Orange II, also known as 2-Naphthol Orange and Acid Orange 7, is an important example of azo compound dye. It is widely used in wool dyeing and as a colouring agent in printing. Its discharge in water bodies after the industrial operations causes severe damage to aquatic environments and provokes health hazards.
Among the different azo dyes, Orange II was chosen for this study for many reasons. It could be easily prepared and purified in our laboratory. Its concentration in water can be easily measured by spectrophotometry because it has an absorbance maximum within the visible light spectrum. In the darkness, it would not be oxidized or reduced by other components in the solution. In addition to this, this azo dye is not a pH indicator, so the hydronium concentration changes, which may happen in the discoloration process, will not shift its absorbance peak during the kinetics experiments.
Due to its high stability, Orange II is not easily biodegradable, and its discoloration requires special methods, as was reported by Daneshvar et al. [1]. The removal of organic pollutants from wastewater and effluents by discoloration processes using different photocatalysts was thoroughly studied by Khan et al. [2]. These processes would combine the adsorption of azo dye over the catalyst and its oxidative degradation in a photoreaction. Appropriate light irradiation over semiconductor oxides results in electron-hole pairs generation, which starts producing hydroxide radicals (•OH). Nishio et al. studied that these reactive agents can oxidize azo compounds to ammonium sulfate, carbon dioxide, and water without generating any solid waste [3].
Semiconductor metal oxides, such as titanium dioxide (TiO2) and zinc oxide (ZnO), can act as good catalysts for azo dye oxidation due to their chemical stability, low toxicity, and high photosensitivity. Daneshvar et al. reported that ZnO has a higher photocatalytic efficiency compared with TiO2 [4]. The effect of the preparation method on the catalytic activity of both semiconductor oxides was studied by Gonçalves et al. [5].
The use of zinc oxide nanoparticles as a catalyst of oxidation processes under sunlight exposition has been thoroughly studied, as it is reviewed by Ong et al. [6]. It is known that ZnO is an n-type semiconductor with a wideband gap of 3.37 eV and a large exciton binding energy of 60 meV [7].
ZnO, as a photocatalyst, has been tested in nanopowder aqueous suspension by Daneshvar et al. [1]. Marto et al. studied its activity supported on ceramic tiles [8]. Zinc oxide thin films were examined by Ali et al. [9]. Danwittayakul et al. used it in nanorods [10]. ZnO nanorods deposited onto borosilicate glass were studied by Sáenz-Trevizo et al. [11]. The activity of a zinc oxide microsphere suspension was measured by Wang et al. [12]. Whereas ceramic-supported ZnO microspheres were tested by Liu et al. [13].
The efficiency of zinc oxide as a catalyst depends on several factors. The effect of preparation methods was reported by Balcha et al. [14]. Sharma et al. examined the influence of annealing temperature [15]. The relation between ZnO activity and its particle size and morphology was studied by Kumar et al. [16].
Magnetic composites as catalyst support have been earlier prepared as it was informed in an article by Govan and Gun’ko [17]. Notwithstanding, along with all these supported catalysts, it seems necessary to protect the magnetic core to retain magnetic properties. For that reason, the core is coated with some non-magnetic relatively inert shell such as silica, as was reported by Faraji et al. [18]. The silica shell is straightforward to functionalize and suitable for binding various catalytic species, including transition metal complexes.
Catalyst remotion from water would be a desirable advantage, as Wu et al. reported [19]. Bacchetta et al. proved that ZnO nanoparticles could be harmful to different aquatic organisms [20]. Chevallet et al. studied the toxicity of zinc oxide on liver cells [21]. Whereas Kononenko et al. tested it on kidney cells [22].
The present work aims to explore the discoloration process of Orange II photocatalyzed by ZnO nanoparticles with two different strontium ferrite/zinc oxide composites prepared by a coprecipitation route. These magnetic phases were hexagonal ferrites (strontium ferrite and neodymium-cobalt strontium ferrite). We report a one-step method for synthesizing the composite Sr ferrite/ZnO as the magnetic phase is a hard ferrite with good chemical stability.
