Climate change and climate protection are priority issues on international and national policy agendas. With the 2015 Paris Agreement, signatory states committed to limiting global warming to well below two degrees (aiming for 1.5 degrees) via national contributions to climate protection (Nationally Determined Contributions, NDCs). While the joint commitment of industrialised, developing and newly industrialising countries seems promising for effective global climate action, analyses of NDCs reveal increasing gaps between the necessary and the expected reductions in greenhouse gas (GHG) emissions through the implementation of the NDCs (see, e.g. [1]).
According to [2], the share of total global GHG emissions stemming from natural resource extraction and processing has risen from around 15% in 1995 to 23% in 2015. This share increases to 45% if the production of goods from natural resources is also taken into account, e.g., the manufacture of products such as cars, clothing, and food [3]. Therefore, fostering resource efficiency and circular economy holds promising potential for reducing GHG emissions on a global level, and therefore for climate protection. A study from 2019 estimated that waste elimination, product re-use, material recirculation and circular economy approaches in the areas of aluminium, cement, food, plastics, and steel “could reduce global CO2 emissions from key industry materials by 40% or 3.7 billion tonnes in 2050” [3] (p. 26). Therefore, it appears relevant to systematically integrate the circular economy and resource efficiency approaches into climate action to step up GHG emission saving potentials. Such a systematic perspective also enables the detection of synergies between climate protection and resource use and trade-offs, in particular the increasing need for (certain) raw materials for the decarbonisation of construction, energy, and mobility systems (such as metals for batteries, photovoltaics, and wind turbines).
In this paper, such a systematic perspective, which integrates interlinkages between climate action and resource conservation, is understood to form the ‘climate-resource-nexus’ which in [4] is called Circular Economy (CE)-climate change nexus.
Although the climate-resource-nexus is not an established term (as compared, for instance, to the water-energy-food nexus; see, e.g. [5]), the importance of resource conservation as a relevant strategy for achieving climate goals was recently stressed at the international level [6], [7]. In a report from 2017, it was stated that improving resource efficiency is indispensable “in helping countries meet their aspirations as enshrined in the SDGs and the Paris Agreement on Climate Change” [8] (p. 299) and “for meeting climate change targets cost effectively” [9] (p. 4). The topic was also raised at G7 [10] and G20 [11] level, e.g., in the context of climate benefits of waste management and resource efficiency approaches.
Furthermore, the topic has been receiving growing attention from the scientific community. Since 2010, the number of studies investigating interactions between climate and resource policy has increased noticeably. One main thread emerging from the literature addresses the increasing (abiotic and biotic) raw materials demands for low-carbon technologies. Here, progress in international climate research and advances in computer modelling fuelled a rise in studies focussing on resource needs of climate-friendly technologies. Issues covered by this scientific research encompass analyses of global metal flows associated with renewable energy transition [12] as well as analyses of global metal requirements related to 100% renewable energy [13] and low-carbon power generation [14]. Their basic observation is that the introduction, scale-up, and spread of low-carbon technologies – such as renewable energies or battery-powered cars – requires a high quantity of raw materials, particularly metals. Another study investigated climate change and sustainability as drivers for the next mining and metals boom [15]. It argued that “analysis of the increased demands of the transition to the low-carbon economy shows it could lead to the next mining and metals boom increasing metals demand by up to 10-fold by 2050” [15] (p. 101205). In this context, numerous studies have been set up to investigate the following question: Is it feasible to implement an ambitious climate policy with modern, metal-intense technologies on a large scale (country, EU, or even global level), or will resource constraints hinder such projects? Hence, some studies take on the perspectives of the supply side, for instance, investigating potential metal supply constraints for a low-carbon transition on a global level [16]. For the national level in China, one study analysed critical metal needs related to national and international energy scenarios [17], while another study investigated short- to medium-term supply risks of clean energy minerals [18]. Although findings vary, the three aforementioned studies consider resource demands at the global level challenging but feasible. Furthermore, despite the potential increase in demands for certain raw materials (mostly metals and minerals) through low-carbon technologies, one study finds that in an integrated lifecycle perspective, low-carbon technologies show global environmental benefits overall [19].
Many comparable studies draw more pessimistic conclusions, indicating that resource-specific constraints are likely to hamper the transition to a low-carbon economy and the achievement of political climate goals. While one study finds that the metal requirements needed to achieve the climate target of staying well below 2 °C could hamper effective climate protection [13]. Another study sees resource constraints regarding critical metals in the International Energy Agency’s Long-Term Low-Carbon Energy Scenarios [20]. In this context, critical metals are considered to play a decisive role in the future markets of clean energy technologies [21].
Moreover, researchers point to various resources that might become critical in the event of a global or regional transition towards climate-friendly infrastructures and technologies. This concerns, for example, the metals silver [22], copper [23], lithium [12], [20], indium and tellurium [20], [21] on the global level. For the European region, this concerns specific rare earth metals such as neodymium [24]. In addition, one study finds the expansion of renewable energies also affects land as a resource, as the accompanying infrastructures (e.g., wind turbines, photovoltaic elements, storage facilities, bioenergy crops) need land area and space to be installed [25].
Another main thread of scientific studies concerns potential synergies between climate protection and resource conservation policies by showing that resource conservation, material efficiency, and circular economy approaches contribute to climate change mitigation. Studies cover analyses of potential GHG emission reduction effects of material efficiency strategies related to buildings, vehicles, and electronics [26], as well as of measures to re-use goods [27], of circular economy approaches in Austria [28] and the city of Guiyang, China [29], and of sustainable consumption strategies [30]. Computer-based model simulations on the global level indicate that there is a significant untapped potential to reduce GHG emissions through measures that enhance material efficiency, achieving GHG emission reductions of 15–20% by 2050, [31] or up to around 40% by 2050 if climate action is included [32]. One study finds a global resource efficiency scenario to help cut GHG emission targets by half [33]. Another study estimated that applying circular economy strategies in the heavy industry sector (focussing on steel, plastics, aluminum, and cement) could save up to 296 million tonnes of CO2 per year in the EU, and on the global level, up to 3.6 billion tonnes by 2050 [34]. However, a critical issue is that rebound effects are likely to occur when such measures are implemented in isolation [31]. This means that resources become cheaper or more easily available – due to resource efficiency gains, which incentivises a higher consumption. There is a risk that such rebound effects may negate both the aggregate resource savings and the associated greenhouse gas emission reductions at the global or European level [32] and at the national level, for instance, in the UK, cf. [35].
Despite the increasing number of studies, interactions between climate protection and resource conservation – the climate-resource-nexus – still need to be better understood and systemically analysed [26]. On the one hand, the climate-resource-nexus seems to be explored from a primarily scientific perspective, with studies taking on either a global or European focus or focussing on specific sectors. On the other hand, only a few studies consider potential effects of resource policy on GHG emissions – and those that do, investigate only a small set of policies. And while quantitative findings of modelling studies relate to the global level, it appears that they apply Eurocentric or Global North environmental policies across the globe and fail to acknowledge whether or not they can be implemented in different socio-cultural contexts across the globe.
To advance the understanding and consideration of the climate-resource-nexus in international policy, perspectives on policy relevance and fit in various world regions were explored, covering, in particular, the Global South. Thus, Global South’s perspectives help us better understand how international policy for the climate-resource-nexus could be configured in different contexts.
As such, the present paper seeks to contribute empirical knowledge to answer the following research questions: (i) Which policy approaches are seen as relevant and promising in the context of the climate-resource-nexus in the scientific literature?; (ii) What are aspects affecting the potential relevance and feasibility of those policies in different world regions?
These research questions were tackled with an exploratory research approach: on the one hand, through an academic literature review, and on the other hand via semi-structured online interviews with topical experts from international organisations, civil society organisations, and academia across different world regions.
This paper presents findings from this research. The literature review reveals that policies-addressing academic publications mainly analyse potential GHG emission reduction effects of different material efficiency and circular economy strategies. However, the climate-resource-nexus appears to guide policy considerations hardly. Interview findings reflect this, as only a few interviewees mention mutual effects of climate protection and resource conservation policies. Those who mention interactions consider insufficient cross-departmental exchange and silo culture as reasons for the lack of climate-resource-nexus thinking in the policy. This kind of analysis could contribute to strengthening systemic nexus thinking in international climate and resource policy while also fostering a view on adapting policies to the needs of specific contexts.
Overall, the present study aimed to analyse policies in relation to the so-called climate-resource-nexus. To guide our analyses, the term policy was operationalised to mean
overarching areas for policy intervention, which
aim at pursuing certain policy objectives,
for which different policy instruments (i.e., concrete implementation approaches, for instance, a tax on CO2 or single-use plastics) are available.
To exemplify this: a possible policy measure could be to raise prices of fossil fuels or virgin raw materials (= 1. policy intervention) to create an economic incentive for less GHG-emitting or resource-consuming activities (= 2. policy objective). Instruments to consider for this measure could be, for instance, a tax for CO2 or a material input tax (= 3. policy instruments).
The literature review aimed to identify academic publications (scientific and grey literature) that address climate and resource policy measures in the context of the climate-resource-nexus. Based on key findings from this review, relevant policy measures should emerge for use in interviews to explore actor perspectives from different world regions on the regional relevance and fit of those measures.
The research used the online search tools ScienceDirect and Google Scholar and applied snowball sampling for identifying relevant academic literature for the review. Since the coverage of the topic is relatively recent, we limited our search to the time 2010 to 2020. As a search strategy, the following combined search strings were used employing Boolean operators (AND / OR):
“resource” OR “resource efficiency” OR “resource conservation” OR “resource scarcity” OR “material efficiency” AND “climate” OR “climate protection” OR “climate mitigation” OR “climate abatement” OR “low carbon” AND “nexus” OR “link” OR “interaction” OR “interlinkage” OR “synergies” OR “trade-off” AND “policy” OR “policies” OR “instrument” OR “policy measure” OR “strategy”.
