The Sustainable Development Goals (SDGs) are a universal call to action to end poverty, protect the environment and climate, and ensure that people everywhere can enjoy peace and prosperity [1]. For the countries to achieve the SDGs, different sectors should participate in this quest. Considering that the power sector is one of the engines of a country's development, it is also responsible for participating in this pursuit. Implementing the SDG philosophy in the energy sector is a significant challenge for achieving efficient and sustainable production systems [2]. Access to electricity is increasingly recognised as a critical enabler of economic growth and poverty reduction in developing countries, driving economic and social development by enhancing productivity and enabling new types of job-creating enterprises [3]. According to Mastoi et al. [4], renewable energy is currently argued as the most prominent solution to environmental pollution, the energy crisis, and social sustainability, being also key element to support sustainable development and a social contributor to people living in isolated communities [5].
While many studies have focused on the environmental and economic assessment of power generation [6]–[9], or power generation related [10], fewer articles address the social life-cycle performance of energy supply systems [11]–[14]. A clear research gap exists in considering social issues in such assessments. Despite deep decarbonisation being a critical pillar in the power sector for a carbon-neutral energy system, its socioeconomic benefits remain unexplored [15]. In this context, Social Life Cycle Assessment (S-LCA) emerges as a tool to evaluate the social aspects associated with the life cycle of goods and services and to identify the hotspots of social risks in the energy value chain [16]. Life Cycle Assessment (LCA) is the compilation and evaluation of inputs, outputs, and the potential environmental impacts of a product system throughout its life cycle [17]. Hence, S-LCA can be considered a methodology to assess the social impacts of products and services across their life cycle, employing the Environmental Life Cycle Assessment (E-LCA) combined with social sciences methods [18]. Additionally, S-LCA has linkages with international initiatives and can monitor progress in ten SDGs (especially SDGs 8 and 12) [2].
The utilisation of S-LCA as a social sustainability assessment tool is still being developed due to the complex nature of social impacts [19]. Currently, those impacts are understood as the positive or negative consequences of the causal relationship between an activity and an aspect relating to human well-being, as covered by impact subcategories [18]. These subcategories must, indirectly, be related to the stakeholders, i.e., individuals or group that has an interest in any decision or activity of an organisation [20], while the stakeholder category is a cluster of stakeholders having common interests due to their similar relationship to the investigated product system [18]. The main stakeholder categories considered in the S-LCA are Workers, Local communities, Value chain actors (e.g., suppliers), Consumers, Children, and Society. Because impact categories are broad themes, a life-cycle initiative project group created by UNEP/SETAC in 2004 has focused its initial effort on identifying and building consensus around subcategories that describe more precisely social areas of interest [21]. Social and socio-economic subcategories of impact have been defined according to international agreements and international best practices, presented in Table 1 and published in the Guidelines for Social Life Cycle Assessment of Products [22]. In Table 1, Fair Salary, a subcategory of the impact category Working Conditions, relates to SDGs 1 and 8 [18].
A Fair Wage is a topic that influences all stakeholder groups identified within the S-LCA guidelines. However, studies considering the wage issue are rare in the electricity sector in S-LCA scopes. Fortier et al. [23] discuss how social LCA can address energy justice for stakeholder categories across the life cycle of electrical energy systems and analyse whether wages are docked by companies for reasons beyond a worker's control, wage gaps between sex, gender, nationality, and race; and the percentage of workers earning a living wage based on their location. Traverso et al. [24] report the sustainability assessment of the assembly step of photovoltaic (PV) modules production by Life Cycle Sustainability Assessment (LCSA) and included indicators like the average wage of male and female workers and the minimum wage of a worker. Contreras et al. [25] assessed the impacts of the bagasse cogenerated bioelectricity using LCSA and encompassed, among the indicators, Lowest Paid Workers, compared to the country's Minimum Wage. Prasara-A et al. [26] identify the environmental, socioeconomic, and social hotspots of products within the Thai sugar industry (e.g., bagasse-based electricity) using LCA and S-LCA, including the indicators Range of Wage Received by Workers, and Percentage of Workers Satisfied with Wage.
Stakeholder categories and subcategories [21]
Stakeholder categories | Subcategories |
---|---|
Stakeholder “worker” | Freedom of association and collective bargaining |
Child labour | |
Fair salary | |
Working hours | |
Forced labour | |
Equal opportunities/discrimination | |
Health and safety | |
Social benefits/social security | |
Stakeholder “consumer” | Health and safety |
Feedback mechanism | |
Consumer privacy | |
Transparency | |
End of life responsibility | |
Stakeholder “local community” | Access to material resources |
Access to immaterial resources | |
Delocalisation and migration | |
Cultural heritage | |
Safe and healthy living conditions | |
Respect of indigenous rights | |
Community engagement | |
Local employment | |
Secure living conditions | |
Stakeholder “society” | Public commitments to sustainability issues |
Contribution to economic development | |
Prevention and mitigation of armed conflicts | |
Technology development | |
Corruption | |
Value chain actors (excluding “consumers”) | Fair competition |
Promoting social responsibility | |
Supplier relationships | |
Respect of intellectual property rights |
Considering this background, a Fair Wage is a concept that goes beyond the notion of a minimum wage enabling needs satisfaction and including the fair remuneration of work according to its quality [27]. It has already been listed as one of the meaningful aspects to be considered in assessing labour rights and decent working conditions, being highly relevant for the future development of human beings and, consequently, of regions and countries, as the basis for prosperity and wealth [28].
A “fair” remuneration along the life cycle of a product can serve as one powerful measure to estimate related social impacts on involved workers. In this context, Neugebauer et al. [29] proposed Fair Wage as a new midpoint impact category and developed a characterisation model to convert inventory data on workers' remuneration along a product's life cycle into category indicator results, creating the Fair Wage Potential (FWP) indicator. FWP considers the actual wage paid at each process step, compared to a minimum living wage, and relates wage to the effective working time, including a factor to account for income inequalities.
The method proposed by Neugebauer et al. [29] is a distance-to-target impact pathway [18] and can be summarised according to eq. (1):
(1)
where FWPn is the Fair Wage Potential (expressed in FWeq) representing the nth process within a product's life cycle at a defined location or sector; RWn is the Real (average) Wages (€/month calculated over one year), which are paid to the worker(s) employed in the nth process; MLWn is the Minimum Living Wage (€/month), which has to be paid to the worker to enable an adequate living standard for an individual and/or family in the respective country or region/sector where the nth process is performed; CWTn is the Contracted Working Time per country or sector (hours/week) for workers performing the nth process (including vacation days); RWTn is the Real Working Time (hours/week) of workers performing the nth process (including vacation days and unpaid overtime); IEFn is the (squared) Inequality Factor (expressed in percentage) of the organisation region, country or sector, where the nth process is performed. For RWn and MLWn, the national currencies are used in eq. (1). FWP depends on mainly three country/region-specific and/or product-specific parameters: 1) living wages, 2) working time, and 3) income (in-)equality [18].
If the RWn value is smaller than the MLWn value, the resulting FWPn will be < 1; hence the greater the distance from the (minimum) targeted state, the lower the FWPn value is. Also, if the Real Working Time is equal to the CWT value, then no effect on the FWPn occurs. On the other hand, if the RWT value is greater than the CWT value (which indicates overtime work), the resulting FWPn will also be < 1 (smaller FWPn values indicate more overtime the worker does). A FWPn equal to 1 (one) is the reference value for determining a Fair Wage; values >1 mean the salary is fair. An accumulation of FWP values <1 may indicate regular annual underpayment [29]. Thus, a determined distance from Fair Wage is a category indicator for the impact category Fair Wage. Excessive working hours may additionally contribute to cases of underpayment through time lost to replace the lack of income.
The methodology by Neugebauer et al. [29] allows for consistently determining Fair Wage impacts along a product's life cycle. However, the characterisation model does not foresee a direct relation to the functional unit. Vitorio Junior & Kripka [30] propose a weighted Fair Wage Potential method to assess building typology and relate material inventory to the social data of the construction sector. However, a knowledge gap remains in the methodology to assess the energy sector, linking the FWP to electricity production.