Spinel ferrites, soft ferromagnetic materials, have also been tested in wastewater treatment, as was reported by Kefeni et al. [23]. Among these materials, zinc ferrite (ZnFe2O4) was studied by Cai et al. as a photocatalyst of dyes degradation in the presence of persulfate [24]. The mechanism of this process has been studied by Zhu et al. [25]. Tadjarodi et al. tested the photocatalytic activity of zinc ferrite encapsulated in clay as a nanocomposite [26]. The use of a ZnO/Zn ferrite composite as a catalyst has been published by Coelho et al. [27]. In comparison, Mandal and Natarajan studied the catalytic properties of a composite of ZnO and a Zn-Fe mixed oxide [28]. Zinc oxide composite catalysts with hematite (Fe2O3) or with elemental iron were tested by Maya-Treviño et al. [29]. All these composite catalysts were prepared with soft ferrites. Notwithstanding, the photocatalyzed discoloration of an azo dye by ZnO supported on hard ferrite particles, as it is informed in this work, would constitute a novel study. To the best of our knowledge, no similar system has been reported yet.
Both phases of the composites were prepared using wet methods in our laboratory.
Strontium hexaferrite particles were synthesized by a self-combustion method, and the hydrated ZnO was precipitated on the magnetic support.
Samples of strontium hexaferrite (labelled as M00, with nominal composition SrFe11O19) and neodymium-cobalt substituted strontium hexaferrite (labelled M20, nominal composition Sr0.8Nd0.2Co0.2Fe10.8O19) were synthesized using a self-combustion method reported by Herme et al. [30]. All the reactants, iron (III) nitrate nonahydrate (Fe(NO3)3·9H2O), strontium carbonate (SrCO3), neodymium oxide (Nd2O3), and cobalt (II) acetate tetrahydrate (Co(CH3CO2)2·4H2O), were analytical grade.
A certain mass of each ferrite, suspended in sodium citrate solution 0.50 mol/L, was stirred with an ultrasonicator for 2 h at 60 °C to disperse any cluster of particles. By this treatment, the surface of the particles was modified to improve zinc hydroxide (Zn(OH)2) coating. The two samples of modified ferrite particles were centrifuged and washed with acetone.
10 mL of a zinc acetate dihydrate (Zn(CH3CO2)2·2H2O) 0.55 mol/L solution and 10 mL of ammonium carbonate ((NH4)2CO3) 0.80 mol/L were slowly and simultaneously added dropwise over 20 mL of surface-modified ferrites suspensions at 25 °C. This technique was adapted from a procedure previously published by Hong et al. to obtain a similar material [31].
Since magnetic stirring would cause aggregation of ferrite particles, the suspensions were mixed with nitrogen gas flow during the precipitation. The CO2 released in the reaction also contributed to the stirring.
Due to the ammonium carbonate addition, the change in the pH caused the hydrated zinc oxide (ZnO∙xH2O) to precipitate over the modified surface of the ferrite particles. The composites were centrifuged and washed with distilled water, diluted ammonia and absolute ethanol, and dried in a vacuum furnace. Then, they were heated for 4 h at 350 °C, to dehydrate the zinc oxide and gently milled.
These precipitations were done with different mass ratios between ferrite particles (either M00 or M20) and zinc acetate solution to prepare various samples. The names and nominal compositions of these samples are presented in Table 1. Finally, only two composites, labelled Z00 (SrFe11O19/ZnO) and Z20 (Sr0.8Nd0.2Co0.2Fe10.8O19/ZnO), were chosen to carry out the kinetics experiments. All these samples were characterized by X-ray diffraction (XRD), scanning electron microscopy (SEM), and vibrating sample magnetometry (VSM).
Nominal compositions of strontium ferrite/zinc oxide composites
Composite | Ferrite and its mass [mg/100 mg ZnO] | |
M00 | SrFe11O19 | - |
Z0L | M00 | 3 |
Z00 | M00 | 45 |
Z01 | M00 | 90 |
Z02 | M00 | 178 |
Z20 | M20 | 45 |
Catalysts particles were suspended in distilled water. Orange II discoloration was scanned by visible light spectrophotometry. All experiments were performed at pH = 7.2.
Orange II (Figure 1) was prepared as its sodium salt by a coupling reaction between beta-naphtol (β-C10H7OH) and sulfanilic acid (para-aminebenzenesulfonic acid) in a sodium nitrite (NaNO2) solution. Dye was recrystallized in ethanol-water, and 100 mg/L solution was made using distilled water.
Kinetics measurements were carried out with composites or ZnO suspensions. Control tests were performed on single ferrite particles or without light exposition. The results of the experiments are shown in Table 2. Samples for discoloration were prepared by mixing 4.00 mL of catalysts suspensions in distilled water (final conc. 1000 mg/L) and 1.00 mL dye solution (final conc. 20 mg/L).