In ScienceDirect, different combinations of a maximum of eight of the aforementioned search strings were used in the search field “Title, abstract or author-specified keywords” under advanced search. In Google Scholar, all of the search strings mentioned above were applied at once.
Subsequently, the titles of the first 100 hits were screened for different combinations of the search strings in ScienceDirect, and the titles of the overall first 100 hits in Google Scholar, for potential relevance. Here, only the abstracts (or, in the case of grey literature, available summaries) of those literature hits whose titles seemed relevant to our research questions were selected for reading. The final step of selecting literature for integration in the review consisted of reading the abstracts or summaries and searching for potential relevance regarding climate and resource policies in the context of the climate-resource-nexus by checking whether or not, according to the abstract, the publication addressed:
Climate and resource (or circularity) issues and their interlinkages; Policies, instruments, or strategies concerning the abovementioned issues.
Only publications fulfilling both criteria a) and b) were included in the review. Through this procedure, 31 publications were identified.
However, after a comprehensive reading of these papers, only 19 studies were deemed relevant for this study because they addressed policy issues in a way that enriched our analyses. Hence, 12 of the 31 publications identified as promising by reading the abstract were dropped from the review because they were not found to offer relevant insights into policy measures or instruments. Instead, they only referred to the term “policy” in a general way, without elaborating on required actions, strategies, or instruments. The remaining 19 studies were then analysed based on the type and scope of policy measures addressed (see section Results).
Semi-structured online expert interviews (using a guideline for interview structuring) were used to explore actor perspectives from different world regions on the regional relevance and fit (regarding potential feasibility or suitability for implementation) of policies identified from the literature review.
Regional foci were (Sub-Saharan) Africa, Asia-Pacific (AP), Latin America and the Caribbean (LAC), Middle East and North Africa (MENA), and North America (NA). A European focus was intentionally left out because climate and resource policy in the EU appears relatively well-developed, considering, for instance, the European Green Deal and the associated European Climate Law, the 2030 Climate Target Plan, and the European Circular Economy Action Plan.
In some cases, the interviewees had particular expertise on a specific country within their regions, which were thus added as a proxy for regional views (or in the case of China, a very particular sub-region). The target group consisted of experts working formally in multilateral UN institutions in the different world regions who had expertise either on resource conservation or climate protection. Potential interviewees in the abovementioned regions of Africa, AP, LAC, MENA, and NA, were sought out through website searches of regional UN institutions and international programs and initiatives relating to climate protection or resource conservation. Interview consent could be secured from UN experts for the regions of Africa, AP, and LAC, but not for MENA and NA, despite repeated telephone and email enquiries, including asking recipients for alternative or additional expert recommendations. Therefore, the search for interviewees was expanded to environmental NGOs and academia to cover the missing regional expertise. Eventually, thirteen interviews covering all of the abovementioned regions could be conducted. For an overview of the interviewed experts, see Table 1.
Interviewees for resource conservation (RC) and climate protection (CP)
Interviewee |
Regions covered |
Thematic focus |
Specific country focus |
Type of organisation |
---|---|---|---|---|
CP1 |
Southern Africa |
Climate |
South Africa |
Research |
CP2 |
AP |
Climate |
- |
UN |
CP3 |
AP |
Climate |
- |
UN |
CP4 |
AP |
Climate |
China |
Civil Society |
CP5 |
LAC; Northern Africa |
Climate |
- |
UN |
CP6 |
LAC |
Climate |
Mexico |
Civil Society |
CP7 |
Middle East |
Climate |
Saudi Arabia |
Research |
CP8 |
NA |
Climate |
USA |
Research |
RC1 |
AP |
Resources |
- |
UN |
RC2 |
LAC |
Resources |
- |
UN |
RC3 |
Africa |
Resources |
- |
UN |
RC4 |
MENA |
Resources |
- |
Research |
RC5 |
NA |
Resources |
USA |
Research |
Two guideline questionnaires were prepared to obtain the expertise of both regional climate and regional resource experts. Each questionnaire contained the same set of questions (see below), differing only in the policy measures exposed to expert feedback – climate protection policy for climate experts and resource policy (including circular economy) for resource experts. Furthermore, each questionnaire posed a question on the climate-resource-nexus, whether or not the measures discussed could impact resource use or GHG emissions in their regions.
Thus, the questions covered the following aspects:
Relevance of (i) climate policy measures to reduce GHG emissions or (ii) resource policy measure to foster resource conservation in the region (relevance including identifying experts’ top 3 list of measures),
Potential challenges and benefits of each measure for the region,
Potential blind spots, i.e., missing measures that could significantly strengthen (i) climate protection or (ii) resource conservation in the region,
Potential impacts of (i) climate protection measures on resource use/raw material needs or (ii) resource policy on GHG emissions/climate change in the region.
Interviewees obtained a brief description of the measures identified in the literature review and the abovementioned interview guideline. After the interview, written summaries of the conversation were sent to the interviewees enabling them to check, correct and validate the interviews. These summaries formed the basis for qualitative content analysis focused on the relevance, challenges, opportunities, and potential foci of measures in the different regions and gaps in those measures. Moreover, the empirical findings helped create an explorative understanding of whether – and to what degree – interviewees perceive potential synergies and trade-offs between measures of climate protection and resource conservation.
Table 2 and Table 3 provide an overview of policy measures addressed by the 19 academic publications selected as relevant to this study (for a more detailed version, see Table 7 to Table 10 in the Annex).
The literature review revealed that publications, on the one hand, analyse policies primarily regarding policy measures for material efficiency, resource conservation, or circular economy and their potential to reduce GHG emissions (see, e.g., [39]). Most policy measures identified were found to look into material efficiency or circular economy approaches and their potential effects on GHG emission reductions. Only a few investigate the possible impacts of climate protection policies and low-carbon transitions on resource conservation issues (see, e,g., [40]). On the other hand, the literature review revealed that several studies address the policies specific to particular areas/sectors, including the construction sector (see, e.g., [41]), heavy industry (see [42]), vehicles (see, e.g., [40]), waste management (see, e.g., [43]), or to particular materials such as metals (see [44]).
In addition, most policy approaches were found either rather broad (in several modelling studies, e.g., lifetime extension, material substitution, product design changes, or taxation) or very specific to particular sectors, materials, or products (e.g., quality standards for secondary construction materials).
Therefore, to select and consolidate policy measures for the interviews on their perceived regional relevance and fit, the set of policy measures from this literature review was cross-checked and expanded relying on literature that explicitly deals with climate protection policies on the one hand and circular economy and resource conservation policies on the other hand.
Overview of policy measures that selected scientific publications address in the context of the climate-resource-nexus; last name author A – G
Publication |
Policy measures addressed |
Specific sectoral or product coverage |
---|---|---|
Aracil et al. 2017 [45] |
Through resource efficiency, i.e., landfill banning, waste-based biofuels yield climate benefits. |
Waste management, biofuels |
Barrett and Scott 2012 [35] |
Material efficiency strategies, including changing diets (reducing animal-based food products), extending product lifetimes and improving durability, shifting from owning products to using services, lightweighting, material substitution, and recycling, show considerable GHG emission reduction potentials. |
(No specific sectoral coverage) |
Bonsu 2020 [40] |
Circular business model, product and service design, enabling legislation and rewards or incentives for circular solutions critical for environmentally and socially robust end-of-life battery management. |
(Electric) Vehicles |
Distelkamp and Meyer 2019 [32] |
Global GHG emission reduction effects of, inter alia, resource efficiency approaches (e.g., upstream tax on metal ores and non-metallic minerals, mandatory recycling quotas). |
(No specific sectoral coverage) |
Dienst et al. 2015 [46] |
Resource efficiency to reduce the use of steel and cement are needed alongside climate protection strategies to achieve per capita CO2 emission levels that comply with the 2 °C target. |
Energy sector, manufacturing industry, transport; steel and cement production |
Ellen MacArthur Foundation 2019 [3] |
Circular economy strategies – in particular, recirculating materials (e.g., recycling), re-using products and components (e.g., remanufacturing), eliminating waste (e.g., by changing product design and extending product lifetimes), and regenerative agriculture will support climate change mitigation. |
Aluminium, cement, food, plastics and steel; agriculture, construction, mobility |
Fang et al. 2017 [29] |
Circular economy strategies via urban industrial symbiosis (in particular substituting cement with aluminium, phosphorous and steel slag) promises great carbon footprint reduction potential (compared to recycling of plastic, steel, and tire waste; utilising waste heat between industrial sectors, for urban areas and the agriculture sector). |
Agriculture and forestry; industry; construction; transportation, storage and postal service; whole sales and retail |
Gallego-Schmid et al. 2020 [47] |
Reducing embodied emissions on the consumption side, for instance, by increasing durability and reusability of materials and products and substituting materials, e.g., for wood. |
Buildings and construction |
Gonzalez Hernandez et al. 2018 [42] |
Material efficiency approaches (including industry standards on material efficiency, industry-led material efficiency initiatives, tax reliefs to encourage material efficiency, redesign of the ETS emissions cap by allowing capturing material efficiency improvements in the calculation of annual emission reduction rates) achieve carbon emission reductions in heavy industries. |
Heavy industries |
Overview of policy measures that selected scientific publications address in the context of the climate-resource-nexus; last name author H – V
Publication |
Policy measures addressed |
Specific sectoral or product coverage |
---|---|---|
Hatfield-Dodds et al. 2017 [31] |
Global GHG emission reduction effects of resource efficiency measures (e.g., technical improvements, resource extraction taxes, as well as regulation and green public procurement). |
(No specific sectoral coverage) |
Hertwich et al. 2019 [26] |
Material efficiency strategies (e.g. lightweighting, lifetime extension, re-use, remanufacturing and repair, material substitution, and recycling) show great GHG emission reduction potential. |
Buildings and construction, electronics, vehicles |
Huang et al. 2013 [48] |
Lifetime extension of buildings and improved materials recycling significantly reduce material (iron ore and limestone) demand, solid waste generation, and CO2 emissions. |
Buildings and construction |
Material Economics 2018 [34] |
Supply-side and demand-side circular approaches (material recirculation, product material efficiency, and circular business models) show great potential for GHG emission reductions. |
Aluminium, plastics, steel; buildings and construction, passenger cars |
Meyer et al. 2018 [39] |
Global GHG emission reduction effects of policy supporting technology improvements in resource-relevant production sectors to foster resource efficiency. |
(No specific sectoral coverage) |
Nußholz et al. 2019 [41] |
Material efficiency approaches, e.g., mandatory pre-demolition audits and selective demolition, quality standards for secondary materials, resource conservation criteria in building codes and public procurement processes, reduce embodied GHG emissions of building materials. |
Buildings and construction |
Röck et al. 2020 [49] |
Building requirements, life cycle performance benchmarks, and end-of-life treatment of construction materials reduce embodied GHG emissions of building materials. |
Buildings and construction |
Shen et al. 2021 [50] |
Policy aimed at increasing resource efficiency and material substitution needed alongside energy efficiency improvements to counteract increasing emissions from rapid urbanisation. |
Buildings and construction, cement production |
Turner et al. 2016 [43] |
Waste prevention, diversion of food waste from the residual waste stream, and waste recycling form the potentially most effective strategy to reduce GHG emissions |
Waste management |
van der Voet et al. 2019 [44] |
Increasing the share of secondary production proves a practical approach to reducing GHG emissions from metal production. |
Metals (aluminium, copper, iron, lead, manganese, nickel and zinc) |
For the selection and consolidation of policy measures for use in the interviews, other literature was analysed despite the fact it was initially excluded from the literature search procedure described above because it did not address nexus issues. This expanded body of literature covered policies exclusively about climate protection, resource conservation, and circular economy. Finally, a set of climate protection policy measures (see Table 4) and resource conservation measures (see Table 5) were consolidated, which served as a basis for the interviews.