The present work aims at fulfilling this gap by proposing an Employment-Weighted Fair Wage Potential (E-WFWP) indicator based on the characterisation model presented by Neugebauer et al. [29]. It differs from the existing social assessment methods by relating the electricity production alternatives to social data, allowing the consideration of social aspects in selecting the best choice among a set of analysed options. Additionally, the study performs an S-LCA of ten power generation technologies, considering their E-WFWP to identify the wage situation of workers involved in the electricity system, searching for social hotspots (well-being threats). It proposes and applies a decision-support indicator based on Fair Wages, in alignment with SDGs 1 and 8, underpinned by a life cycle approach.
This section presents the premises for selecting electricity technologies, the S-LCA parameters, and the data-gathering procedure. The methodology used is described as follows.
The main electricity generation technologies currently in use worldwide are solar photovoltaic (solar PV), large hydropower plants (reservoir), small hydropower plants (Run-of-River – R-o-R), onshore wind, offshore wind, oil, gas, coal, nuclear, and biomass (biogas).
S-LCA presents a systematic assessment process like that of the E-LCA. This subsection presents the definition of objective and scope, life cycle inventory, and premises.
The present S-LCA aims to assess the potential of alternative power generation technology to offer a Fair Wage to the workers' category along the life cycle of electricity generation. The Functional Unit (FU) is 1 TWh of produced electricity. The scope of the power plant analysis is cradle-to-grave, and encompasses the stages presented by Rutovitz et al. [31], i.e., the power station construction and installation, manufacturing of parts, operation & maintenance (O&M), decommissioning, and fuel extraction and processing.
A product system is a collection of unit processes with elementary and product flows, performing one or more defined functions, and which models the life cycle of a product [32]. Figure 1 presents the product system of the study, as well as its system boundary. It can be observed that the extraction of primary resources and waste treatment and disposal are outside the scope of this analysis.
Product system and system boundary of the study
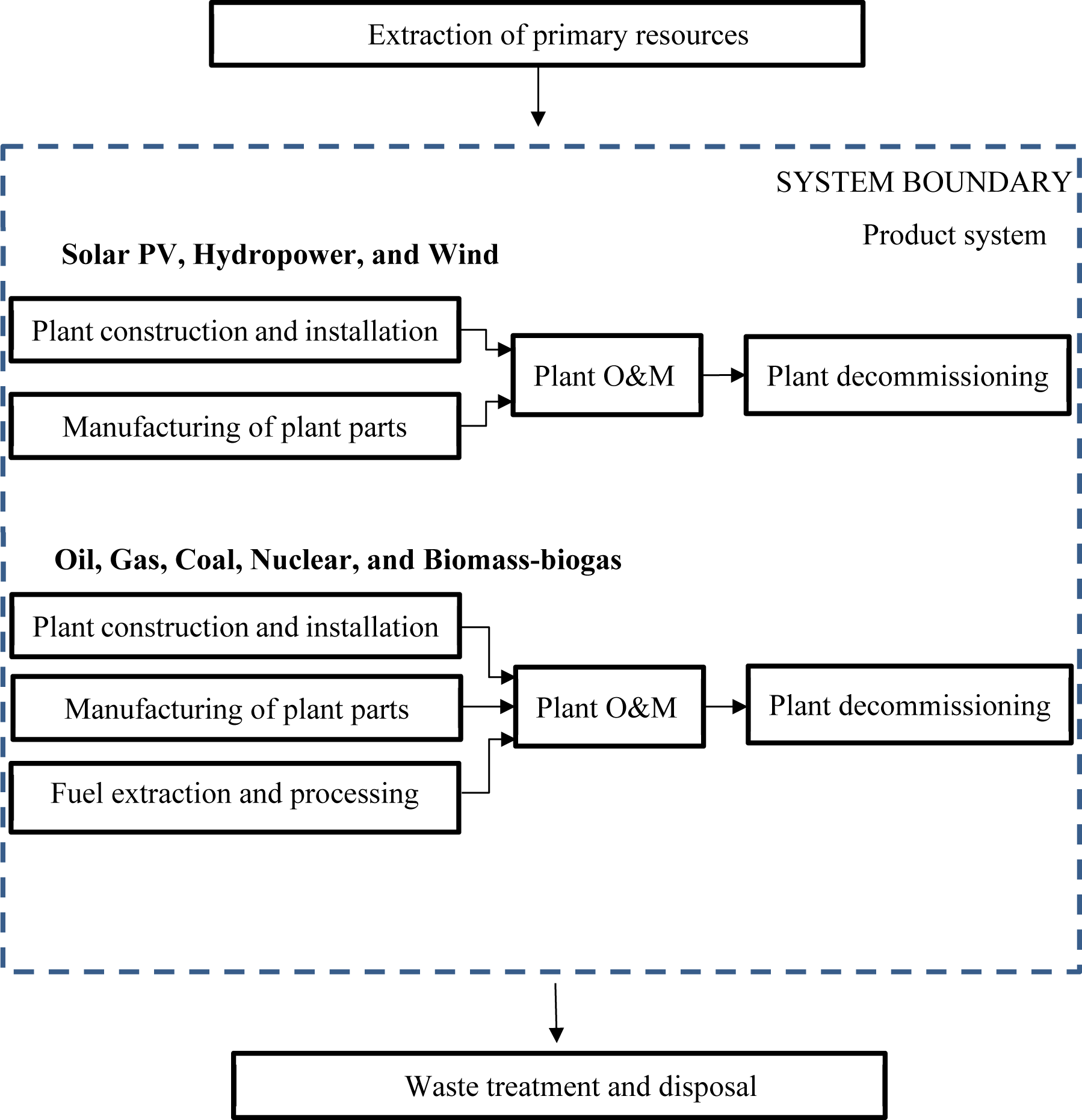
Primary and secondary data are gathered for the Life Cycle Inventory (LCI) phase. Table 2 displays the technical assumptions for each power technologies alternative. The installed capacity values presented in Table 2 are the theoretical necessary capacities, considering the efficiencies also presented in Table 2, to meet the production of 1 TWh/year (the functional unit). The data of the world installed capacity for each power technology is based on the world breakdown of the technology’s installed capacity in 2020 [33]– [35].
