The environmental legislation establishes the conditions and standards for the discharge of effluents and, consequently, defines the objectives of wastewater treatment plants (WWTPs). Thus, WWTPs are designed, operated, and maintained in light of environmental legislation, which mainly determines the removal of certain pollutants [1].
In Brazil, federal environmental legislation basically requires the removal of carbonaceous organic matter [2], [3]. However, local environmental laws, such as those of the federation states, may be stricter and require the removal of nitrogen compounds, phosphorus, and pathogenic organisms [4]. The determination to remove such pollutants is imperative because when not removed, they cause several impacts, such as oxygen depletion and eutrophication of water bodies, waterborne diseases, and toxicity to fish and plants, among others [5].
Although several technological routes for wastewater treatment have the same treatment objectives, each route has its particularities, holding advantages and disadvantages concerning implementation, operation and maintenance costs, electricity consumption, sludge management, operational requirements, space requirements, reliability, etc. [6], [7]. In general, the decision-making processes to choose the most suitable system for the treatment of wastewater are mainly influenced by conventional techno-economic aspects of engineering, that is, by the costs of implementation, operation, and maintenance and by the space requirements [8], as long as the system meets the treatment objectives defined by local environmental standards [9].
However, a broader approach to sustainability needs to be considered, including environmental and social aspects, in the decision-making process of the route to be designed, operated, and maintained since, despite the benefits generated by the WWTPs, they also generate negative impacts during their lifespans, from construction to decommissioning. Among these impacts, the following can be highlighted: climate change, water depletion, eutrophication, depletion of mineral resources, odour emission, noise level, capacity to generate employment, and biological risk [10], [11].
The importance of the subject of this study is also reflected by the United Nations' (UN) 6th Sustainable Development Goal, whose ultimate purpose is to ensure the availability and sustainable management of water and sanitation for all by 2030 [12]. The expectation is that the number of sewage treatment plants will grow considerably in the coming years in the country since (i) the treatment rate of wastewater generated in Brazil in 2021 was only 51.2% [13]; (ii) the Brazilian federal law No. 14026/2020, which establishes national guidelines for basic sanitation, determines that 90% of the population must be served with wastewater collection and treatment by 2033 [14]; and, (iii) the fulfilment of the 6th Sustainable Development Goal mentioned above, to which Brazil is a signatory, must be carried out by 2030 [12].
However, meeting these goals has to occur gradually and progressively, including expanding the objectives of wastewater treatment, which should first focus on removing carbonaceous organic matter, nutrients, and other contaminants. This concern was externalised and analysed by Chernicharo et al. [15], who sought to understand the scenario of the main treatment technologies used in Brazil by assessing 1,667 wastewater treatment plants present in six Brazilian states. The authors concluded that the most applied compact wastewater treatment technologies are up-flow anaerobic sludge blanket (UASB) reactors and activated sludge.
UASB reactors, as single units or followed by some form of post-treatment, are the main current trend, which places Brazil as the holder of the largest park of anaerobic reactors in the world, considering the technology application for wastewater treatment [16]. One of the challenges of wastewater treatment expansion in Brazil lies in the relative diversity of options for technological routes that aim to remove carbonaceous organic matter exclusively.
Life cycle assessment (LCA) and life cycle costing (LCC) can comprehensively assess wastewater systems, promoting the development of system sustainability by identifying their critical processes and potential areas for improvement [17]. LCC can be processed besides LCA calculation by using commercial software. However, according to Estevan and Schaefer [18], LCC can also be calculated based on the main components of capital expenditure (CAPEX) and operational expenditure (OPEX). Although these tools are standardised by the International Organization for Standardization (ISO): 14040:2006 [19], 14044:2006 [20], and 15686-5:2017 [21], and have great value in evaluating the environmental and economic performance of WWTPs [22], [23], the LCA and LCC are rarely considered in decisions about wastewater projects in developing countries [9].
In Brazil, there is a lack of studies that contemplate LCA and wastewater treatment [24]. Therefore, it is inferred that the joint use of LCA and LCC to evaluate the performance of wastewater treatment is even smaller [23]. In this context, there is a need to evaluate the environmental and economic performance of wastewater treatment plants commonly used in Brazil, both due to the lack of studies of this nature and the advanced treatment of wastewater due to the new Brazilian law on basic sanitation, with an increase in new WWTPs, which are mainly chosen for their simplicity, self-sustainability, and costs [6].
So, this article proposes to comparatively assess, through LCA and LCC, the environmental and economic impacts of different wastewater treatment technological routes commonly used in Brazil, applied to different nominal capacities, but which aim to remove carbonaceous organic matter exclusively.
The material and methods were divided into four steps, as detailed in the following sections.
The studied wastewater treatment technologies are described in Table 1, which were selected considering the particularities of sanitation provision in Brazil and its technological expansion challenges.