Overview of policy measures for climate protection (MC)
No. |
Policy measure |
Mechanism and objective(s) of policy measure |
---|---|---|
MC1 |
Shifting to 100% Renewable Energy |
Renewable energy sources supply all energy such as electricity, heating and cooling, and fuels and gases. The use of technology and infrastructure is changed to support renewable energy utilisation in electricity grids, gas networks, heat infrastructure, and energy storage systems (see, e.g., [51]). |
MC2 |
Increasing Electrification |
Electrification across all sectors reduces the direct use of (fossil) fuels, e.g., by electrifying transportation and industrial processes, and by using electricity to generate fuels (power-to-gas; power-to-liquids; see, e.g., [52]). The measure aims at achieving sector coupling, i.e., using electricity in various sectors in an integrated way (e.g., physical linking of infrastructures, new institutional or organisational integration). Electrification as a means of climate mitigation depends on 100% renewable electricity (see MC1). |
MC3 |
Shifting to Zero-Emission Buildings |
The measure yields a high renovation rate with deep energy-oriented modernisations in the existing building stock and implements zero-emission standards for new buildings (see, e.g., [53]). Renewable energies will provide direct and indirect energy consumption, e.g., electricity or heating/cooling. It also includes the use of low-emission construction materials. |
MC4 |
Reducing Fertiliser Use and Liquid Manure Input on Fields |
Reducing the use of fertilisers and liquid manure in the agriculture sector reduces direct and indirect emissions of methane (liquid manure) and nitrous oxides (liquid manure and mineral fertilisers), which are potent GHGs. This measure includes the option of manure pre-treatment in biogas facilities and changes in agricultural practices, including organic farming (see, e.g., [54]). |
MC5 |
Changing Life-Styles and Sufficiency |
Global consumption patterns have a high impact on GHG emissions. There is an urgent need for changes (see, e.g., [55]), including a dietary shift towards a more plant-based diet. This measure aims at increasing social acceptance for lifestyle changes and sufficiency, e.g., through awareness-raising. |
MC6 |
Increasing Natural Carbon Sinks |
The measure aims to increase natural carbon sinks and raise climate mitigation through land use, land-use change, and forestry (see, e.g., [56]). A focus is on the protection of forests and their economic use. |
Overview of policy measures for resource conservation (MR)
No. |
Policy measure |
Mechanism and objective(s) of policy measure |
---|---|---|
MR1 |
Setting global per-capita resource-use budgets |
Capping per capita resource use may be an avenue to reducing resource consumption (see, e.g., [57]). Developing an international convention for resource conservation could be a means to negotiate and implement global per-capita resource-use budgets. |
MR2 |
Raising prices of virgin materials |
Material prices reflect the value a material has for users. Relatively higher primary material prices, e.g., through taxes, can incentivise a more thoughtful, more sustainable use of materials, such as using secondary instead of primary materials (see, e.g., [58]). |
MR3 |
Fostering markets for sustainable products and secondary raw materials |
Resource-conserving products and services, as well as secondary raw materials, often lack a proper market. Therefore, demand is rather low, and prices are relatively high, hampering further uptake and diffusion of such products and secondary raw materials. The measure aims at creating a market for secondary raw materials (see, e.g., [59]). |
MR4 |
Designing products and business models for material efficiency and long service life |
The design of products, production processes and business models largely determines the amount of primary raw materials needed for them. Therefore, this measure includes improving design considerations regarding material selection, material efficiency in production, and extended lifetime of products to reduce raw material needs, e.g., through innovative business models (see, e.g., [60]). |
MR5 |
Making re-use and repair of products easier and more attractive |
Re-using and repairing products can make their service life significantly longer. However, repair options are scarce and expensive, and spare parts and incentives for product re-use are missing. This measure includes incentivising repair and re-use of products (with a particular focus on electronic devices) (see, e.g., [60]). |
MR6 |
Reducing plastic waste |
Plastics production and disposal have steeply increased worldwide throughout the last five decades, primarily as single-use packaging and utensils. Linked to this increased plastic production is an increase in littering and incineration, which has a variety of negative impacts on the ecosystem. The measure aims to reduce plastics quantities considerably along the entire value chain, thus lowering the need for virgin plastics (see, e.g., [34]). |
MR7 |
Making the built environment more material-efficient |
The built environment is increasing exponentially worldwide, and the construction sector constitutes one of the largest material consumers and GHG emitters worldwide. Therefore, reducing the amount of primary construction materials offers significant potential. This measure aims at increasing material efficiency in buildings, as well as incentivising an improved use of construction and demolition waste (CDW) as a raw material (see, e.g., [61]). |
MR8 |
Creating a sustainable food system |
One of the biggest sustainability challenges in light of continuous population growth is achieving food security without depleting resources such as land and water beyond the planet’s carry capacity. The measures aim at creating a food system that reduces the resources needed to feed the population healthily (e.g., through plant-based diets and the reduction of food wastesee, e.g., [62]). |
Climate protection policy measures primarily aim at: (i) reducing CO2 emissions from energy generation and energy use by substituting fossil for renewable energy sources; (ii) fostering energy efficiency and zero-emission approaches in specific sectors (agriculture and buildings) and overall consumption patterns (lifestyle changes). In addition, changing land and forest management to increase natural carbon sinks plays a crucial role.
The resource conservation measures follow two primary goals. These are, on the one hand, reducing the amounts of virgin resources used to produce goods and services employing economic levers (MR2, MR3, and partially MR4 in terms of industrial material efficiency) (see, e.g., [2]). On the other hand, measures aim at increasing circularity in the entire lifecycle of products and business models (MR4 and MR5; see, e.g., [63]).
Moreover, three resource conservation measures (MR6 to MR8) focus on specific sectors that are particularly relevant regarding resource use or resource intensity:
The building sector (MR6) regarding its use of large quantities of mineral raw materials and the lifetimes of buildings and infrastructure, which can lock-in design and structures and binds resources for several decades (see, e.g., [26]);
Plastics (MR7) concerning globally increasing production quantities, mostly still fossil-fuel based, and the relevance of plastics regarding secondary material management and managing plastic littering (MR7) (see, e.g., [3]);
Food (MR8) in the context of the high socio-economic relevance of food security, complex value chains from ‘farm to fork’, and the diverse set of abiotic and biotic resources (including fertilisers, fossil energy carriers, water) needed, and environmental impacts (for instance, eutrophication, GHG emissions) associated with food production (see, e.g., [3]).
Despite the differentiation into climate protection and resource conservation measures, some aspects, such as life-style changes, play a role for both mitigation of GHG and reduction of resource use. ‘Changing life-styles and sufficiency’ has been formulated as a stand-alone measure for climate protection (MC5), which is a good summary of a variety of policy approaches aimed at raising awareness of the effect that individual choices can have on GHG emissions (see, e.g., [30]). In the case of resource conservation, life-styles mainly affect measures related to circular economy approaches (see, e.g., [29]) and less so material efficiency at an industrial level, making it less suitable as a stand-alone measure.
The literature underlying the abovementioned policy measures indicates their potential relevance for fostering climate protection and resource conservation on a global level. However, these measures might reflect a Eurocentric or industrialised country perspective, with most of the analysed literature coming from authors affiliated with European or North American research institutions. According to a study on design and implementation issues of governance for regional innovation [64], and another study on the role of integrating the regional context in landscape policy [65], the economic, geographical, governance, and socio-cultural context conditions primarily affect how and which policies are designed and implemented. Therefore, these measures were then exposed to the experience and views of regional experts regarding the measures’ contextual relevance and fit (suitability for implementation).
Figure 1 shows the most relevant measures as perceived by the interviewees from the different world regions.