Hydrated zinc oxide reacts either with hydronium or hydroxide ions [32]. Therefore, the pH is stabilized by combined acid-base/solubility equilibria of ZnO in the suspensions [33], which are presented here in equations (1)-(3) as follows:
(1)
(2)
(3)
The azo dye Orange II as its sodium salt [34]
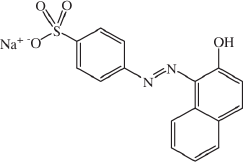
Reactor tubes content in each discoloration experiment
Experiment # | Concentration [mg/L] | Light exposition | ||
1 | - | 0 | yes | |
2 | - | 0 | no | |
3 | M20 | 1000 | yes | |
4 | ZnO | 1000 | yes | |
5 | ZnO | 1000 | no | |
6 | Z00 | 100 | yes | |
7 | Z00 | 250 | yes | |
8 | Z00 | 1000 | yes | |
9 | Z20 | 1000 | yes |
Samples were placed into glass test tubes (1.5 cm diameter) of high optic quality and attached around the external surface of a borosilicate glass flask (15.0 cm diameter) in a carrousel-like array. A 160 W mixing lamp Philips ML was placed at the center of the flask, and the whole set was put into a thermostat water bath which could be mechanically stirred. The array of reactor tubes can be seen in Figure 2.
Images of the carrousel array of tubes around the flask and the mixing lamp (a-d)
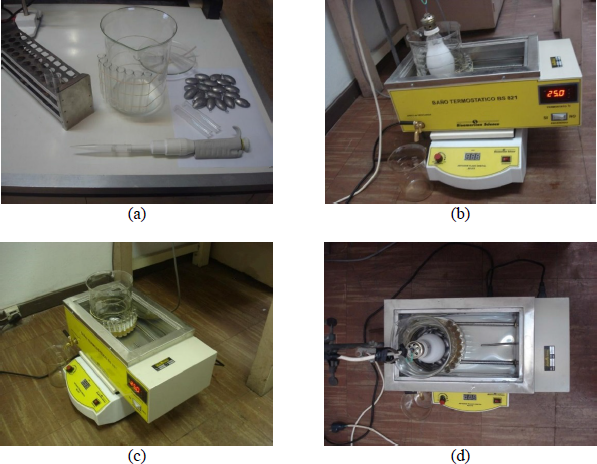
Sunlight was emulated with this mixing lamp. Its emission combines a continuous spectrum of an incandescent tungsten filament and a line spectrum from a high-pressure mercury source. During the kinetics experiments, the irradiance calculated value at the center of each test tube was 1900 W/m2 approximately.
All experiments were performed at 25 °C. Samples were homogenized in darkness by mechanical stirring and, immediately, all the test tubes were attached to the flask. At t = 0, the lamp was switched on; the first tube content was extracted with a syringe and centrifuged for 1 min at approx. 800 g (8000 m/s2). The supernatant solution was put into a quartz cell; its absorbance was measured in a Shimadzu UV-2401 spectrophotometer at its maximum absorbance value within the visible spectrum, λmax = 485 nm (reference: distilled water). This procedure was repeated with the next test tube after fixed intervals up to 240 min. Dye concentration values in each tube were calculated as a function of time using Lambert-Beer’s law [35].
Composites were structurally and magnetically characterized. Discoloration kinetics was studied by spectrophotometry.
XRD, SEM, and VSM were used to study the properties of the catalyst composites.
The XRD patterns of all the composites, measured in a Philips diffractometer (using CuKα radiation), are shown in Figure 3. The profiles confirm the hexagonal phase of Sr ferrite and the main peaks assigned to ZnO.
X-ray diffractograms of M00 (Sr hexaferrite) and all the composites
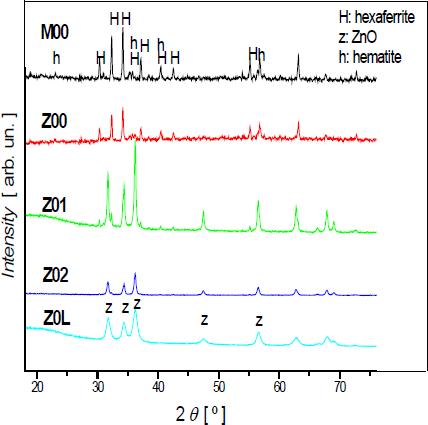
Strontium hexaferrite, Z00 composite (SrFe11O19/ZnO), and zinc oxide nanoparticles were structurally characterized by SEM in a Zeiss DSM 982 GEMINI microscope. Figure 4 shows the SEM images. The hexagonal ferrite looks like platelets (average particle size 230 nm), while the ZnO nanoparticles are spherical and well dispersed (average particle size 60 nm).