Summary of life cycle inventory data and assumptions
Power options | Power plant assumptions | |||
---|---|---|---|---|
Lifetime | Efficiency | Installed capacity | Breakdown of the world installed capacity | |
Solar PV | 30 years [36] | 25% [37] | 456.6 MW | China - 36.0%, USA - 10.7%, Japan - 9.5%, Germany - 7.6%, Italy - 3.1%, Australia - 2.5%, South Korea - 2.1%, Spain - 2.0%, RoW ¹ - 26.7% |
Hydro (Reservoirs) | 150 years [36][12] | 78% [36] | 146.4 MW | Brazil - 9.5%, USA - 7.3%, Canada - 7.0%, Russia - 4.4%, India - 4.0%, Norway - 2.9%, Turkey - 2.7%, Japan - 2.4%, France - 2.1%, RoW - 57.8% |
Hydro (R-o-R) | 80 years [36][12] | 82% [36] | 139.2 MW | USA - 7.3%, Canada - 7.0%, Russia - 4.4%, India - 4.0%, Turkey - 2.7%, Japan - 2.4%, France - 2.1%, RoW - 70.1% |
Onshore wind | 20 years [36]² | 20% [36] | 570.8 MW | China - 46.4%, USA - 20.0%, Germany - 9.3%, India - 6.6%, RoW - 17.8% |
Offshore wind | 20 years [36][13] | 30% [13] | 380.5 MW | UK - 30.2%, China - 26.2%, Germany - 22.5%, Netherlands - 7.3%, RoW - 13.8% |
Oil | 30 years [38] | 40%[13] | 285.4 MW | China - 28.2%, USA - 16.2%, India - 7.4%, Japan - 4.9%, Russia - 4.2%, RoW - 39.0% |
Gas | 30 years [39], [40] | 38% [36] | 300.4 MW | China - 28.2%, USA - 16.2%, India - 7.4%, Japan - 4.9%, Russia - 4.2%, RoW - 39.0% |
Coal | 30 years [36], [39] | 36.5% [39] | 312.8 MW | China - 50%, USA - 13%, India - 11%, RoW - 25% |
Nuclear | 40 years [36] | 80.4% [36] | 142.0 MW | USA - 25.0%, France - 16.1%, China - 11.6%, Japan - 8.1%, RoW - 39.2% |
Biogas | 25 years [41] | 33% [41] | 345.9 MW | Germany - 37%, USA - 11.4%, UK - 9.2%, Italy - 7.1%, Turkey - 3.7%, RoW - 31.6% |
RoW – Rest of the World;
lifetimes of the moving parts
The FWP indicator applied in this study is an adaptation of the indicator proposed by Neugebauer et al. [29]. The FWP is obtained using eq. (1). The RWn, MLWn, CWTn, and RWTn values for the construction and decommissioning (C&D), manufacture, fuel extraction, and processing are obtained from the "Fair wage characterisation" file provided by the Technischen Universität Berlin (TU Berlin) [42], and shown in Table A1 in the Appendix. Considering the countries presented in Table 2, Brazil, India, and Italy lacked data on construction, manufacturing, fuel extraction, and processing values. In these cases, additional research was carried out on specialised websites ([43]–[45]) and updated according to inflation ([44], [46]–[48]). For the IEFn values, 2020’s Gini Coefficients are considered for each country [49]. As a premise, the foreign workforce was not contemplated in the analysis, considering that globally migrant workers constituted 4.9% of the labour force of destination countries in 2019 [50].
Regarding the O&M stage, there is a lack of RWn data in TU Berlin's file. In this case, eq. (1) calculates the FWP employing the data found for each company in a country. RWn values for each analysed country considering different power technologies are calculated from spread information. Income data are from specialised websites when available [43]. Data related to “Living Wages” are from dedicated websites ([51]–[53]). Table A2 in the Appendix compiles the aforementioned factors’ values. Currency values are corrected due to inflation based on information from specific websites that estimate each country's inflation [54], [55], and values are presented in Table A3 in the Appendix.
Whenever the wage value for a given power technology is unknown, the country's workforce for this specific technology is considered. For the chosen countries, power companies that present a significant rate of their electricity generation portfolio in the form of the studied power technology are selected and analysed. Wage data is collected by means of the available reports for each company, i.e., annual/ financial/ consolidated/ or Corporate Responsibility reports. In all cases, the most recent published reports are considered. The wage reported in each document was compared with the country's minimum wage for the reference year of the report. The average values found for each company within the same country were calculated. Next, the weighted average wage among the analysed countries for the specific power technology was estimated, and this value was extrapolated to the rest of the world.
In this study, employment is the sum of direct jobs, i.e., the number of jobs during construction and installation, O&M, and decommissioning, plus indirect jobs, i.e., related to fuel extraction and processing, in the case of thermal power, as well as in the manufacture of plant parts [12]. The unit of this indicator is “jobs-year”, that is, the number of people employed for a whole year in a complete working day. The measurement procedure is based on Atilgan and Azapagic [12], Stamford and Azapagic [56], and Roinioti and Koroneos [40]. Employment for each technology is estimated, for different life cycle stages, using the Employment Factors (EFi) compiled by Rutovitz et al. [31]. Employment Factors for the selected power technologies are presented in Table 3. The factors presented in eq. (2) and Table 3 allow the calculation of the total employment:
(2)
where TE is the total employment provision over the life cycle of a given energy technology (jobs-year/TWh); Ct is the Installed Ccapacity of an energy technology (MW); EFi is the Employment Factor in the ith life-cycle stage (jobs-year/MW); di is the Duration of Employment in the ith life cycle stage (years); Ptot is the Total Amount of Energy generated over the lifetime of energy technology (TWh); J is the total number of life cycle stages; and i is the life cycle stage.
Employment in each life cycle stage at a given technology is calculated similarly to TE, although considering only the employment factor of that stage, i.e., construction and installation; manufacturing; O&M; or fuel extraction and processing. For calculating jobs created in the decommissioning stage, it is considered that it employs 20% of the number of workers in the construction stage [12]. Other premises, like efficiency, annual electricity generation, and installed capacity, are presented in Table 2.
Employment factors for different power technologies
Power technology | Construction and installation (jobs-year/MW) | Manufacturing (jobs-year/MW) | O&M (jobs/MW) | Fuel extraction and processing (jobs/PJ) |
---|---|---|---|---|
Solar PV | 13.00 | 6.70 | 0.70 | - |
Hydro (Reservoirs) | 7.40 | 3.50 | 0.20 | - |
Hydro (R-o-R) | 15.80 | 10.90 | 4.90 | - |
Onshore wind | 3.20 | 4.70 | 0.30 | - |
Offshore wind | 8.00 | 15.60 | 0.20 | - |
Oil | 1.30 | 0.93 | 0.14 | 8.60 |
Gas | 1.30 | 0.93 | 0.14 | 8.60 |
Coal | 11.20 | 5.40 | 0.14 | 40.10 |
Nuclear | 11.80 | 1.30 | 0.60 | 0.001 (jobs/GWh) |
Biogas | 14.00 | 2.90 | 1.50 | 29.90 |
By relating the FWP with the number of jobs estimated in each life cycle stage, the Employment-Weighted Fair Wage Potential (E-WFWPt) of a t technology is calculated with eq. (3):
(3)
where E-WFWPt is the Employment-Weighted Fair Wage Potential over the life cycle of a given energy technology; FWPi is the Fair Wage Potential on the life-cycle stage i; Ei is the Employment Provision in life-cycle stage i (jobs-year/TWh); TE is the Total Employment Provision over the life cycle of a given energy technology (jobs-year/TWh); J is the total number of life cycle stages; and i is the life cycle stage.
The E-WFWPi is a weighted average of the FWPn values for a life cycle stage i, considering each country's contribution to the number of jobs worldwide available for the power technology or its installed capacity. The intended results indicate the wage situation of the analysed power technologies. As in the FWPn, the E-WFWPi presents a distance-to-target impact pathway, where values smaller than 1 indicate unfair wages, while values greater than 1 suggest fair wages.
This section presents the results of each step of the assessment process.
The FWPn is calculated using eq. (1). Table 4 shows the results of FWPn for the construction, decommissioning, manufacturing, and fuel extraction and processing stages in the analysed countries. The complete data set, including RWn, MLWn, CWTn, RWTn and IEFn values, is shown in Table A1 in the Appendix.
According to the data shown in Table 4, FWP in C&D, and manufacturing stages presents the highest values in Spain (3.03 and 3.89, respectively) while China presents the lowest values (0.60 and 0.68, respectively). Regarding the fuel extraction and processing stage, Italy presents the highest FWP value for agriculture,1.78, while Germany shows the lowest, 0.79. For mining, India presents the greater value, 3.47, and China presents the lowest, 1.02.