Overall, the interviewees considered most of the climate protection and resource conservation measures relevant in their regional context. Interviewees only perceived a few measures to be either not relevant or unfeasible to implement:
Climate protection measure MC6 ‘Improving agricultural practices and increasing natural carbon sinks’ was not considered feasible, mainly due to the immense economic importance that agriculture has in all interviewed regions;
Resource conservation measure MR1 ’setting global per-capita resource use budgets’ was unanimously considered unthinkable in the current political economy of the regions interviewed. However, having industrialised countries taking the lead by example and providing concessions to developing countries could help.
Measures interviewees from different world regions perceived as most relevant
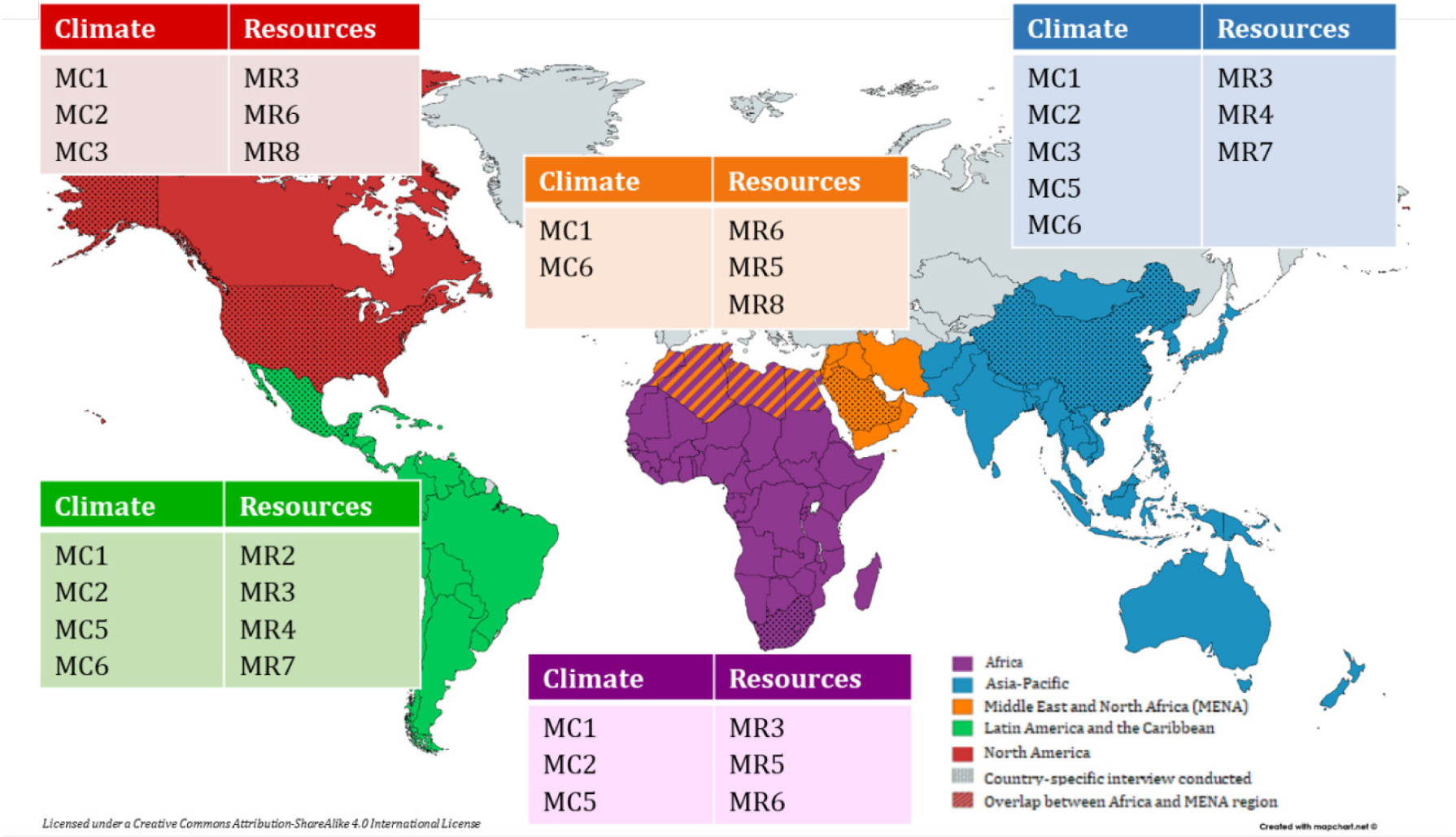
For climate protection, the majority of the interviewees identified the measure ’shifting to 100% renewable energy’ (MC1) as being particularly relevant in their regions. The only exception was an interviewee for the Middle East, who assigned an even higher regional relevance to decarbonising fossils fuels, e.g. shifting from gas or oil to hydrogen or using so-called CO2-enhanced oil recovery (EOR+), and also considered the measure ‘increasing natural carbon sinks’ (MC6) very relevant. The shift to 100% renewable energy is a key measure as it can reduce GHG emissions significantly. However, interviewees regarded full implementation as unrealistic in all explored regions due to the current economic and cultural constraints. Among these are inexpensive and readily available fossil fuels, in particular oil, gas, or coal, as well as infrastructure and political systems poised to perpetuate the use of fossil fuels.
Furthermore, several interviewees considered it a challenge to finance the renewable-energy transition in low- and middle-income countries. It was argued that the electrical grid was rarely built to accommodate a large share of renewables, thus requiring additional infrastructure investments, particularly in Africa. China makes large investments in renewable energy, which drives down costs. However, the country still relies on coal power due to its large and increasing energy needs. South Africa, which is experiencing increasing unemployment, remains attached to coal power despite its decreasing economic feasibility due to its importance as a source of employment and the strong organisation of its employees in unions. In Central America and parts of Asia, the trade-off between land use to produce renewable energies versus food poses a challenge. More specifically, using land to ensure food security for the population has a higher priority than using it for producing renewable energies, for example, solar energy or biofuels.
Interviewees for the regions Africa, AP, and LAC also considered the climate protection measure ‘changing lifestyles and sufficiency’ very relevant, but at the same time very difficult to implement as changing habits and consumption patterns is a challenging field for policy-making. All interviewees above point out a general lack of awareness among the population about changing towards more sustainable life styles. However, some see a change in younger generations towards greater awareness for sustainable life styles and sufficiency, also in the light of increased visibility of climate impacts. One interviewee stated that Mexico experiences a divide between the younger and older generations. For instance, urban young people appear more open to adopting plant-based diets. At the same time, safety issues pose a significant challenge to change consumption patterns. As outside spaces are perceived unsafe in some countries (e.g., in parts of Africa and LAC), many young people retreat to malls and similar recreational areas that are closely linked with consumption. If policymakers want to induce life style changes, they need to offer safe spaces.
Food and sustainable food systems emerged as a central policy area calling for life style changes, both for interviewees covering climate protection and resource conservation. On the one hand, interviewees for NA and LAC report meat-intensive diets inter-linked with tradition and culture, while one interviewee views meat consumption as rising. Interviewees argue that meat consumption in many countries of these three regions either plays a very important role in the local culture (for instance, in the so-called “southern cone” sub-region of LAC, encompassing Argentina, southern Brazil, Chile, Paraguay, and Uruguay), is tied with cultural and religious tradition (in the USA), and tends to be perceived as a status symbol because meat consumption is associated with high income (both for AP and LAC). Here, aiming at reducing meat consumption can face opposition from the public, but also from the meat and dairy industries, which are perceived to have a very strong lobbying power, particularly in NA, but also LAC. Therefore, interviewees argued for shifting towards plant-based diets more aspirational through awareness-raising campaigns without launching a general attack on the meat and dairy industries. According to interviewees in AP, LAC, and NA, there is a growing, albeit still niche, development of shifting towards more plant-based diets due to health and environmental concerns. Therefore, instead of framing policy measures against those who choose to eat meat and promoting giving up meat entirely, plant-based diets should be made a more vital component of general diets, highlighting health effects over the associated climate protection benefits. Here, interviewees considered engaging role models helpful to show that dietary changes can be made, inspiring people towards such changes. One interviewee regarded the support of religious or widely known figures relevant to make plant-based diets more aspirational and culturally acceptable.
Besides meat consumption, food waste issues were also raised. In AP and LAC, food waste is seen as an increasing problem that is strongly related to increasing income and a growing middle class. In AP and MENA, interviewees also saw a cultural component linked to food waste. It is culturally encouraged – linked to virtues such as hospitality and generosity – to have big feasts with a great variety and quantity of food and offer more food than can be eaten. Hence, interviewees perceived a lack of awareness about using only the amount of food needed and called for interventions to change behaviour. Again, making use of role models to support such changes was considered relevant.
Almost all interviewees for resource conservation considered the measure MR3 ‘fostering markets for sustainable products and secondary raw materials’ to be particularly relevant, as it is seen as a clear win-win approach. Increasing the demand for secondary materials is vital, but low capacities and technologies for proper waste management are challenging. Interviewees from LAC, AP, and Africa see the creation of a market for secondary raw materials as having potential positive effects for both the environment and business. However, interviewees also mentioned a variety of challenges for establishing a secondary raw material market for the different regions:
Secondary raw materials tend to be more expensive than virgin materials, mainly due to being difficult to control within LAC and MENA. For instance, the (currently low) price of crude oil and the fluctuation in the global market prices of virgin materials create essential barriers for establishing a market for secondary materials.
A lack of awareness and political will among decision-makers in MENA and Africa, and a lack of capacities and budget in municipalities in charge of waste management, hamper policy implementation and set-up of necessary infrastructures.
A lack or insufficiency of infrastructure, for instance, inadequate systems for waste collection and recovery, and a lack of technology for transforming waste into secondary raw materials (LAC and USA). There is also a lack of investment regarding this infrastructure and related innovation, which keeps production costs of secondary raw materials relatively high. As a result, no market pull would help to match the pricing and product quality of secondary materials.