Technological routes and their units dimensioned and evaluated comparatively
Technological Routes | Liquid Phase | Solid Phase | Gaseous Phase |
---|---|---|---|
Activated sludge (AS) | Coarse screen |
Gravity thickening |
Enclosed gas flare |
UASB reactor + Activated sludge (UASB + AS) | Coarse screen |
Centrifuge |
Enclosed gas flare |
Trickling filter (TF) | Coarse screen |
Gravity thickening |
Enclosed gas flare |
UASB reactor + Trickling filter (UASB + TF) | Coarse screen |
Centrifuge |
Enclosed gas flare |
The selected wastewater treatment plants were dimensioned under the recommendations of the Brazilian standard on hydraulic and process projects for WWTPs [25]. In addition, the technical guidelines described in the following references were adhered to [5]–[7], [26]–[28]. Furthermore, the reliability concept was considered as the probability of adequate performance during a specified period under specified conditions. In other words, regarding the performance of a WWTP, it would be the percentage of time that the effluent concentrations meet the emission requirements [26].
The methodology used was developed by Niku et al. [29]. For all the studied WWTPs, a level of reliability frequently used (which should be greater than 80%) and a coefficient of variation often found in practice (which should be lower than 1) were adopted, namely 95% and 0.80, respectively, resulting in a coefficient of reliability of 0.40 [30]. Other adopted parameters were: per capita water consumption of 200 L⸱inhab⁻¹⸱d⁻¹ [31]; per capita biochemical oxygen demand of 48 g⸱inhab⁻¹⸱d⁻¹; per capita chemical oxygen demand of 96 g⸱inhab⁻¹⸱d⁻¹; per capita total suspended solids of 56 g⸱inhab⁻¹⸱d⁻¹ [25]; wastewater flow/water flow return coefficient of 80%; peak coefficient for the day with the highest water consumption of 1.20; and, peak coefficient for the hour with the highest water consumption of 1.50 [32].
The wastewater treatment technological routes were designed to remove carbonaceous organic matter exclusively; therefore, they had to comply with Brazilian environmental legislation in this regard: (i) biochemical oxygen demand (BOD) of 60 mg⸱L-1 for treated wastewater [33] and (ii) dilution capacity of receptor bodies as a function of framing classes [2], [3].
Thus, considering the concept of reliability and the above parameters adopted, the wastewater treatment plants were designed so that their treated wastewater had a BOD of 24 mg⸱L-1, which would allow the WWTPs to serve more than half of the Brazilian municipalities since they have receptor bodies with the capacity to dilute the treated wastewater in these characteristics, managing to maintain their framing classes [34].
Finally, it should be noted that the wastewater treatment plants were dimensioned to meet two different population sizes: 10,000 inhabitants and 100,000 inhabitants. Thus, it was intended to verify whether the variation in the magnitude of the environmental and economic impacts of the WWTPs is proportional to the variation in their respective treatment capacities. In addition, the article covers the population of most Brazilian cities [35] once WWTPs can be implemented through modules, usually around 4 or 5 modules.
The dimensioning was realised using electronic spreadsheets available in the supplementary material.
This step was prepared based on ISO standards 14040:2006 [19], 14044:2006 [20], and the good practices described by Corominas et al. [22].
The wastewater treatment plants were designed to remove carbonaceous organic matter exclusively, quantified in terms of biochemical oxygen demand. Therefore, the functional unit adopted was the removal of BOD load. The lifespan adopted for each WWTP was 20 years, a value following Brazilian National Health Foundation (Funasa) guidelines, which determine that municipal sanitation plans must be prepared with a 20-year horizon [36].
In this context, considering the design parameters described above, the WWTPs designed to serve 10,000 inhabitants have an average flow of 18.52 L⸱s-1 and an average BOD removal efficiency of 92%, so in 20 years, they should treat 11,680,934.40 m³ of wastewater and remove 3,223.68 tons of carbonaceous organic matter. Regarding the WWTPs designed to serve 100,000 inhabitants, an average flow of 185.19 L⸱s-1 and an average BOD removal efficiency of 92% were adopted. Thus, in 20 years, they should treat 116,803,036.80 m³ of wastewater and remove 32,236.80 tons of carbonaceous organic matter.
The system boundary was limited to each technological route's liquid and solid phases. In contrast, the gaseous phase was disregarded because it is good practice not to estimate the emissions from enclosed flaring, as most carbon dioxide (CO2) emissions are of biogenic origin [27]. The methane (CH4) and nitrous oxide (N2O) emissions are negligible because they are very small [28].
Moreover, the discharge of wastewater treated into the surface water body was also disregarded since the LCAs aim to comparatively evaluate the WWTPs, which have treated wastewater with the same characteristics. Consequently, it can exclude equal parts of the life cycle. Thus, the steps covered were construction and operation, excluding maintenance and decommissioning, which also can be disregarded for the study systems since maintenance activities do not require significant inputs, and decommissioning impacts are negligible over the typical operating lifetime of WWTPs [22].