Fair Wage Potential in different life cycle stages for the analysed countries
Country | Construction & Decommissioning | Manufacturing | Fuel extraction and processing | |
---|---|---|---|---|
Agriculture | Mining | |||
Germany | 1.64 | 2.09 | 0.79 | - |
Brazil | 0.76 | 1.46 | - | - |
China | 0.60 | 0.68 | - | 1.02 |
Spain | 3.03 | 3.89 | - | - |
USA | 2.53 | 1.93 | 1.17 | 2.21 |
France | 1.87 | 2.16 | - | 2.11 |
India | 1.71 | 2.91 | - | 3.47 |
Italy | 2.38 | 2.81 | 1.78 | - |
Japan | 1.50 | 1.46 | - | 1.44 |
UK | 2.23 | 2.33 | 1.55 | - |
Russia | 0.94 | 0.81 | - | 1.68 |
For the O&M stage, eq. (1) was used to calculate the FWP, using the data found for each company in a country. Table A2 in the Appendix shows the compilation of these values. Inflation corrections were applied as needed, and values are presented in Table A3 in the Appendix. The calculated FWPn values for each country and weighted FWPn for the O&M life cycle stage are shown in Table 5. Gas and oil technologies present the highest weighted FWPn values (3.55 and 3.51, respectively) for the O&M stage. In contrast, solar PV technology presents the lowest value (1.32), followed by biomass-biogas (1.86). At a country level, India's coal O&M shows the highest FWPn (8.14), followed by Brazil's hydropower O&M (5.69) and Japan's nuclear O&M (5.13). China’s solar PV O&M presents the lowest FWPn (0.92), followed closely by Japan's oil and gas O&M (0.97), and USA's solar PV O&M (1.52).
Fair Wage Potential of the O&M stages for the analysed technologies
Power Technology | Country | Rate of workstation/installed capacity | O&M's FWPn | O&M's weighted FWPn |
---|---|---|---|---|
Solar [57] | China | 59.0% | 0.92 | 1.32 |
Japan | 8.4% | 3.61 | ||
USA | 8.3% | 1.52 | ||
India | 7.1% | 1.71 | ||
Hydro [57] | China | 29.0% | 3.03 | 3.49 |
India | 19.0% | 2.91 | ||
Brazil | 11.0% | 5.69 | ||
Wind [35] | China | 36.4% | 2.95 | 2.65 |
USA | 16.2% | 2.42 | ||
Germany | 9.4% | 2.46 | ||
India | 5.8% | 1.94 | ||
Spain | 4.0% | 2.28 | ||
UK | 3.6% | 2.67 | ||
Oil [58] ¹ | China | 28.2% | 4.28 | 3.51 |
USA | 16.2% | 2.60 | ||
India | 7.4% | 3.48 | ||
Japan | 4.9% | 0.97 | ||
Russia | 4.2% | 4.94 | ||
Gas [58] ¹ | China | 28.2% | 4.28 | 3.55 |
USA | 16.2% | 2.74 | ||
India | 7.4% | 3.48 | ||
Japan | 4.9% | 0.97 | ||
Russia | 4.2% | 4.94 | ||
Hard coal [59] ¹ | China | 50.1% | 2.55 | 3.42 |
USA | 13.2% | 2.65 | ||
India | 11.4% | 8.14 | ||
Nuclear [58] ¹ | USA | 25.0% | 3.24 | 3.04 |
France | 16.1% | 1.71 | ||
China | 11.6% | 3.00 | ||
Japan | 8.1% | 5.13 | ||
Biomass-biogas [57] | Germany | 36.4% | 1.74 | 1.86 |
USA | 12.2% | 1.88 | ||
UK | 9.2% | 1.88 | ||
Italy | 8.1% | 2.34 |
installed capacity values used
Using the Employment Factors (EFi) for the selected power technologies presented in Table 3, the data from Table 2, the functional unit, and applying eq. (2), the employment results are depicted in Figure 2. The complete data is presented in Table A4 in the Appendix. The results suggest biomass provides the highest employment, equivalent to 1118 jobs-years/TWh. The second-best option is run-of-river with 734 jobs-years/TWh, followed by solar PV at 659 jobs-years/TWh. For this indicator, the reservoir provides the lowest life-cycle employment (41 jobs-years/TWh), possibly due to its relatively high efficiency (see Table 2) and lower labour requirements per unit of electrical output. With the results presented in Figure 2, it is possible to estimate the percentage of work positions for different electricity technologies in each life cycle stage (Table 6).
Employment provided by different electricity options.
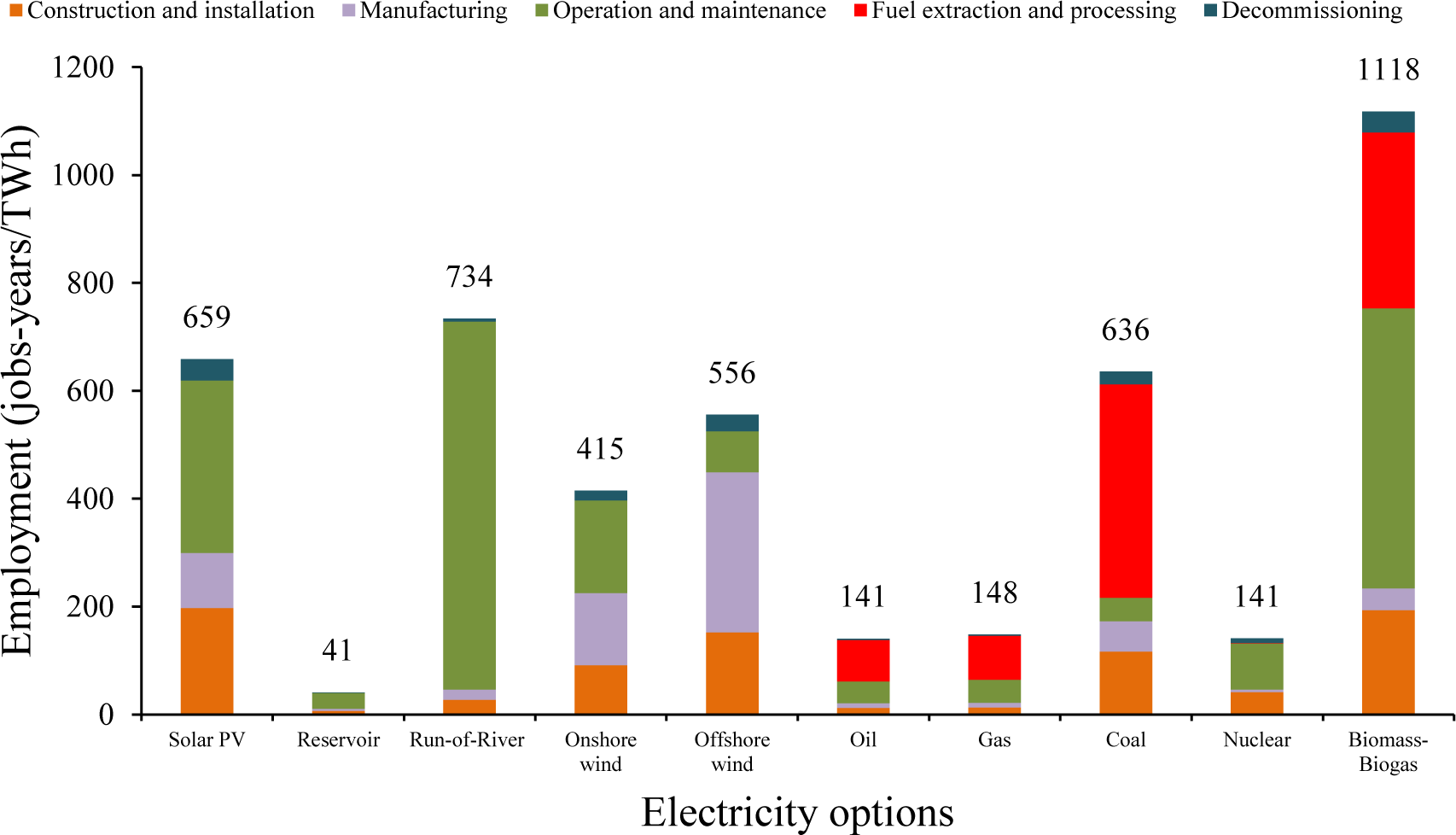
Percentage of work positions in each life cycle stage for different electricity technologies
Power technology | Percentage of work positions | ||||
---|---|---|---|---|---|
Construction & installation | Manufacturing | O&M | Fuel extraction & processing | Decommissioning | |
Solar PV | 30.0% | 15.5% | 48.5% | NA | 6.0% |
Hydro (Reservoir) | 17.5% | 8.3% | 70.8% | NA | 3.5% |
Hydro (R-o-R) | 3.7% | 2.6% | 92.9% | NA | 0.7% |
Onshore wind | 22.0% | 32.3% | 41.3% | NA | 4.4% |
Offshore wind | 27.4% | 53.4% | 13.7% | NA | 5.5% |
Oil | 8.8% | 6.3% | 28.3% | 54.9% | 1.8% |
Gas | 8.8% | 6.3% | 28.3% | 54.9% | 1.8% |
Coal | 18.4% | 8.9% | 6.9% | 62.2% | 3.7% |
Nuclear | 29.7% | 3.3% | 60.4% | 0.7% | 5.9% |
Biomass-biogas | 17.3% | 3.6% | 46.4% | 29.2% | 3.5% |
As outlined in Table 6, the O&M stage presents the highest percentage of work positions for run-of-river (92.9%), reservoir (70.8%), nuclear (60.4%), solar PV (48.5%), biomass-biogas (46.4%), and onshore wind (41.3%), showing the importance of this life cycle stage on the employment for the analysed technologies. Manufacturing emerges as a significant employment stage for offshore wind (53.4%), while the fuel extraction and processing stage presents the highest percentage of work positions for coal (62.2%), oil and gas (both with 54.9%).