A lack of awareness among consumers (e.g., what is being thrown away still has a value) and a lack of user-friendly collection systems that could encourage less environmentally aware people to participate in recycling (LAC and USA).
One interviewee stated that fostering the market for secondary raw materials in Africa requires a clear legal and institutional framework and the involvement of the private sector. In this context, demonstration projects are necessary to show the benefits and encourage the adoption of more circular practices by companies, e.g., through government programs that promote a circular economy.
In LAC, possible instruments to increase the demand for sustainable products and secondary raw materials could be, for instance, eco-labelling and putting adequate fiscal policies in place (link to measure MR2 ‘raising the prices of virgin materials’). Furthermore, incentivising innovation and adopting of technologies, e.g., through investment, would also be a suitable approach.
The interviewed expert for AP pointed out that developing official guidelines communicating the benefits of a circular economy and providing guidance on implementing such approaches could support a transition to and establishment of a secondary raw materials market.
For the USA, one interviewee considered it most essential to create incentives for increasing the secondary material content in products. For instance, this could include creating an infrastructure to optimise technology investment, e.g., in the waste separation process, to enable more efficient recovery systems for secondary materials. Such incentives should be created at state and local government levels first, for instance, through pilot projects experimenting and developing approaches suitable for upscaling. Such projects would have to start in states or municipalities with a sound infrastructure system and recycling policies that allow for testing such measures.
For MENA, one interviewee proposes a reduction of the price of sustainable products and secondary raw materials to be achieved through research and development to reduce the cost of these products.
Overall, only six interviewees mentioned interactions between their respective field of environmental policy expertise (either climate protection or resource conservation) and the other environmental policy field. Reactions of the other interviewees to this interview question seem to indicate that the climate-resource-nexus is no (particular) focus of their work or in their professional environments. Hence there is limited expertise on the climate-resource-nexus. Furthermore, interviewees perceive silo-thinking – either for climate protection or resource conservation – as one underlying cause of lacking knowledge on the nexus.
Regarding the six interviewees who mentioned the climate-resource-nexus, their reflections match the critical lines of debate as outlined in the introduction to this paper. Interviewees working in climate protection mainly stated that more efficient use and the recycling of resources also reduces GHG emissions compared to extraction, production, processing, and transport of virgin materials and products. Three interviewees mentioned that a trade-off between climate mitigation and resource conservation exists, as renewable energy production increases the use of (certain) raw materials. For instance, the construction of wind turbines increases the consumption of steel and cement; the installation of photovoltaics – consumption of rare minerals. In this context, interviewees argued that under a lifecycle perspective – which includes sourcing, production, transport, and end-of-life management – the climate protection advantages of renewable energy vs. fossil fuels seem to diminish.
All six interviewees saw all proposed resource conservation measures to have an impact on GHG emissions. However, one interviewee cautioned that the sectoral composition effects of many of the proposed measures should be scrutinised to identify those with the highest benefit for climate protection.
According to the interviewee from NA, for most people, both globally and in the USA, it is not clear that creating a circular economy is a value-add proposition for climate protection. In the interviewee’s view, people do not link climate issues with materials, even though materials use across the value chain is responsible for about half of global GHG emissions. This lack of awareness further complicates the scaling-up of resource conservation measures, where climate co-benefits could provide an additional argument favouring resource conservation. Here, the interviewee argued that introducing a material-specific metric (e.g., metals or food) to make explicit co-benefits for people, the economy, and climate protection could help.
In our view, the results presented above reveal two main findings:
Policy measures focus either on climate protection or resource conservation and circular economy but hardly consider mutual effects and interactions in their design or reasoning.
Regional relevance and fit of policy measures require context-adapted solutions along overarching lines of policy reasoning.
Below, each of these findings is discussed in turn.
It follows from both the literature review and interviews that policy measures hardly consider mutual effects between climate protection and resource conservation or circular economy in their design or reasoning. While the literature review revealed some policy approaches with different levels of detail, studies predominantly look at GHG emission reduction effects of material efficiency policy or at raw material needs of the energy transition. However, among the 19 studies analysed in the literature review, none investigated a policy mix explicitly designed for the climate-resource-nexus, i.e., explicitly combining climate protection with resource conservation measures. This finding is visible in other literature, e.g., in [66] and [67] regarding the UK’s carbon budget and how material productivity and resource efficiency approaches could contribute. Although [66] finds relevant contributions to reducing the UK’s anticipated emissions deficit up to 73% (i.e., extending the time until the budget is used up by several months), there is no integrated policy approach. Instead, scenarios are considered individually. The lack of integration of climate protection and resource conservation might be because the international, European, and national environmental policy does not provide much orientation for researchers to select and assess such policy mixes. Despite the relevance of a low-carbon and circular economy, which has increasingly emerged as an objective of environmental policy in recent years, policies seem to aim for action in several areas in parallel to each other, but without clear interactions between them.
In the interviews, only a few interviewees mentioned issues of the climate-resource-nexus when asked. While looking for experts, it was not possible to find interviewees working on both topics. The experts interviewed were predominantly reluctant to provide answers on the climate-resource-nexus, as they considered the other field (climate or resources) to be beyond their expertise. Some of those who answered that question pointed to silo culture and a lack of cross-departmental policy-making – or policy integration – as an underlying root cause.
Hence, both the literature review and the interviews indicate that policy-making in silos hampers climate-resource-nexus policy. Where policy processes of executive bodies do remain exclusively within sectoral departments or thematic arenas (in ‘policy silos’ [68]), they risk overlooking or ignoring interlinkages with other policy areas. The policy-makers might reduce the risk by integrating robust knowledge of such interlinkages, in particular via cross-departmental interaction, and thus can consider and factor these into their policies [69]. According to [42], revising the Emissions Trading Scheme (ETS) to include material efficiency – one example of considering the climate-resource-nexus in policy-making – will require such cross-departmental and wider stakeholder exchange. Here, robust external knowledge, particularly scientific findings, is essential to identify and design effective climate-resource-nexus policy. However, as scientific findings will always come with a degree of uncertainty, science appears partly at odds with policy-making, which requires concrete policy proposals with (near) certainty [70]. Hence, policy-making needs to weigh different sources of knowledge and different options against each other to create long-term policy strategies with a view to potential policy interactions regarding the climate-resource-nexus (see, e.g., [71]). Yet, political practices and needs, such as existing alliances, election-based tactics, or temporary terms in government, motivate incoming new governments to roll back some of the policies from predecessors, complicate long-term policy strategies and often lead to so-called policy-layering: adding new policy pieces without an overarching strategy [71]. Policy-layering increases the risk of producing trade-offs and neglecting potential interactions with other policy areas [72]. Thus, policy-layering may create mismatches between policy instruments and outcomes as well as conflicting objectives between the different instruments stacked upon each other [73].
It is argued that this highlights how relevant an overarching policy reasoning is to foster policy integration and reduce the risk of policy-layering. Here, thinking along the climate-resource-nexus – or nexus thinking in general – may offer a promising approach. Table 6 summarises relevant issues regarding the integrated climate-resource-nexus policy.
Overview of relevant issues regarding integrated climate-resource-nexus policy
Issue |
Brief description of a relevant issue |
---|---|
Lacking integration |
Findings from this paper show that 1) Resource conservation and material efficiency policy measures help to reduce GHG emissions; 2) Climate protection policy, in particular the energy transition, yields specific raw material needs; 3) Mutual effects and interactions are hardly considered in research, let alone in policy design. => Climate-resource-nexus policy as integrated climate protection and resource conservation policy with combined measures or interaction design does not seem to exist in its own right. |
Siloed policy design |
Climate and resource conservation and material efficiency policy appear as distinctly separate policy areas or even policy silos. Policy-making in silos hampers climate-resource-nexus policy |
Cross-departmental interaction and nexus thinking |
Cross-departmental interaction can facilitate integrated climate-resource-nexus policy-making Nexus thinking can yield overarching, cross-departmental policy reasoning, thus reducing risks of policy-layering and trade-offs between climate and resource policy – and other policy areas. |
An overarching line of policy reasoning also appears relevant to allow policy-making at different levels to adapt policies according to context-specific needs. The interviews show that the relevance of policy measures and their potential fit differ across regions, which is a central finding in the light of many modelling studies that analyse potential global GHG emission reduction effects of resource conservation measures. The model studies on such measures have to make assumptions on which effects the measures may have on resource use in different world regions and often apply policy measures from the European or industrialised country contexts to the global level. These assumptions and the modelling mechanisms of the studies thus limit a detailed differentiation according to different socio-cultural or socio-economic contexts. Moreover, beyond the scientific implications that this approach has regarding sensitivities of modelling findings to different contextual assumptions, it may also lower the relevance or legitimacy of such studies for actors in the Global South and contribute to maintaining the North-South Divide (see, e.g. [74])
Therefore, introducing an overarching line of climate-resource-nexus policy reasoning at the international level could give national and regional policy makers new opportunities. Policies can be designed to fit their respective geographic, socio-economic, and cultural settings while pursuing the overarching line of reasoning. Thus, an overarching line of reasoning ensures overall consistency of policy orientation while enabling to fit concrete policy measures to specific contexts, benefitting perceived policy relevance and thus aiding policy implementation.
In this light, the interview findings show that several areas of policy intervention are relevant across several regions covered by the interviewees – these could thus form part of an overarching line of climate-resource-nexus policy. For all five regions covered, interviewees considered shifting to renewable energies as a relevant measure for climate protection. For three or four regions covered, interviewees assessed increasing electrification, fostering markets for sustainable products and secondary material, changing lifestyles, and reducing plastic waste as relevant. Therefore, it is argued that there is overlap in overall policy orientation regarding what to do to curb GHG emissions and conserve resources. However, the concrete instrument sets considered by the interviewees as relevant to this policy orientation differ depending on the context. For instance, in the MENA region, where fossil fuels are abundant, and energy prices are low (see, e.g., [75]), a milestone or interim step towards shifting to renewable energies seems to be to decarbonise fossil fuel production, e.g., by using more hydrogen or EOR+.
