Series in Energy, 2013
Liquid biomedical waste management: An emerging concern for physicians ,Muller Journal of Medical Sciences and Research , Vol. 4 (2),pp 99 , 2013
, - Waste to Energy, , https://www.energy.gov/eere/bioenergy/waste-energy
Medical waste to energy: experimental study ,ORAL & implantology , Vol. 6 (4),pp 83-88 , 2014
, Methane Production from Biomedical Waste (Blood) ,International Journal of Energy and Environmental Engineering , Vol. 12 (11),pp 657-664 , 2018
, Early transmission dynamics in Wuhan, China, of novel coronavirus–infected pneumonia ,New England Journal of Medicine , 2020
, Epidemiological and clinical characteristics of 99 cases of 2019 novel coronavirus pneumonia in Wuhan, China: a descriptive study ,The Lancet , Vol. 395 (10223),pp 507-513 , 2020, https://doi.org/https://doi.org/10.1016/S0140-6736(20)30211-7
, A familial cluster of pneumonia associated with the 2019 novel coronavirus indicating person-to-person transmission: a study of a family cluster ,The Lancet , Vol. 395 (10223),pp 514-523 , 2020, https://doi.org/https://doi.org/10.1016/S0140-6736(20)30154-9
, Importation and human-to-human transmission of a novel coronavirus in Vietnam ,New England Journal of Medicine , Vol. 382 (9),pp 872-874 , 2020
, The digestive system is a potential route of 2019-nCov infection: a bioinformatics analysis based on single-cell transcriptomes ,BioRxiv , 2020
, First case of 2019 novel coronavirus in the United States ,New England Journal of Medicine , 2020
, Persistence and clearance of viral RNA in 2019 novel coronavirus disease rehabilitation patients ,Chinese medical journal , 2020
, Prolonged presence of SARS-CoV-2 in feces of pediatric patients during the convalescent phase ,medRxiv , 2020
, SARS-CoV-2 spillover into hospital outdoor environments ,medRxiv , 2020
, Potential spreading risks and disinfection challenges of medical wastewater by the presence of Severe Acute Respiratory Syndrome Coronavirus 2 (SARS-CoV-2) viral RNA in septic tanks of Fangcang Hospital ,Science of The Total Environment , Vol. 741 ,pp 140445 , 2020, https://doi.org/https://doi.org/10.1016/j.scitotenv.2020.140445
, Safe disposal and effective destruction of clinical wastes ,Journal of Hospital Infection , Vol. 60 (4),pp 295-297 , 2005, https://doi.org/https://doi.org/10.1016/j.jhin.2005.01.029
, Compendium of technologies for treatment/destruction of healthcare waste , 2012
, PROMETHEE: A comprehensive literature review on methodologies and applications ,European Journal of Operational Research , Vol. 200 (1),pp 198-215 , 2010, https://doi.org/https://doi.org/10.1016/j.ejor.2009.01.021
, Assessment and selection of the best treatment alternative for infectious waste by Sustainability Assessment of Technologies (SAT) methodology ,Journal of the Air & Waste Management Association , Vol. 70 (3),pp 333-340 , 2020
, Assessment and selection of the best treatment alternative for infectious waste by modified Sustainability Assessment of Technologies methodology ,Journal of Environmental Health Science and Engineering , Vol. 14 (1),pp 10 , 2016
, Application of best available technologies on medical wastes disposal/treatment in China (with case study) ,Procedia Environmental Sciences , Vol. 16 ,pp 257-265 , 2012
, Dioxin emission factors for the incineration of different medical waste types ,Archives of Environmental Contamination and Toxicology , Vol. 44 (4),pp 460-466 , 2003
, Emission of polycyclic aromatic hydrocarbons from medical waste incinerators ,Atmospheric Environment , Vol. 36 (5),pp 781-790 , 2002
, - , , 2019
Air pollution control in municipal solid waste incinerators ,The impact of air pollution on health, economy, environment and agricultural sources ,pp 331-358 , 2011
, - , , Advances in Ultra-Low Emission Control Technologies for Coal-Fired Power Plants, 2019
Sustainable utilization of municipal solid waste incineration fly ash for ceramic bricks with eco-friendly biosafety ,Materials Today Sustainability , Vol. 1-2 ,pp 32-38 , 2018, https://doi.org/https://doi.org/10.1016/j.mtsust.2018.11.002
, Recycling of pre-treated medical waste fly ash in mortar mixtures ,Journal of Material Cycles and Waste Management , Vol. 22 (1),pp 207-220 , 2020
, Effects of the addition of inertized MSW fly ash on calcium aluminate cement mortars ,Construction and Building Materials , Vol. 157 ,pp 1106-1116 , 2017, https://doi.org/https://doi.org/10.1016/j.conbuildmat.2017.09.189
, Reuse of bottom ash and fly ash from mechanical-bed and fluidized-bed municipal incinerators in manufacturing lightweight aggregates ,Ceramics International , Vol. 44 (11),pp 12691-12696 , 2018, https://doi.org/https://doi.org/10.1016/j.ceramint.2018.04.070
, DC geoelectrical methods applied to landfill investigation: case studies from Greece ,first break , Vol. 32 (8), 2014
, - , , Modelling trends in solid and hazardous waste management, 2017