The E-WFWP results for each option were estimated using Table 4, Table 5, Table 6, and eq. (3) and are displayed in Figure 3. The results indicate that run-of-river has the fairest wage potential option (3.33). Reservoir is ranked second best (2.80), followed by nuclear (2.56) and gas (2.17), the latter followed closely by oil (2.16). Solar PV technology presents the lowest E-WFWP value (1.16) but is still above the considered fair wage line. The relatively low value found for solar PV can be explained by the significant contribution of China's work positions on the weighting process (59%), with C&D, manufacturing, and O&M FWPs of 0.60, 0.68 and 0.92, respectively. Hydropower technologies also presented a low C&D FWP (0.99) due to China's C&D FWP (0.60) and Brazil's C&D FWP (0.76). However, the E-WFWPs were the highest, mainly because of the high FWP values of the O&M stage (3.49) and high work position rates: 92.9% for run-of-river and 70.8% for reservoir (see Table 6).
Employment-Weighted Fair Wage Potential of the different electricity options
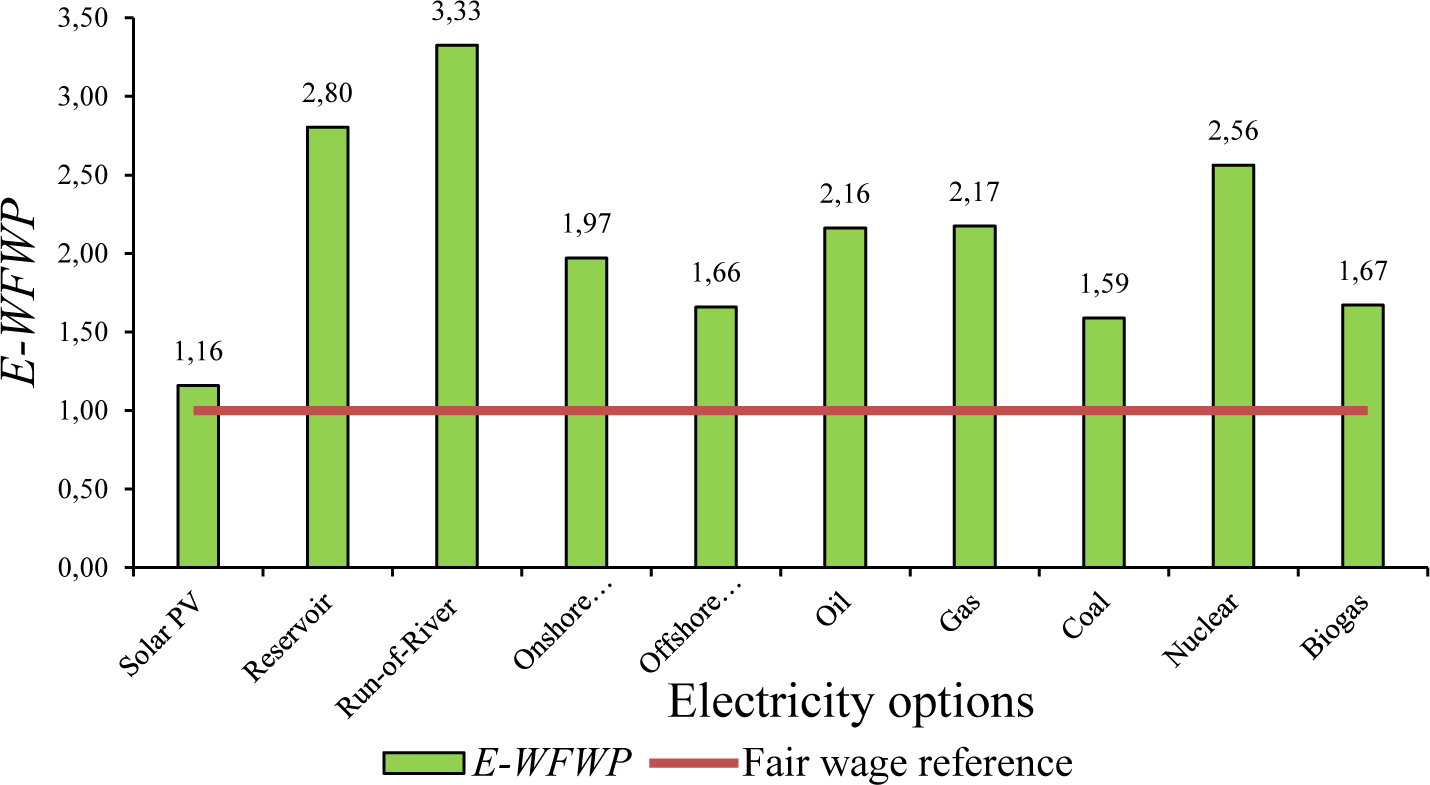
The results suggest, within the assumed premises, that the run-of-river option provides a higher social benefit concerning fair wages in the electricity generation sector. Considering the life cycle stages analysed, run-of-river could generate more positive social impacts than the other power technologies because, besides being the second most employing option (see Figure 2), the workers of the involved sectors, especially the O&M stage, have high incomes. The wage level of an individual or a family directly relates to the living situation and nutritional status, which can be linked with life expectancy, and thus to human health and social well-being [30]. In the company scope, according to the United Nations [60], beyond fulfilling a duty of care, ensuring the payment of decent wages to workers can be translated into an investment in human capital, bringing returns, such as a reduction of absenteeism and an increase of retention and motivation.
The results presented in the S-LCA, considering the impact subcategory within the stakeholder category under concern, support the selection of the electricity options with the best potential social impacts, promoting the sustainability of the power sector. Through E-WFWP, decision-makers can choose among electricity generation options considering the potential upgrades to the worker's category. The results indicate the potential use of the indicator E-WFWP as a useful tool for assessing the social benefits of power technologies besides being potentially applicable to other industries. It is worth mentioning that the reference wage of the sector/region/country significantly influences the results of a fair or unfair wage.
This paper originally proposes an employment-weighted fair wage assessment that aims to identify and implement socially sustainable electricity generation options. Recognizing the close relationship between fair salaries and SDGs 1 and 8, the study focuses on the power sector and adopts a life cycle approach to evaluate the Fair Wage Potential of ten power technologies.
At a life cycle stage level, the findings for each stage are the following:
C&D and manufacturing: Spain presents the highest FWP values (3.03 and 3.89, respectively), and China presents the lowest (0.60 and 0.68, respectively).
Fuel extraction and processing:
Agriculture: Italy has the highest FWP value for agriculture (1.78), while Germany has the lowest (0.79).