SEM images, magnified × 90000 M00 Sr hexaferrite (a); Z00 composite (b); and ZnO nanoparticles (c)
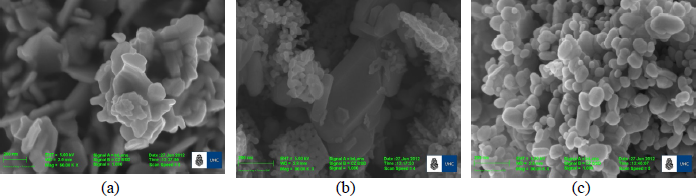
Magnetization versus magnetic field (M-H) loops of the M00/ZnO composites were measured with a Lakeshore 7300 VSM, and their main magnetic properties were calculated (Table 3). Magnetic results of M20/ZnO composite (Z20) are not presented.
Magnetic properties values of the composites
Sample | Saturation magnetization, MS [Am2/kg] | Coercive field, HC [A/m] |
M00 ferrite | 97 | 62.0 |
Z0L | 0.7 | 65.8 |
Z00 | 7.2 | 53.5 |
Z01 | 15.1 | 52.6 |
Z02 | 76 | 43.2 |
Since magnetization of a two-component system depends on component masses [36] and zinc oxide is a diamagnetic material, it is possible to ponderate mass composition from MS results. In this way, for the Z00 composite, a ZnO mass fraction of 0.926 was calculated. The enhancement in HC for the Z0L composite is related to the high dilution of the ferrite particles into a non-magnetic material. These composites show good magnetic properties after the one-step preparation method.
Preliminary experiments to explore/discard Orange II adsorption on catalyst were performed. The absorbance of azo dye solutions with and without ZnO nanopowder was measured in darkness. Besides, Orange II stability was tested with M20 ferrite (without ZnO coating) suspended particles. Results are presented in Figure 5. All these profiles show a very small negative slope, so it can be concluded that dye adsorption on ZnO particles or the magnetic support is negligible (< 0.3%). These experiments show that neither ZnO nanoparticles nor Sr ferrite particles diminish Orange II concentration by adsorption processes.
Orange II adsorption on ZnO or M20 nanoparticles
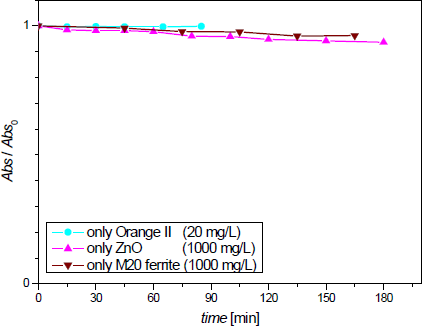
Figure 6 shows dye absorbance at 485 nm in the centrifuged Z00 composite supernatants divided by the initial value, Abs(t)/Abs0 vs. time. By Lambert-Beer’s law, these relative absorbances should be equal to the relation C(t)/C0, between the concentration at any time and the initial concentration at the beginning of the light exposition. Orange II solution behaviour without any catalyst is included for comparison (Experiment #1 - Table 2).
It can be seen in Figure 6 that dye concentration slightly varies in the absence of catalyst (orange symbol), whereas suspended Z00 catalyst particles (blue symbols) cause an exponential decay in the azo dye absorbance. This type of curve would match with a first-order kinetics reaction.
Orange II / Z00 composite discoloration with light exposition
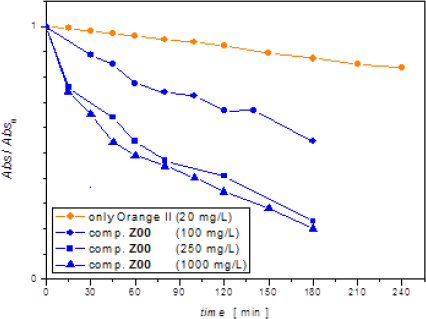
Figure 7 shows Abs(t)/Abs0 values as a function of time for the kinetics experiments carried out with 1000 mg/L of Z00, Z20, or ZnO nanoparticles suspended in the Orange II 20 mg/L solution.