Regional differences in policy design seem particularly relevant for changing lifestyles including dietary choices and the food system. The debate around lifestyle changes regarding food consumption seems to be gaining importance across many different regions, both for resource conservation and climate protection. Actions in this regard are likely to reduce both resource use and GHG emissions, but interventions will need to be sensitive to socio-cultural settings. For instance, religious figures and further relevant role models to encourage people to shift towards less meat-based diets and reduce food quantities provided at festivities may create public support for policy implementation in some regions (e.g., AP; see, e.g., [76]). Furthermore, subsidies for plant-based diets might receive stronger public support than increasing consumption costs of meat and animal-based proteins (e.g., via value-added tax increases). Such a situation is likely to occur in regions where eating meat has a high socio-cultural relevance (e.g. LAC and NA; see, e.g. [77]).
Furthermore, linking higher intake of plant-based foods to positive health effects appears to be a promising strategy in some regional contexts (see, e.g., [78]). Hence, policy design should embrace several motivations which may incentivise and encourage sustainable behaviour beyond environmental reasons. It would then be possible to enhance context relevance and fit while staying within the guardrails that an overarching line of policy reasoning could provide.
Overarching lines of policy reasoning along the climate-resource-nexus seem achievable via cross-departmental exchange on different levels. At the international level, for instance, such lines can be found in the context of the G20 Resource Efficiency Dialogue, the G7 Alliance for Resource Efficiency, and Conferences of Parties to the UNFCCC. For Europe, the framework of implementing the EGD and its environmental policy pillars is available. At the national level, new cross-departmental processes along national sustainability strategies could ensure positive coordination, e.g., via inter-ministerial working groups with appropriate organisational structures and conflict resolution mechanisms [79]. Experience with international environmental conventions (like UNFCCC and UNCBD) shows that differentiated levels of policy ambition and policy pace facilitate context-specific progress, in different countries or regions, towards a common goal. The climate policy approach of contraction and convergence can be seen as an example (see, e.g., [80]).
Therefore, an overarching line of reasoning for climate-resource-nexus policy should follow this approach and enable regional differences in implementation. International standards as globally binding rules seem particularly relevant regarding adverse social-ecological effects along value chains for low-carbon technologies to protect vulnerable communities from the environmental hazards related to material extraction or waste generation (e.g., regarding battery raw materials and e-waste, see [40]).
The assessment of the regional relevance and fit of policy measures offered insights into potential socio-cultural and socio-economic implications of policy needs in the context of the climate-resource-nexus in different regions. As there is no specific, clear-cut policy for the climate-resource-nexus, scientific studies should further investigate potential impacts and relevant correlations between regional approaches for GHG emission reductions and material efficiency. With climate-resource-nexus policy hopefully emerging step-by-step, researchers could then scientifically assess its possible effects on a regional level as well. Additional research findings for local or city levels could enable a better understanding of the relevance of climate-resource-nexus policy designed at local and regional levels and its compatibility with an overarching policy reasoning – and what this means for supranational, overall policy reasoning. For the latter, the participation of experts and policy-makers from both fields would be essential for future research to understand issues of silo culture better and identify ways of bridging silos at different levels.
Expanding the set of regional experts’ views on the climate-resource-nexus and potential effects of policy measures appears crucial to complement the current exploratory study approach. With thirteen interviews overall, our study can only provide a first qualitative overview of regional policy perspectives of the climate-resource-nexus and the relevance of overcoming silo culture. Implementing a quantitative methodological approach based on testing hypotheses across many participants will help to remove elements of subjectivity present in the exploratory approach. For instance, in the interviewees’ responses, one could not discern whether an interviewee perceived a policy measure as relevant because it supports the achievement of environmental policy goals or because it is potentially feasible in the context of the region. However, identifying many study participants may meet with similar difficulties to our approach: as disciplinary expertise seems to prevail, i.e., either on climate protection or on circular economy and resource conservation, climate-resource-nexus experts might be difficult to find – at least as of now.
The recent increase in scientific nexus studies, increasing relevance of nexus thinking in academia, and the encompassing nature of international and European policy seem promising to expand and build such expertise. As relevant examples, the group of independent research initiatives labelled the Nexus Project Cluster† and the Sustainable Development Goals, and the European Green Deal EGD can be named. For instance, the EGD from December 2019 assumes achieving climate neutrality in 2050 by integrating a diverse set of environmental policies – including ones for biodiversity, circular economy, construction, energy, food, industry, and mobility. However, according to its roadmap of key actions (the Annex to the EGD), implementing the EGD still requires sectoral environmental policy actions, which seem to have to happen in parallel. These processes and implementation steps will have to prove that the EGD can operationalise nexus thinking in environmental policy – including, but not limited to, the climate-resource-nexus. Learning from the approaches developed at the EU level through the EGD could inspire climate-resource-nexus exchange processes at the international level, e.g., regarding G7 and G20.
Hence, ongoing policy processes could yield the necessary silo-bridging expertise and capacities alongside an increasing scientific focus on the climate-resource-nexus. Creating an overarching line of reasoning for an international climate-resource-nexus policy depends on different regional implementation pathways.
Detailed overview of policy measures that selected scientific publications address in the context of the climate-resource-nexus; last name author A – D
Publication (ordered alphabetically for author’s last name) |
Policy measures addressed |
Specific sectoral or product coverage |
---|---|---|
Aracil et al. 2017 [45] |
Assessing potential climate mitigation potentials of biofuels produced from the non-recyclable fraction of municipal solid waste (MSW); arguing that with resource efficiency measures, i.e. a progressive landfill banning in Europe, MSW-based biofuels will yield clear climate benefits. |
Waste management, biofuels |
Barrett and Scott 2012 [35] |
Analysing links between dematerialisation and climate change mitigation in the UK regarding a wide range of material efficiency strategies; finding greatest GHG emission reduction potential for the consumption-side strategies dietary changes (reducing animal-based food products), extending product lifetimes and improving durability, lifetime optimisation and shifting from owning products to using services; on the production-side lean production (e.g. lightweighting), waste reduction throughout supply chains, material substitution and waste recycling show greatest GHG emission reduction potentials. |
(No specific sectoral coverage) |
Bonsu 2020 [40] |
Investigating potential end-of-first-life applications of electric vehicle Lithium-Ion-Batteries (LIBs) in a net zero economy; arguing that policy should foster business model design as well as product and service design for circularity, create enabling legislation, provide rewards or incentives for circular solutions; environmental and social standards and certification for battery raw materials needed to protect vulnerable populations from extraction related impacts as well as from end-of-life batteries ending up as e-waste in poor economies. |
(Electric) Vehicles |
Distelkamp and Meyer 2019 [32] |
Modelling global GHG emission reduction effects of different scenarios, including resource efficiency approaches (e.g. upstream tax on metal ores and non-metallic minerals, mandatory recycling quotas and information instruments). |
(No specific sectoral coverage) |
Dienst et al. 2015 [46] |
Analysing potential pathways for a low carbon future of the industrial city of Wuxi, China; arguing that resource efficiency approaches are needed alongside climate protection strategies in order to achieve an overall reduction in resource use (such as steel and cement), thus achieving per capita CO2 emission levels, which comply with the 2 °C target. |
Energy sector, manufacturing industry, transport; steel and cement production |
Detailed overview of policy measures that selected scientific publications address in the context of the climate-resource-nexus; last name author E – G
Publication (ordered alphabetically for author’s last name) |
Policy measures addressed |
Specific sectoral or product coverage |
---|---|---|
Ellen MacArthur Foundation 2019 [3] |
Investigating the potential of circular economy approaches to contribute towards achieving global climate targets; arguing that circular economy strategies – in particular recirculating materials (e.g. recycling), re-using products and components (e.g. remanufacturing), eliminating waste (e.g. by changing product design and extending product lifetimes) and regenerative agriculture – will support climate change mitigation alongside fostering value creation and distribution, innovation and societal well-being. |