Mining: India presents the greatest value (3.47), and China the lowest (1.02).
O&M:
Gas and oil options present the highest weighted FWPn values (3.55 and 3.51, respectively), while solar PV technology presents the lowest value (1.32), followed by biomass-biogas (1.86).
At a country level, India’s coal O&M shows the highest FWPn (8.14), followed by Brazil’s hydropower O&M (5.69). China’s solar PV O&M presents the lowest FWPn (0.92), followed closely by Japan’s oil and gas O&M (0.97).
The study's outcomes on employment assessment reveal that biomass-biogas is the option with the highest employment potential, presenting 1118 jobs-years/TWh. R-o-R ranks second with 734 jobs-years/TWh, and solar PV follows closely with 659 jobs-years/TWh. On the other hand, reservoir-based power generation is identified as the least favorable option in terms of employment, with only 41 jobs-years/TWh.
Based on the results of this work, hydro options emerge as the fairest wage potential options presenting values around three times greater than the target (3.33 for R-o-R, and 2.80 for reservoir), followed by nuclear (2.56). Solar PV technology presents the lowest E-WFWP value (1.16) but is still above the fair wage line.
According to the findings of this study, it is possible to realize that the method described in this paper incorporates the social dimension into the assessment of power options' sustainability and can also be adapted to different industries and countries, which has particular significance from a community standpoint. By considering an additional social aspect when implementing new power plants, this approach can enhance power sector policies. The E-WFWP sets itself apart from existing social sustainability indicators by linking the electricity generated by power options to social data, allowing decision-makers to move beyond technical and environmental issues.
However, there are certain limitations to the method. One drawback is the challenge of obtaining sector-specific primary data such as working hours and real wages, especially if the companies being analysed do not publish annual reports. Additionally, Minimum Living Wage and Real Wage values used in the assessment need to be updated annually. Finally, it is important to note that this study did not consider unjustifiably high wages, such as managerial salaries, which warrants further discussion. Future research should expand the methodology to other stages of the power sector, such as transmission and distribution systems, as well as explore its applicability to other industries. Another issue that should be addressed is the capacity of powerful companies (ex: from oil and gas industry) to intentionally increase their employees' wages to impact the public perception as more socially sustainable than competitive low workforce power technologies.
C | Installed Capacity | [MW] |
CWT | Contracted Working Time | [hours/week] |
d | Duration of Employment | [years] |
EF | Employment Factor | [jobs-year/MW] |
E | Employment Provision | [jobs-year/TWh] |
E-WFWP | Employment-Weighted Fair Wage Potential | [FWeq] |
FWP | Fair Wage Potential | [FWeq] |
IEF | Inequality Factor | [%] |
MLW | Minimum Living Wage | [(€/month] |
P | Amount of Energy Generated | [TWh] |
RW | Real (average) Wage | [(€/month] |
RWT | Real Working Time | [hours/week] |
TE | Total Employment | [jobs-year/TWh] |
i | i-th life cycle stage |
J | total number of life cycle stages |
n | process n |
t | of an energy technology |
tot | total |
C&D | Construction & Decommissioning |
E-LCA | Environmental Life Cycle Assessment |
FU | Functional Unit |
LCA | Life Cycle Assessment |
LCI | Life Cycle Inventory |
LCSA | Life Cycle Sustainability Assessment |
O&M | Operation & Maintenance |
PV | Photovoltaic |
R-o-R | Run-Of-River |
SDG | Sustainable Development Goal |
S-LCA | Social Life Cycle Assessment |
TU | Technischen Universität |
- The Sustainable Development Agenda, 2018, https://www.un.org/sustainabledevelopment/development-agenda
A protocol for the definition of supply chains in product social life cycle assessment: application to bioelectricity ,Sustain Energy Fuels , Vol. 4 (11),pp 5533–5542 , 2020, https://doi.org/https://doi.org/10.1039/d0se00919a
, Linking energy access, gender and poverty: A review of the literature on productive uses of energy ,Energy Res Soc Sci , Vol. 53 ,pp 170–181 , 2019, https://doi.org/https://doi.org/10.1016/j.erss.2019.02.019
, A Comprehensive Analysis of the Power Demand–Supply Situation, Electricity Usage Patterns, and the Recent Development of Renewable Energy in China ,Sustainability (Switzerland) , Vol. 14 (6), 2022, https://doi.org/https://doi.org/10.3390/su14063391
, Solar Model for Rural Communities: Analysis of Impact of a Grid-Connected Photovoltaic System in the Brazilian Semi-arid Region ,Journal of Sustainable Development of Energy, Water and Environment Systems , Vol. 10 (3),pp 1–14 , 2022, https://doi.org/https://doi.org/10.13044/j.sdewes.d9.0405
, Life cycle assessment of a small hydropower plant in the Brazilian amazon ,Journal of Sustainable Development of Energy, Water and Environment Systems , Vol. 4 (4),pp 379–391 , 2016, https://doi.org/https://doi.org/10.13044/j.sdewes.2016.04.0029
, Renewable Energy Resources Technologies and Life Cycle Assessment: Review ,Energies (Basel) , Vol. 15 (24),pp 1–36 , 2022, https://doi.org/https://doi.org/10.3390/en15249417
, A review of how life cycle assessment has been used to assess the environmental impacts of hydropower energy ,Renewable and Sustainable Energy Reviews , Vol. 167 ,pp 112684 , 20212022, https://doi.org/https://doi.org/10.1016/j.rser.2022.112684
, Life cycle costing as a bottom line for the life cycle sustainability assessment in the solar energy sector: A review ,Solar Energy , Vol. 192 ,pp 238–262 , 20172019, https://doi.org/https://doi.org/10.1016/j.solener.2018.04.011
, Sustainability assessment of innovative energy technologies – Hydrogen from wind power as a fuel for mobility applications ,Journal of Sustainable Development of Energy, Water and Environment Systems , Vol. 9 (3),pp 1–21 , 2021, https://doi.org/https://doi.org/10.13044/j.sdewes.d8.0371
, Exploring the global and local social sustainability of wind energy technologies: An application of a social impact assessment framework ,Appl Energy , Vol. 312 ,pp 118808 , 2022, https://doi.org/https://doi.org/10.1016/j.apenergy.2022.118808
, An integrated life cycle sustainability assessment of electricity generation in Turkey ,Energy Policy , Vol. 93 ,pp 168–186 , 2016, https://doi.org/https://doi.org/10.1016/j.enpol.2016.02.055
, Life cycle sustainability assessment of electricity options for the UK ,Int J Energy Res , Vol. 36 (14),pp 1263–1324 , 2012, https://doi.org/https://doi.org/10.1002/er.2962
, Life cycle sustainability assessment and circularity of geothermal power plants ,Sustain Prod Consum , Vol. 35 ,pp 141–156 , 2023, https://doi.org/https://doi.org/10.1016/j.spc.2022.10.027
, Uncovering the socioeconomic impacts of China’s power system decarbonization ,Environ Impact Assess Rev , Vol. 99 ,pp 107015 , 20222023, https://doi.org/https://doi.org/10.1016/j.eiar.2022.107015