Orange II / Z00 or Z20 composites and zinc oxide discoloration with light exposition (for comparison Orange II is included)
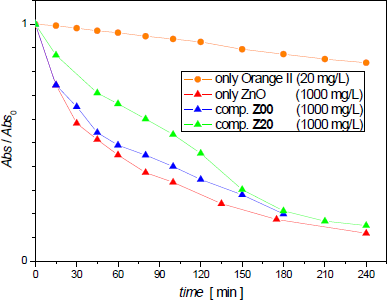
It can be seen in Figure 7 that Z00 composite and single zinc oxide suspended particles cause similar absorbance exponential decays. The Z20 composite (Nd-Co substituted strontium ferrite) curve presents a similar profile, although its initial slope is less negative than the others.
Kinetics of all photocatalytic reactions agreed with a first-order law, as Marto and other authors had found using only ZnO [8]. The first-order reaction rate constant (k1) and half-life (t½) parameters were calculated from the initial slopes of relative absorbance logarithmic graphs. These values are shown in Table 4.
Kinetics parameters calculated from the experiments (T = 25 °C)
Experiment # | Description | Slope, k1 [1/min] | t½ = ln 2 / k1 [min] |
4 | ZnO 1000 mg/L | 0.0161 | 43.0 |
6 | Z00 100 mg/L | 0.0037 | 187 |
7 | Z00 250 mg/L | 0.0107 | 64.7 |
8 | Z00 1000 mg/L | 0.0142 | 48.8 |
9 | Z20 1000 mg/L | 0.0078 | 89.1 |
The t½ values for the 1000 mg/L catalyst suspensions were 49 min for Z00 composite and 90 min for Z20 composite. Then, the rate of the reaction catalyzed by composites decreased compared with that of the ZnO suspension with the same nominal concentration (t½ = 43 min).
However, it should be considered that suspensions of Z00 composite have a real mass of zinc oxide 7.4% less than their nominal composition (ZnO mass fraction = 0.926). Therefore, it should be concluded that the decrease in the reaction rate using the composite instead of single ZnO should be lesser than the previously calculated value.
It can be seen in Figure 6 that the initial rate is related to the catalyst concentration up to a value of 250 mg/L. For a higher catalyst concentration (1000 mg/L), the discoloration rates behave similarly for both hexaferrite composites after 180 min, although their initial rates are somewhat different (Figure 7). It is noticeable that the Sr ferrite supported catalyst (blue symbol) presents a similar behaviour as single ZnO nanoparticles (red symbol).
No other analytical technique was used in this work to measure any degradation products from the Orange II. However, as control experiments in darkness showed no significant azo dye adsorption, we proposed that the main discoloration process should be the catalyzed oxidation of the azo group with light exposition. It can also be inferred that photocatalyzed azo dye reaction should be the same with all the tested catalysts, magnetic composites, or suspended ZnO nanopowders.
Figure 8 shows reaction rate constant k1 values obtained from the experiments with ZnO powder and Z00 composite vs. catalyst loading. Within the tested range of catalyst concentration, the value of k1 for the ZnO powder measurements increases linearly with the catalyst load. However, the rate-constant increment in Z00 experiments becomes less steep for amounts of suspended composite greater than 250 mg/L. This behaviour has already been reported and analyzed by Sakthivel et al. [37].
At first, a linear rise in the rate constant with the amount of catalyst is observed. This has been attributed to the increase of active sites on the photocatalyst surface, which results in a greater generation of •OH radicals, that would oxidize more azo dye molecules. But, as Nishio et al. found, for ZnO concentrations between 1000 and 1500 mg/L, the slope substantially decreases, and the rate constant becomes nearly independent of the catalyst load [3].
It has been reported by Kaur et al. that the increment of solution turbidity would hinder light transmission due to the significant scattering, and there would be no longer increment of hydroxide radical generation [38]. Therefore, there is an optimal level of zinc oxide concentration, and a further increase of the catalyst load will not be effective. This optimal value would seem smaller for the Z00 composite (between 250 and 1000 mg/L), probably due to a more intensive scattering of the suspended ferrite particles.