Aluminium, cement, food, plastics and steel; agriculture, construction, mobility |
Fang et al. 2017 [29] |
Analysing potential effects of circular economy strategies on carbon footprints in the Chinese city of Guiyang; finding that substituting cement with aluminium, phosphorous and steel slag provides most carbon footprint reductions of all urban industrial symbiosis activities considered (in addition recycling of plastic, steel and tire waste; utilising waste heat between industrial sectors, for urban areas and the agriculture sector). |
Agriculture and forestry; industry; construction; transportation, storage and postal service; whole sales and retail |
Gallego-Schmid et al. 2020 [47] |
Investigating links between circular economy approaches and climate mitigation in the built environment; arguing that embodied emissions must be reduced on the consumption side, for instance by increasing durability and reusability of materials and products, and substituting materials, e.g. for wood. |
Buildings and construction |
Gonzalez Hernandez et al. 2018 [42] |
Discussing the role of material efficiency approaches for achieving carbon emission reductions in heavy industries (including industry standards on material efficiency, industry-led material efficiency initiatives, tax reliefs to encourage material efficiency, redesign of the ETS emissions cap by allowing capturing material efficiency improvements in calculation of annual emission reduction rates). |
Heavy industries |
Detailed overview of policy measures that selected scientific publications address in the context of the climate-resource-nexus; last name author H – R
Publication (ordered alphabetically for author’s last name) |
Policy measures addressed |
Specific sectoral or product coverage |
---|---|---|
Hatfield-Dodds et al. 2017 [31] |
Modelling global GHG emission reduction effects of a mix of resource efficiency measures (inter alia, industrial material savings though technical improvements, resource extraction taxes, as well as regulation and green public procurement). |
(No specific sectoral coverage) |
Hertwich et al. 2019 [26] |
Reviewing potential global GHG emission reductions associated with material efficiency strategies, such as light-weighting, product lifetime extension, re-use, remanufacturing and repair as well as material substitution and recycling; arguing that lifetime extension, light-weighting and – in construction: substituting for timber – show greatest GHG emission reduction potential, there might also be relevant trade-offs, e.g. regarding the potential supply of timber. |
Buildings and construction, electronics, vehicles |
Huang et al. 2013 [48] |
Estimating raw material demand and environmental impacts associated with buildings and building construction in China; finding lifetime extension of buildings and improved materials recycling to significantly reducing material (iron ore and limestone) demand, solid waste generation and CO2 emissions. |
Buildings and construction |
Material Economics 2018 [34] |
Exploring potentials of circular economy and material efficiency approaches for reducing GHG emissions from material production and use segments; concluding that supply-side and demand-side circular approaches (material recirculation, product material efficiency and circular business models) show the potential for significant GHG emission reductions from heavy industry. |
Aluminium, plastics, steel; buildings and construction, mobility/passenger cars |
Meyer et al. 2018 [39] |
Modelling global GHG emission reduction effects of policy supporting technology improvements in resource-relevant production sectors to foster resource efficiency. |
(No specific sectoral coverage) |
Nußholz et al. 2019 [41] |
Investigating potentials of lower-impact building materials with secondary material input to reduce embodied GHG emissions of building materials; policy issues include, inter alia, mandatory pre-demolition audits and selective demolition, quality standards for secondary materials, resource conservation criteria in building codes and public procurement processes. |
Buildings and construction |
Röck et al. 2020 [49] |
Analysing GHG emissions embodied in buildings; suggesting building requirements and policies require to integrate embodied GHG emissions via life cycle performance benchmarks and by fostering the decarbonisation of construction material manufacturing, including end-of-life treatment of construction materials. |
Buildings and construction |
Detailed overview of policy measures that selected scientific publications address in the context of the climate-resource-nexus; last name author S – V
Publication (ordered alphabetically for author’s last name) |
Policy measures addressed |
Specific sectoral or product coverage |
---|---|---|
Shen et al. 2021 [50] |
Analysing CO2 emissions from cement production in China; arguing that energy efficiency improvements will need to be combined with policy aimed at increasing resource efficiency and material substitution in order to counteract increasing emissions from rapid urbanisation. |
Buildings and construction, cement production |
Turner et al. 2016 [43] |
Combining material flow analysis and life cycle assessment to assess GHG emission generation associated with solid waste management; arguing that waste prevention, diversion of food waste from residual waste stream and waste recycling together form the potentially most effective strategy to reduce GHG emissions. |
Waste management |
van der Voet et al. 2019 [44] |
Developing and applying a method for assessing environmental impacts associated with different scenarios of supply and demand for seven major metals; finding that increasing the share of secondary production proves an effective approach to reducing GHG emissions. |
Metals (aluminium, copper, iron, lead, manganese, nickel and zinc) |
Emissions Gap Report 2020 ,United Nations Environment Programme , 2020
, - , Resource Efficiency and Climate Change: Material Efficiency Strategies for a Low-Carbon Future, A Report of the International Resource Panel, 2020
, Completing the Picture: How the Circular Economy Tackles Climate Change. , 2019
, Bridging the gap between circular economy and climate change mitigation policies through eco-innovations and Quintuple Helix Model ,Technol Forecast Soc Change , Vol. 160 ,pp 120246 , 2020, https://doi.org/https://doi.org/10.1016/j.techfore.2020.120246
, The Development of the Water-Energy-Food Nexus as a Framework for Achieving Resource Security: A Review ,Front Environ Sci. , Vol. 7 ,pp 8 , 2019, https://doi.org/https://doi.org/10.3389/fenvs.2019.00008
, - , , In: Climate Change 2014: Impacts, Adaptation, and Vulnerability Part A: Global and Sectoral Aspects. Contribution of Working Group II to the Fifth Assessment Report of the Intergovernmental Panel on Climate Change, 2014
10 Key Message on Climate Change ,International Resource Panel , 2015
, Resource Efficiency: Potential and Economic Implications ,A Report of the International Resource Panel , 2017
, Resource Efficiency: Potential and Economic Implications ,Summary for Policy-Makers , 2016
, , Toyama Framework on Material Cycles , 2016
, First G20 Resource Efficiency Dialogue Meeting Takes Place in Berlin ,Joint Press Release , 2017
, Global metal flows in the renewable energy transition: Exploring the effects of substitutes, technological mix and development ,Energy Policy , Vol. 119 ,pp 226–241 , 2018, https://doi.org/https://doi.org/10.1016/j.enpol.2018.04.056
, Energy modeling approach to the global energy-mineral nexus: Exploring metal requirements and the well-below 2 °C target with 100 percent renewable energy ,Appl Energy , Vol. 225 ,pp 1158–1175 , 2018, https://doi.org/https://doi.org/10.1016/j.apenergy.2018.05.047
, Metal requirements of low-carbon power generation ,Energy , Vol. 36 (9),pp 5640-5648 , 2011, https://doi.org/https://doi.org/10.1016/j.energy.2011.07.003
, Climate change and sustainability as drivers for the next mining and metals boom: The need for climate-smart mining and recycling ,Resour Policy , , https://doi.org/https://doi.org/10.1016/j.resourpol.2018.05.016
, Metal supply constraints for a low-carbon economy? ,Resour Conserv Recycl , Vol. 129 ,pp 202–208 , 2018, https://doi.org/https://doi.org/10.1016/j.resconrec.2017.10.040
, Energy-material nexus: The impacts of national and international energy scenarios on critical metals use in China up to 2050 and their global implications ,Energy , Vol. 180 ,pp 903–917 ,
, Assessing the short-to medium-term supply risks of clean energy minerals for China ,J Clean Prod. , Vol. 215 ,pp 217–225 , 2019, https://doi.org/https://doi.org/10.1016/j.jclepro.2019.01.064
, Integrated lifecycle assessment of electricity-supply scenarios confirms global environmental benefit of low-carbon technologies ,Proc Natl Acad Sci. , Vol. 112 (20),pp 6277–6282 , 2015, https://doi.org/https://doi.org/10.1073/pnas.1312753111
, Analysis of Potential for Critical Metal Resource Constraints in the International Energy Agency’s Long-Term Low-Carbon Energy Scenarios ,Minerals , Vol. 8 (4),pp 156 , 2018, https://doi.org/https://doi.org/10.3390/min8040156
, Role of critical metals in the future markets of clean energy technologies ,Renew Energy , Vol. 95 ,pp 53–62 , 2016, https://doi.org/https://doi.org/10.1016/j.renene.2016.03.102
, Dynamic analysis of the global metals flows and stocks in electricity generation technologies ,J Clean Prod , Vol. 59 ,pp 260–273 , 2013, https://doi.org/https://doi.org/10.1016/j.jclepro.2013.07.003
, Copper demand, supply, and associated energy use to 2050 ,Glob Environ Change , Vol. 39 ,pp 305–315 , 2016, https://doi.org/https://doi.org/10.1016/j.gloenvcha.2016.06.006
, Assessing the need for critical minerals to shift the German energy system towards a high proportion of renewables ,Renew Sustain Energy Rev. , Vol. 49 ,pp 655–671 , 2015, https://doi.org/https://doi.org/10.1016/j.rser.2015.04.070
, Environmental impacts of high penetration renewable energy scenarios for Europe ,Environ Res Lett , Vol. 11 (1),pp 014012 , 2016, https://doi.org/https://doi.org/10.1088/1748-9326/11/1/014012
, Material efficiency strategies to reducing greenhouse gas emissions associated with buildings, vehicles, and electronics-a review ,Environ Res Lett , Vol. 14 (4),pp 043004 , 2019, https://doi.org/https://doi.org/10.1088/1748-9326/ab0fe3