, Social Life Cycle Assessment of a Concentrated Solar Power Plant in Spain: A Methodological Proposal ,J Ind Ecol , Vol. 21 (6),pp 1566–1577 , 2017, https://doi.org/https://doi.org/10.1111/jiec.12541
, - ISO 14040:2006(E) - Environmental management — Life cycle assessment — Principles and framework, 2006, http://www.springerlink.com/index/10.1007/s11367-011-0297-3
- Guidelines for social life cycle assessment of products and organizations 2020, 2020, https://www.lifecycleinitiative.org/wp-content/uploads/2020/12/Guidelines-for-Social-Life-Cycle-Assessment-of-Products-and-Organizations-2020-sml.pdf
Development of social sustainability assessment method and a comparative case study on assessing recycled construction materials ,Int J Life Cycle Assess , Vol. 23 (8),pp 1654–1674 , 2018, https://doi.org/https://doi.org/10.1007/s11367-017-1373-0
, - ISO 26000:2010 (E) - Guidance on social responsibility, 2010, https://documentation.lastradainternational.org/lsidocs/3078-ISO 26000_2010.pdf
The guidelines for social life cycle assessment of products: Just in time! ,International Journal of Life Cycle Assessment , Vol. 15 (2),pp 156–163 , 2010, https://doi.org/https://doi.org/10.1007/s11367-009-0147-8
, - Guidelines for Social Life Cycle Assessment of Products, 2009, https://wedocs.unep.org/20.500.11822/7912
Introduction to evaluating energy justice across the life cycle: A social life cycle assessment approach ,Appl Energy , Vol. 236 ,pp 211–219 , 20182019, https://doi.org/https://doi.org/10.1016/j.apenergy.2018.11.022
, Towards life cycle sustainability assessment: An implementation to photovoltaic modules ,International Journal of Life Cycle Assessment , Vol. 17 (8),pp 1068–1079 , 2012, https://doi.org/https://doi.org/10.1007/s11367-012-0433-8
, Sustainability assessment of electricity cogeneration from sugarcane bagasse in Jamaica ,J Clean Prod , Vol. 200 ,pp 390–401 , 20182018, https://doi.org/https://doi.org/10.1016/j.jclepro.2018.07.322
, Environmental and social life cycle assessment to enhance sustainability of sugarcane-based products in Thailand ,Clean Technol Environ Policy , Vol. 21 (7),pp 1447–1458 , 2019, https://doi.org/https://doi.org/10.1007/s10098-019-01715-y
, Living wages in Portugal: In search of dignity in a polarised labour market ,Soc Policy Adm ,pp 1–16 , 20222023, https://doi.org/https://doi.org/10.1111/spol.12887
, Impact pathways to address social well-being and social justice in SLCA-Fair wage and level of education ,Sustainability (Switzerland) , Vol. 6 (8),pp 4839–4857 , 2014, https://doi.org/https://doi.org/10.3390/su6084839
, Calculation of Fair wage potentials along products’ life cycle – Introduction of a new midpoint impact category for social life cycle assessment ,J Clean Prod , Vol. 143 ,pp 1221–1232 , 2017, https://doi.org/https://doi.org/10.1016/j.jclepro.2016.11.172
, Fair wage potential as a tool for social assessment in building projects ,Engineering, Construction and Architectural Management , Vol. 28 (4),pp 1295–1318 , 2020, https://doi.org/https://doi.org/10.1108/ECAM-01-2020-0024
, - Calculating global energy sector jobs: 2015 methodology - Prepared for Greenpeace International by the Institute for Sustainable Futures, University of Technology, 2015, https://opus.lib.uts.edu.au/bitstream/10453/43718/1/Rutovitzetal2015Calculatingglobalenergysectorjobsmethodology.pdf
- , ISO 14044:2006 (en) Environmental management — Life cycle assessment — Requirements and guidelines, 2006
- Data and statistics, 2020, https://www.iea.org/data-and-statistics
- Country Rankings, 2020, https://www.irena.org/Statistics/View-Data-by-Topic/Capacity-and-Generation/Country-Rankings
- Global Wind Installations, 2021, https://library.wwindea.org/global-statistics
- , Life Cycle Inventories of Energy Systems: Results for Current Systems in Switzerland and other UCTE Countries. Ecoinvent report No. 5. Paul Scherrer Institut Villigen, Swiss Centre for Life Cycle Inventories, 2007
- Photovoltaic Projects in Energy Auctions - Analysis of the 2019 A-4 and A-6 auctions (in Portuguese), 2020, https://www.epe.gov.br/sites-pt/publicacoes-dados-abertos/publicacoes/PublicacoesArquivos/publicacao-457/NT EPE-DEE-003-2020-r0.pdf
Life cycle sustainability assessment of electricity generation in Pakistan: Policy regime for a sustainable energy mix ,Energy Policy , Vol. 111 ,pp 111–126 , 2017, https://doi.org/https://doi.org/10.1016/j.enpol.2017.09.022
, Life-cycle sustainability assessment of key electricity generation systems in Portugal ,Energy , Vol. 176 ,pp 131–142 , 2019, https://doi.org/https://doi.org/10.1016/j.energy.2019.03.166
, Integrated life cycle sustainability assessment of the Greek interconnected electricity system ,Sustainable Energy Technologies and Assessments , Vol. 32 ,pp 29–46 , 20182019, https://doi.org/https://doi.org/10.1016/j.seta.2019.01.003
, - Life cycle inventories of bioenergy. ecoinvent report No. 17, Swiss Centre for Life Cycle Inventories, 2007, https://db.ecoinvent.org/reports/17_bioenergy.pdf
- Fair wage equivalents, 2016, https://www.tu.berlin/see/forschung/datensaetze-methoden-und-tools/fair-wage-aequivalente
- PayScale - Salary Comparison, Salary Survey, Search Wages, 2021, http://www.payscale.com
- RAIS - 2021, 2021, http://pdet.mte.gov.br/index.php/rais
- 2012 Construction Sectoral Study (in Portuguese), 2013, http://www.dieese.org.br/estudosetorial/2012/estPesq65setorialConstrucaoCivil2012.pdf
- Citizen’s calculator (in Portuguese), 2021, https://www3.bcb.gov.br/CALCIDADAO/publico/corrigirPorIndice.do?method=corrigirPorIndice
- Inflation Calculator, 2021, https://www.inflationtool.com
- EUR/INR - Euro Indian Rupee, 2021, https://br.investing.com/currencies/eur-inr-historical-data
- Ranking of the Gini index by country 2020, 2021, https://www.statista.com/forecasts/1171540/gini-index-by-country
- ILO Global Estimates on International Migrant Workers, 2021, https://www.ilo.org/wcmsp5/groups/public/---dgreports/---dcomm/---publ/documents/publication/wcms_808935.pdf
- Minimum Wages, Living Wages and Labour Law Around the World, 2021, https://wageindicator.org
- The Calculation: The living wage - based on the real cost of living, 2021, http://www.livingwage.org.uk/calculation
- Report on Hong Kong Living Wage Research, 2018, https://www.oxfam.org.hk/en/f/news_and_publication/16369/Oxfam_Living Wage_eNG_20181228.pdf
- Empowering people with data, 2021, https://www.statista.com
- Macrotrends - The Premier Research Platform for Long Term Investors, 2021, https://www.macrotrends.net
Sustainability indicators for the assessment of nuclear power ,Energy , Vol. 36 (10),pp 6037–6057 , 2011, https://doi.org/https://doi.org/10.1016/j.energy.2011.08.011
, - Renewable Energy and Jobs – Annual Review 2020, 2020, https://www.irena.org/publications/2020/Sep/Renewable-Energy-and-Jobs-Annual-Review-2020
World Energy Statistics 2019 ,World Energy Statistics , 2019, https://doi.org/https://doi.org/10.1787/2e828dea-en
, - Mapped: The world’s coal power plants in 2020, 2020, https://www.carbonbrief.org/mapped-worlds-coal-power-plants