Variation of rate constant with catalyst loading
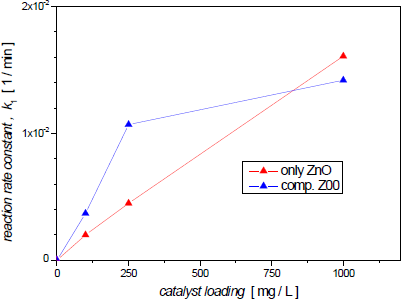
The suspended photocatalysts were removed from dye solutions by magnetic decantation with an iron-neodymium-boron (Fe-Nd-B) alloy magnet and then dried and weighed [39]. Composites were recovered with an average factor of 70%. This magnetic removal was carried out as a lab procedure and has not been tested at an industrial scale. Probably, there would be no severe disadvantages of the usage and recovery of a photocatalyst supported on magnetic particles in an industrial application since similar techniques have already been published and patented [40]. These procedures included the remotion of magnetic particles from seawater [41].
Composites prepared with ZnO precipitated nanoparticles on hexaferrite magnetic particles (Sr ferrite and Nd-Co substituted Sr ferrite) were successfully synthesized by a one-step coprecipitation method. These composites retain their magnetic properties after preparation at neutral pH. SEM images show that zinc oxide nanoparticles cannot completely coat Sr ferrite particles, although they seem to be steadily attached to the hard magnetic phase.
ZnO nanopowders and the prepared composites were tested as photocatalysts for Orange II dye discoloration in aqueous solution at 25 °C with light exposition at pH = 7.2. Preliminary experiments performed in darkness showed that the adsorption of the azo dye over ZnO or ferrite particles is negligible.
The synthesized composites present good catalyst properties in the photocatalyzed discoloration of the pollutant Orange II compared to single zinc oxide nanoparticles dispersed in water at the same experimental conditions. First-order reaction rate coefficients and half-life values calculated for Sr ferrite/ZnO composites are quite similar to those observed with zinc oxide nanopowder. The Nd-Co substituted Sr ferrite/ZnO catalyst showed the lowest catalytic activity, but its kinetics parameters were good enough to achieve a significant azo dye discoloration.
Composite particles can be easily removed from the water, by magnetic decantation, with a significant recovery factor (70%). These preliminary results indicate that these supported magnetic catalysts can be satisfactorily employed for industrial remediation. The influence of ZnO particle size on the photocatalytic activity of these composites and the re-use of the recovered catalyst particles will be studied in further experiments.
Removal of C.I. Acid Orange 7 from aqueous solution by UV irradiation in the presence of ZnO nanopowder ,J. Hazard. Mater , Vol. 143 (1-2),pp 95-101 , 2007, https://doi.org/https://doi.org/10.1016/j.jhazmat.2006.08.072
, Photocatalytic Degradation of a Real Textile Wastewater Using Titanium Dioxide, Zinc Oxide and Hydrogen Peroxide ,Int. J. Eng. Sci , Vol. 5 (7),pp 61-70 , 2016
, Photocatalytic Decolorization of Azo-dye with Zinc Oxide Powder in an External UV Light Irradiation Slurry Photoreactor ,J. Hazard. Mater , Vol. 138 (1),pp 106-115 , 2006, https://doi.org/https://doi.org/10.1016/j.jhazmat.2006.05.039
, Photocatalytic Degradation of Azo Dye Acid Red 14 in Water on ZnO as an Alternative Catalyst to TiO2 ,J. Photochem. Photobiol. A Chem , Vol. 162 (2-3),pp 317-322 , 2004, https://doi.org/https://doi.org/10.1016/S1010-6030(03)00378-2
, Evaluation of the Photocatalytic Potential of TiO2 and ZnO Obtained by Different Wet Chemical Methods ,Mater. Res. - Iberoam. J. Mater , Vol. 20 (2),pp 181-189 , 2017, https://doi.org/https://doi.org/10.1590/1980-5373-MR-2016-0936