, The Environmental Impacts of Reuse: A Review: The Environmental Impacts of Reuse: A Review ,J Ind Ecol , Vol. 21 (1),pp 38–56 , 2017, https://doi.org/https://doi.org/10.1111/jiec.12388
, Providing an economy-wide monitoring framework for the circular economy in Austria: Status quo and challenges ,Resour Conserv Recycl. , Vol. 137 ,pp 156–166 , 2018, https://doi.org/https://doi.org/10.1016/j.resconrec.2018.05.022
, Carbon footprints of urban transition: Tracking circular economy promotions in Guiyang, China ,Ecol Model. , Vol. 365 ,pp 30–44 , 2017, https://doi.org/https://doi.org/10.1016/j.ecolmodel.2017.09.024
, Low carbon lifestyles: A framework to structure consumption strategies and options to reduce carbon footprints ,J Clean Prod. , Vol. 139 ,pp 1033–1043 , 2016, https://doi.org/https://doi.org/10.1016/j.jclepro.2016.08.154
, Assessing global resource use and greenhouse emissions to 2050, with ambitious resource efficiency and climate mitigation policies ,J Clean Prod. , Vol. 144 ,pp 403–414 , 2017, https://doi.org/https://doi.org/10.1016/j.jclepro.2016.12.170
, Pathways to a Resource-Efficient and Low-Carbon Europe ,Ecol Econ. , Vol. 155 ,pp 88–104 , 2019, https://doi.org/https://doi.org/10.1016/j.ecolecon.2017.07.014
, Exploring resource efficiency for energy, land and phosphorus use: Implications for resource scarcity and the global environment ,Glob Environ Change. , Vol. 36 ,pp 21–34 , 2016, https://doi.org/https://doi.org/10.1016/j.gloenvcha.2015.09.016
, The Circular Economy – a Powerful Force for Climate Mitigation ,Material Economics Sverige AB , 2018
, Link between climate change mitigation and resource efficiency: A UK case study ,Glob Environ Change , Vol. 22 (1),pp 299–307 , 2012, https://doi.org/https://doi.org/10.1016/j.gloenvcha.2011.11.003
, European policy responses to climate change: progress on mainstreaming emissions reduction and adaptation ,Reg Environ Change , Vol. 15 (6),pp 949–959 , 2015, https://doi.org/https://doi.org/10.1007/s10113-015-0801-6
, A Systemic and Systematic Approach to the Development of a Policy Mix for Material Resource Efficiency ,Sustainability , Vol. 8 (4),pp 373 , 2016, https://doi.org/https://doi.org/10.3390/su8040373
, Advancing to a Circular Economy: three essential ingredients for a comprehensive policy mix ,Sustain Sci , Vol. 13 (3),pp 861–878 , 2018, https://doi.org/https://doi.org/10.1007/s11625-017-0502-9
, Contemporary Resource Policy and Decoupling Trends-Lessons Learnt from Integrated Model-Based Assessments ,Sustainability , Vol. 10 (6),pp 1858 , 2018, https://doi.org/https://doi.org/10.3390/su10061858
, Towards a circular and low-carbon economy: Insights from the transitioning to electric vehicles and net zero economy ,J Clean Prod , Vol. 256 ,pp 120659 , 2020, https://doi.org/https://doi.org/10.1016/j.jclepro.2020.120659
, Circular building materials: Carbon saving potential and the role of business model innovation and public policy ,Resour Conserv Recycl. , Vol. 141 ,pp 308–316 , 2019, https://doi.org/https://doi.org/10.1016/j.resconrec.2018.10.036
, Leveraging material efficiency as an energy and climate instrument for heavy industries in the, EU ,Energy Policy , Vol. 120 ,pp 533–549 , 2018, https://doi.org/https://doi.org/10.1016/j.enpol.2018.05.055
, Combined material flow analysis and life cycle assessment as a support tool for solid waste management decision making ,J Clean Prod. , Vol. 129 ,pp 234–248 , 2016, https://doi.org/https://doi.org/10.1016/j.jclepro.2016.04.077
, Environmental Implications of Future Demand Scenarios for Metals: Methodology and Application to the Case of Seven Major Metals ,J Ind Ecol , Vol. 23 (1),pp 141–155 , 2019, https://doi.org/https://doi.org/10.1111/jiec.12722
, Proving the climate benefit in the production of biofuels from municipal solid waste refuse in Europe ,J Clean Prod. , Vol. 142 ,pp 2887–2900 , 2017, https://doi.org/https://doi.org/10.1016/j.jclepro.2016.10.181
, Wuxi – a Chinese City on its Way to a Low Carbon Future ,J Sustain Dev Energy Water Environ Syst , Vol. 3 (1),pp 12–25 , 2015
, Links between circular economy and climate change mitigation in the built environment ,J Clean Prod , Vol. 260 ,pp 121115 , 2020
, Materials demand and environmental impact of buildings construction and demolition in China based on dynamic material flow analysis ,Resour Conserv Recycl. , Vol. 72 ,pp 91–101 , 2013, https://doi.org/https://doi.org/10.1016/j.resconrec.2012.12.013
, Embodied GHG emissions of buildings – The hidden challenge for effective climate change mitigation ,Appl Energy , Vol. 258 ,pp 114107 , 2020, https://doi.org/https://doi.org/10.1016/j.apenergy.2019.114107
, Energy-Cement-Carbon Emission Nexus and its Implications for Future Urbanization in China ,J Sustain Dev Energy Water Environ Syst , Vol. 9 (2),pp 1–15 , 2021, https://doi.org/https://doi.org/10.13044/j.sdewes.d8.0354
, Global patterns of national climate policies: Analyzing 171 country portfolios on climate policy integration ,Environ Sci Policy , Vol. 84 ,pp 177–185 , 2018, https://doi.org/https://doi.org/10.1016/j.envsci.2018.03.003
, Policy developments for the reduction of climate change impacts by the transportation sector ,Transp Policy , Vol. 61 ,pp 36–50 , 2018
, , In: Climate Change 2014: Mitigation of Climate Change. Contribution of Working Group III to the Fifth Assessment Report of the Intergovernmental Panel on Climate Change , 2014
, Mitigating greenhouse gas emissions through replacement of chemical fertilizer with organic manure in a temperate farmland ,Sci Bull. , Vol. 60 ,pp 598–606 , 2015
, It starts at home? Climate policies targeting household consumption and behavioral decisions are key to low-carbon futures ,Energy Res Soc Sci. , Vol. 52 ,pp 144–158 , 2019
, Pricing forest carbon: Implications of asymmetry in climate policy ,J For Econ. , Vol. 32 ,pp 84–93 , 2018
, Per capita resource consumption and resource carrying capacity: A comparison of the sustainability of 17 mainstream countries ,Energy Policy. , Vol. 42 ,pp 603–612 , 2012
, Key drivers for unsustainable resource use – categories, effects and policy pointers ,J Clean Prod. , Vol. 132 ,pp 13–31 , 2016, https://doi.org/https://doi.org/10.1016/j.jclepro.2015.02.038
, Policies for transitioning towards a circular economy: Expectations from the European Union (EU) ,Resour Conserv Recycl , Vol. 155 ,pp 104634 , 2020
, From Waste Management to Resource Efficiency-The Need for Policy Mixes ,Sustainability , Vol. 8 (7),pp 622 , 2016
, Pathways to circular construction: An integrated management of construction and demolition waste for resource recovery ,J Clean Prod , Vol. 244 ,pp 118710 , 2020, https://doi.org/https://doi.org/10.1016/j.jclepro.2019.118710
, Sustainable food transition in Portugal: Assessing the Footprint of dietary choices and gaps in national and local food policies ,Sci Total Environ , Vol. 749 ,pp 141307 , 2020, https://doi.org/https://doi.org/10.1016/j.scitotenv.2020.141307
, The Circular Economy and Benefits for Society ,A Study Pertaining to Finland, France, the Netherlands, Spain and Sweden. A Study Report at the Request of the Club of Rome with Support from the MAVA Foundation , 2015
, Regional innovation governance and place-based policies: design, implementation and implications ,Reg Stud Reg Sci , Vol. 6 (1),pp 101–116 , 2019, https://doi.org/https://doi.org/10.1080/21681376.2019.1578257
, A conceptual model to integrate the regional context in landscape policy, management and contribution to rural development: Literature review and European case study evidence ,Geoforum , Vol. 82 ,pp 1–12 , 2017, https://doi.org/https://doi.org/10.1016/j.geoforum.2017.03.012
, Bridging the climate mitigation gap with economy?wide material productivity ,J Ind Ecol , Vol. 23 (4),pp 918–931 , 2019, https://doi.org/https://doi.org/10.1111/jiec.12831
, Public acceptance of resource-efficiency strategies to mitigate climate change ,Nat Clim Change , Vol. 8 (11),pp 1007–1012 , 2018, https://doi.org/https://doi.org/10.1038/s41558-018-0298-3
, Silo-Busting: Overcoming the Greatest Threat to Organizational Performance ,Sustainability , Vol. 11 (23),pp 6860 , , https://doi.org/https://doi.org/10.3390/su11236860
, Breaking out of silos: explaining cross-departmental interactions in two European bureaucracies ,J Eur Public Policy , , https://doi.org/https://doi.org/10.1080/13501763.2020.1784253
, Decision-Making Under Scientific Uncertainty: The Economics of the Precautionary Principle ,J Risk Uncertain , Vol. 27 (1),pp 77–103 , 2003
, Beyond Good and Evil in Policy Implementation: Instrument Mixes, Implementation Styles, and Second Generation Theories of Policy Instrument Choice ,Policy Soc , Vol. 23 (2),pp 1–17 , 2004, https://doi.org/https://doi.org/10.1016/S1449-4035(04)70030-2
, Design Principles for Policy Mixes: Cohesion and Coherence in ‘New Governance Arrangements.’ ,Policy Soc , Vol. 26 (4),pp 1–18 , 2007, https://doi.org/https://doi.org/10.1016/S1449-4035(07)70118-2
, Beyond the “Tinbergen Rule” in Policy Design: Matching Tools and Goals in Policy Portfolios ,SSRN Electron J , 2013, https://doi.org/https://doi.org/10.2139/ssrn.2247238
, Bridging the North-South Divide: International Environmental Law in the Anthropocene ,Pace Environ Law Rev , Vol. 32 ,pp 407 , 2015
, A review and assessment of energy policy in the Middle East and North Africa region ,Energy Policy , Vol. 102 ,pp 249–269 , 2017, https://doi.org/https://doi.org/10.1016/j.enpol.2016.12.023
, Vegetarianism as a social identity ,Curr Opin Food Sci. , Vol. 33 ,pp 45–51 , 2020, https://doi.org/https://doi.org/10.1016/j.cofs.2019.12.005
, How to transition to reduced-meat diets that benefit people and the planet ,Sci Total Environ , Vol. 718 ,pp 137208 , 2020
, A Comprehensive Review of the Benefits of and the Barriers to the Switch to a Plant-Based Diet ,Sustainability , Vol. 12 (10),pp 4136 , 2020, https://doi.org/https://doi.org/10.3390/su12104136
, Inter-Ministerial Working Groups as a Panacea for Coordination Problems? ,Mod Staat – Z Für Public Policy Recht Manag , Vol. 9 (1),pp 65–81 , 2016, https://doi.org/https://doi.org/10.3224/dms.v9i1.23641
, Contraction and convergence: an assessment of the CCOptions model ,Clim Change , Vol. 91 (3-4),pp 275–290 , 2008, https://doi.org/https://doi.org/10.1007/s10584-008-9468-z
,