- Improving Wages to Advance Decent Work in Supply Chains, 2023, https://livingwages.unglobalcompact.org/#undefined
- 2019 ANNUAL REPORT, 2016, https://www.xinyisolar.com/uploadfiles/2020/04/20200414181308551.pdf
- 2019 Annual Report (in Chinese), 2020, https://pdf.dfcfw.com/pdf/H2_AN202003291377152197_1.pdf
- 2019 Annual Report (in chinese), 2019, https://en.longigroup.com/uploadfile/3/2020/0617/20200617082853768.pdf
- Annual Report 2019, 2020, http://media-gclpoly.todayir.com/2020042917400380139259604_en.pdf
- 2019 Annual Report (in Chinese), 2020, https://pdf.dfcfw.com/pdf/H2_AN202004171378199829_1.pdf
- Annual Report 2020, https://group.softbank/system/files/pdf/ir/financials/annual_reports/annual-report_fy2020_01_en.pdf
- FORM 20-F, 2021, https://www.sec.gov/Archives/edgar/data/1070304/000095012320006565/ix-20200331.htm
- 2020 Sustainability Report, 2020, https://www.mitsui.com/jp/en/sustainability/sustainabilityreport/2020/index.html
- Annual Report, 2020, https://global.kyocera.com/ir/library/pdf/FY20_4Q_ar.pdf
- Clean Jobs, Better Jobs: An examination of clean energy job wages and benefits, 2020, https://e2.org/wp-content/uploads/2020/10/Clean-Jobs-Better-Jobs.-October-2020.-E2-ACORE-CELI.pdf
- Solar Photovoltaic (PV) Power Generation Salary in India _ PayScale, 2021, https://www.payscale.com/research/IN/Industry=Solar_Photovoltaic_(PV)_Power_Generation/Salary
- Renewable Energy Power Generation Salary in China _ PayScale, 2021, https://www.payscale.com/research/CN/Industry=Renewable_Energy_Power_Generation/Salary
- 2019 Annual Report (in Chinese), 2019, http://m.zdydep.com/skin/default/file/远达环保2019年年度报告.pdf
- 2018 Annual Report (in Chinese), 2019, https://www.sdicpower.com/gtdl/tzzgx/dqbg/webinfo/2020/07/1595186412816566.htm
- Hydroelectric Power Generation Salary in India _ PayScale, 2021, https://www.payscale.com/research/IN/Industry=Hydroelectric_Power_Generation/Salary
- 2019 Annual Report (in Portuguese), 2020, https://eletrobras.com/pt/Paginas/Relatorio-Anual.aspx
- 2019 Annual and Socioenvironmental Report (in Portuguese), 2019, https://www.norteenergiasa.com.br/assets/norteenergia-pt-br/media/documents/attachments/source/20210311103154686-20201117164719915-Relat%C3%B3rio%20Anual%20e%20Socioambiental%20%202019.pdf
- 2019 Annual Report Itaipu Binacional (in Portuguese), 2019, https://www.itaipu.gov.br/sites/default/files/af_df/RELATORIO_ANUAL_ITAIPU_2019.pdf
- Release 4T19 and 2019, 2020, https://ri.aesbrasil.com.br/listresultados.aspx?idCanal=odsi0VDA//tdq2Cw4tP9Zw==
- 2019 Annual Report (in chinese), 2020, http://qccdata.qichacha.com/ReportData/PDF/405bc48fa0c78857641569f779380ba4.pdf
- 2019 Annual Report, 2020, https://www1.hkexnews.hk/listedco/listconews/sehk/2020/0428/2020042800780.pdf
- 2019 Annual Report (in chinese), 2020, https://pdf.dfcfw.com/pdf/H2_AN202004301379078639_1.pdf
- Wind Power Generation Salary _ PayScale (US), 2021, https://www.payscale.com/research/US/Industry=Wind_Power_Generation/Salary
- Wind Power Generation Salary in Germany _ PayScale, 2021, https://www.payscale.com/research/DE/Industry=Wind_Power_Generation/Salary
- Wind Power Generation Wind Energy Generation in India, 2021, https://www.payscale.com/research/IN/Industry=Wind_Power_Generation/Salary
- Wind Power Generation Salary in Spain _ PayScale, 2021, https://www.payscale.com/research/ES/Industry=Wind_Power_Generation/Salary
- Wind Power Generation Salary in United Kingdom _ PayScale, 2021, https://www.payscale.com/research/UK/Industry=Wind_Power_Generation/Salary
- Oil and Gas Exploration Salary in China | PayScale, 2021, https://www.payscale.com/research/CN/Industry=Oil_and_Gas_Exploration/Salary
- Electric Power Distribution Salary in China _ PayScale, 2021, https://www.payscale.com/research/CN/Industry=Electric_Power_Distribution/Salary
- FOSSIL FUEL POWER GENERATION US, 2021, https://www.payscale.com/research/US/Industry=Fossil_Fuel_Power_Generation/Salary
- Fossil Fuel Power Generation Salary in India _ PayScale, 2021, https://www.payscale.com/research/IN/Industry=Fossil_Fuel_Power_Generation/Salary
- TEPCO Integrated Report 2020 Financial Section, 2020, https://www.tepco.co.jp/en/hd/about/ir/library/integratedreport/pdf/FS20.pdf
- 2019 Annual Report, 2020, https://www.unipro.energy/en/investors/information_disclosure/annual_reports
- PJSC Gazprom Annual Report 2019, 2019, https://www.gazprom.com/f/posts/72/802627/gazprom-annual-report-2019-en.pdf
- Annual report 2019 - Sustainable Development and Environmental Responsibility Report, 2020, https://www.interrao.ru/upload/InterRAO_AR19_ENG.pdf
- 2019 Annual Report, 2020, https://www1.hkexnews.hk/listedco/listconews/sehk/2020/0427/2020042700611.pdf
- Annual Report 2018, 2019, http://en.hdpi.com.cn/webfront/common/info/doFileOnline/serviceId/articleAttach_20190417165807
- 2019 Annual Report, 2020, https://www1.hkexnews.hk/listedco/listconews/sehk/2020/0329/2020032900663.pdf
- 43rd Annual Report 2018-19, 2019, https://www.ntpc.co.in/annual-reports/8842/download-complete-annual-report-2018-19
- Annual Report 2019-20, 2021, https://reports.adani.com/adanipower/annual_reports_20/index.html
- Future ready for smart choices - INTEGRATED ANNUAL REPORT 2019-20, 2020, https://www.tatapower.com/investor-relations/tata-power/pdf/Tata_Power_IR_201920.pdf
- Annual Report (2019-20), 2019, https://www.reliancepower.co.in/documents/2181716/2359750/Abridged_AR_2018-19.pdf
- 64th Annual Report 2019-20, 2020, https://www.nlcindia.com/investor/AnnualReport2020.pdf
- Nuclear Power Generation US, 2021, https://www.payscale.com/research/US/Industry=Nuclear_Power_Generation/Hourly_Rate
- Nuclear Power Generation FR, 2021, https://www.payscale.com/research/FR/Industry=Nuclear_Power_Generation/Salary
- 2020 Annual report, 2021, https://www.clpgroup.com/content/dam/clp-group/channels/investor/document/3-3-financial-reports/2020/e_2020Annual Report.pdf.coredownload.pdf
- 2019 Annual Report, 2020, https://pdf.dfcfw.com/pdf/H2_AN202004231378507869_1.pdf
- 2019 Annual Report, 2020, https://www1.hkexnews.hk/listedco/listconews/sehk/2020/0402/2020040201283.pdf
- 2019 CSR & Financial Report, 2019, https://www.kepco.co.jp/english/corporate/list/report/pdf/e2019.pdf
- Annual Report 2019, 2020, https://www.envitec-biogas.com/fileadmin/media/pdf_downloads/subpage_company/InvestorRelations/EN/Financial_reports/2019_Annual_Report_EN_web.pdf
- Animal Waste Biomethane Gas Collection Salary _ PayScale US, 2021, https://www.payscale.com/research/US/Industry=Animal_Waste_Biomethane_Gas_Collection/Salary
- Biofuel Power Generation Salary _ PayScale US, 2021, https://www.payscale.com/research/US/Industry=Biofuel_Power_Generation/Salary
- Biofuel Power Generation Salary in United Kingdom _ PayScale, 2021, https://www.payscale.com/research/UK/Industry=Biofuel_Power_Generation/Salary
- Renewable Energy Power Generation Salary in Italy _ PayScale, 2021, https://www.payscale.com/research/IT/Industry=Renewable_Energy_Power_Generation/Salary
- Hong Kong Inflation Rate 1982-2021, 2021, https://www.macrotrends.net/countries/HKG/hong-kong/inflation-rate-cpi