, A Review of ZnO Nanoparticles as Solar Photocatalysts: Synthesis, Mechanisms and Applications ,Renew. Sustain. Energy Rev , Vol. 81 ,pp 536-551 , 2018, https://doi.org/https://doi.org/10.1016/j.rser.2017.08.020
, Preparation of Zinc Oxide (ZnO) Powders with Different Types of Morphology by a Combustion Synthesis Method ,Mater. Sci. Eng. B , Vol. 140 (1-2),pp 31-37 , 2007, https://doi.org/https://doi.org/10.1016/j.mseb.2007.03.023
, Photocatalytic Decolouration of Orange II by ZnO Active Layers Screen-printed on Ceramic Tiles ,J. Hazard. Mater , Vol. 163 (1),pp 36-42 , 2009, https://doi.org/https://doi.org/10.1016/j.jhazmat.2008.06.056
, Photocatalysis with Nanostructured Zinc Oxide Thin Films: The Relationship between Morphology and Photocatalytic Activity under Oxygen Limited and Oxygen Rich Conditions and Evidence for a Mars Van Krevelen Mechanism ,Appl. Catal. B Environ , Vol. 97 (1-2),pp 168-181 , 2010, https://doi.org/https://doi.org/10.1016/j.apcatb.2010.03.037
, Enhancement of Photocatalytic Degradation of Methyl Orange by Supported Zinc Oxide Nanorods/Zinc Stannate (ZnO/ZTO) on Porous Substrates ,Ind. Eng. Chem. Res , Vol. 52 (38),pp 13629-13636 , 2013, https://doi.org/https://doi.org/10.1021/ie4019726
, On the Discoloration of Methylene Blue by Visible Light ,J. Fluoresc , Vol. 29 (1),pp 15-25 , 2019, https://doi.org/https://doi.org/10.1007/s10895-018-2304-6
, ZnO Hierarchical Microsphere for Enhanced Photocatalytic Activity ,J. Alloys Compd , Vol. 741 ,pp 622-632 , 2018, https://doi.org/https://doi.org/10.1016/j.jallcom.2018.01.141
, Flower-like ZnO Hollow Microspheres on Ceramic Mesh Substrate for Photocatalytic Reduction of Cr(VI) in Tannery Wastewater ,Ceram. Int , Vol. 42 (14),pp 15968-15974 , 2016, https://doi.org/https://doi.org/10.1016/j.ceramint.2016.07.098
, Photocatalytic Degradation of Methylene Blue Dye by Zinc Oxide Nanoparticles Obtained from Precipitation and Sol-gel Methods ,Environ. Sci. Pollut. Res , Vol. 23 (24),pp 25485-25493 , 2016, https://doi.org/https://doi.org/10.1007/s11356-016-7750-6
, Study on Photocatalyst Zinc Oxide Annealed at Different Temperatures for Photodegradation of Eosin Y dye ,J. Alloys Compd , Vol. 695 ,pp 270-279 , 2017, https://doi.org/https://doi.org/10.1016/j.jallcom.2016.10.194
, Synthesis, Characterization and Optical Properties of Zinc Oxide Nanoparticles ,Int. Nano Lett , Vol. 3 (30),pp 1-6 , 2013, https://doi.org/https://doi.org/10.1186/2228-5326-3-30
, Recent Advances in the Application of Magnetic Nanoparticles as a Support for Homogeneous Catalysts ,Nanomaterials , Vol. 4 (2),pp 222-241 , 2014, https://doi.org/https://doi.org/10.3390/nano4020222
, Synthesis and Characterization of Cobalt-supported Catalysts on Modified Magnetic Nanoparticle: Green and Highly Efficient Heterogeneous Nanocatalyst for Selective Oxidation of Ethylbenzene, Cyclohexene and Oximes with Molecular Oxygen ,J. Colloid Interface Sci , Vol. 506 ,pp 10-26 , 2017, https://doi.org/https://doi.org/10.1016/j.jcis.2017.06.100
, Comparative Dissolution, Uptake, and Toxicity of Zinc Oxide Particles in Individual Aquatic Species and Mixed Populations ,Environ. Toxicol. Chem , Vol. 38 (3),pp 591-602 , 2019, https://doi.org/https://doi.org/10.1002/etc.4349
, Role of Soluble Zinc in ZnO Nanoparticle Cytotoxicity in Daphnia magna: A Morphological Approach ,Environ. Res , Vol. 148 ,pp 376-385 , 2016, https://doi.org/https://doi.org/10.1016/j.envres.2016.04.028
, Metal Homeostasis Disruption and Mitochondrial Dysfunction in Hepatocytes Exposed to Sub-toxic Doses of Zinc Oxide Nanoparticles ,Nanoscale , Vol. 8 (43),pp 18495-18506 , 2016, https://doi.org/https://doi.org/10.1039/c6nr05306h
, Comparative in vitro Genotoxicity Study of ZnO Nanoparticles, ZnO Macroparticles and ZnCl2 to MDCK Kidney Cells: Size Matters ,Toxicol. Vitr , Vol. 40 ,pp 256-263 , 2017, https://doi.org/https://doi.org/10.1016/j.tiv.2017.01.015
, Application of Spinel Ferrite Nanoparticles in Water and Wastewater Treatment: A Review ,Sep. Purif. Technol , Vol. 188 ,pp 399-422 , 2017, https://doi.org/https://doi.org/10.1016/j.seppur.2017.07.015
, Visible Light Enhanced Heterogeneous Photo-degradation of Orange II by Zinc Ferrite (ZnFe2O4) Catalyst with the Assistance of Persulfate ,Sep. Purif. Technol , Vol. 165 ,pp 42-52 , 2016, https://doi.org/https://doi.org/10.1016/j.seppur.2016.03.026
, Visible-light-induced Photocatalysis and Peroxymonosulfate Activation over ZnFe2O4 Fine Nanoparticles for Degradation of Orange II ,Catal. Sci. Technol , Vol. 6 (7), 2016, https://doi.org/https://doi.org/10.1039/c5cy01735a
, ZnFe2O4 Nanoparticles and a Clay Encapsulated ZnFe2O4 Nanocomposite: Synthesis Strategy, Structural Characteristics and the Adsorption of Dye Pollutants in Water ,RSC Adv , Vol. 5 (69),pp 56145-56156 , 2015, https://doi.org/https://doi.org/10.1039/c5ra02163d
, Characterization-performance of ZnO and ZnO/ZnFe2O4 Catalyst Using Artificial and Solar Light for Mercury (II) Reduction ,Brazilian J. Chem. Eng , Vol. 36 (2),pp 797-810 , 2019, https://doi.org/https://doi.org/10.1590/0104-6632.20190362s20180280
, Adsorption and Catalytic Degradation of Organic Dyes in Water Using ZnO/ZnxFe3-xO4 Mixed Oxides ,J. Environ. Chem. Eng , Vol. 3 (2),pp 1185-1193 , 2015, https://doi.org/https://doi.org/10.1016/j.jece.2015.04.021
, Comparison of the Solar Photocatalytic Activity of ZnO-Fe2O3 and ZnO-Fe(0) on 2,4-D Degradation in a CPC Reactor ,Photochem. Photobiol. Sci , Vol. 14 (3),pp 543-549 , 2015, https://doi.org/https://doi.org/10.1039/c4pp00274a
, Nd-Co Substituted Strontium Hexaferrite Powders with Enhanced Coercivity ,Mater. Res. Bull , Vol. 47 (11),pp 3881-3887 , 2012, https://doi.org/https://doi.org/10.1016/j.materresbull.2012.08.047
, Preparation, Characterization and Application of Fe3O4/ZnO Core/Shell Magnetic Nanoparticles ,Mater. Res. Bull , Vol. 43 (8-9),pp 2457-2468 , 2008, https://doi.org/https://doi.org/10.1016/j.materresbull.2007.07.035
, - , , Revised Ed. Hoboken, 1998
- , , 2nd ed. Barcelona, 1999
Adsorptive Removal of Acid Orange 7 from Aqueous Solution with Metal-organic Framework Material, Iron (III) Trimesate ,Desalin. Water Treat , Vol. 57 (7),pp 3218-3226 , 2016, https://doi.org/https://doi.org/10.1080/19443994.2014.982199
, - , , 7th ed. Orlando, 2000
- , , 2nd ed. Hoboken, 2009
Solar Photocatalytic Degradation of Azo Dye: Comparison of Photocatalytic Efficiency of ZnO and TiO2 ,Sol. Energy Mater. Sol. Cells , Vol. 77 (1),pp 65-82 , 2003, https://doi.org/https://doi.org/10.1016/S0927-0248(02)00255-6
, Photocatalytic Degradation of Methyl Orange Using ZnO Nanopowders Synthesized via Thermal Decomposition of Oxalate Precursor Method ,Phys. B Condens. Matter , Vol. 416 ,pp 33-38 , 2013, https://doi.org/https://doi.org/10.1016/j.physb.2013.02.005
, - , , University of Buenos Aires (UBA), 2017
- , Process for Cleaning Up Oil Spills, US 3635819 A, 1972
- , Magnetic Colloid Petroleum Oil Spill Clean-up of Ocean Surface, Depth, and Shore Regions, WO 2012/115814 A1, 2012