The preparation of life cycle inventories of the technological routes for wastewater treatment was carried out based on the results of the design and sizing, which made it possible to quantify the inputs and outputs that occurred during the construction and operation stages, available in the supplementary material. During the construction stage, civil works and equipment were considered. Civil works were estimated from the total volumes of concrete calculated through the dimensions of the slabs and walls of the units that compose the studied WWTPs. A concrete thickness of 0.25 m was adopted for all units. The other materials and services, based on Navàs WWTP inventory [37], were estimated based on multiplication factors for the total concrete volumes. This methodology is recommended by Corominas et al. [22] and Morera et al. [37] when one can find construction information.
The equipment was specified based on the units that compose the studied WWTPs, considering only the large pieces, whose adoption depends on the technological route: manual coarse screen, mechanical coarse screen, fine screen, mechanical grit chamber, Parshall flume, sludge scraper, blower, packing medium in plastic rings, rotating distribution system, sludge mixing device, enclosed gas flare, and centrifuge. They were included in the inventories by accounting for the masses of their primary materials [22], [37]. This information was obtained from Brazilian suppliers.
In the operation stage, the energy consumption by electromechanical equipment was estimated based on its potencies obtained from Brazilian suppliers. Another aspect considered was the transport and consumption of polyelectrolyte by centrifuges. Finally, the transport and disposal of dewatered sludge in the sanitary landfill were also considered. It is important to mention that the distance considered for both transports was 40 km, coinciding with the distance adopted in the Navàs WWTP inventory [37].
For the background system, the inputs and outputs from the inventories were linked to global or Brazilian processes in the Ecoinvent database 3.8 Cut-off Unit Regionalized.
The life cycle impact assessment (LCIA) method used was ReCiPe 2016, considering the following impact categories of the Midpoint approach for a 100-year horizon (Hierarchist): Fine Particulate Matter Formation; Fossil Resource Scarcity; Global Warming; Human Carcinogenic Toxicity; Land Use; Ozone Formation – Terrestrial Ecosystem; Terrestrial Acidification; and Water Consumption. These impact categories were chosen because they were identified as relevant after screening the Endpoint Indicators; that is, they contributed at least 5% in some area of protection [22]. It is important to note that although the gaseous phase was not considered, the impact categories of Global Warming and Ozone Formation – Terrestrial Ecosystem are still representative because of processes related to energy consumption, civil works, and others, as detailed in the next section. The life cycle assessments were applied using the software openLCA, version 1.11.0.
Differently from the application of openLCA software for LCA calculations, LCC was calculated through an Excel spreadsheet based on the main components of CAPEX and OPEX and according to good practices described by Estevan and Schaefer [18] and Ilyas et al. [23]. It is important to say that the cost of land was not considered because a WWTP is a public infrastructure, and the price of the area strongly depends on the location.
As in the LCA, the costs of technological routes were divided into construction and operation costs. The construction costs considered were those related to civil works and equipment acquisition.
The costs of civil works were estimated by multiplying the total volumes of concrete from the WWTPs by the unit cost of the execution of reinforced concrete structures for a single-story institutional building (3,402.09 R$⸱m-3), which is a synthetic composition elaborated by the Brazilian National System of Costs Survey and Indexes of Construction (SINAPI) for the state of Minas Gerais, contemplating, in short: the framing of reinforced concrete structures; manufacture, assembly and dismantling of formworks; and, launching, densifying and finishing of concrete [38].
The equipment considered was the same as described in the Inventory analysis, and its acquisition costs were obtained from Brazilian suppliers. The proposals did not consider the costs of transporting the equipment because they are unique products which cannot be easily found in any city. So, such costs depend on the locations of the WWTPs and suppliers.
Only the above costs were considered as the construction costs of the technological routes because they are the most important in constructing wastewater treatment plants.
The operating costs included energy consumption by electromechanical equipment, transport and consumption of polyelectrolyte by centrifuges, and transport and disposal of dewatered sludge in a sanitary landfill. The energy consumption costs by electromechanical equipment were estimated based on their potencies, time of use, and average electricity tariff of 0.619 R$⸱kWh−1 for the state of Minas Gerais [39]. The acquisition costs of polyelectrolyte (26.04 R$⸱kg−1) and disposal of dewatered sludge in a sanitary landfill (140.00 R$⸱t−1) were obtained from Brazilian companies.
Finally, the costs due to transportation were estimated based on the unit cost of SINAPI synthetic composition for transport (1.15 R$⸱t−1⸱km−1) for the state of Minas Gerais [38]. As in the LCA, it is highlighted that the distance considered for the transports described above was 40 km, coinciding with the distance adopted in the Navàs WWTP inventory [37].
It should also be noted that the above costs refer to the second half of 2021. However, the operating costs of each WWTP were estimated over its entire lifespan, that is, 20 years. For this, the Present Value method was used to bring future costs to present costs [6], considering as an annual nominal inflation rate the arithmetic average of the last 20 years of the General Price Index – Market (IGP–M): 8.6850%, which takes into account variations in the prices of goods and services, as well as those of raw materials used in agriculture, manufacturing, and construction. In Brazil, it is widely used in the parametric formula for readjusting public tariffs (such as energy), rental contracts, and service provision contracts [40].
Figure 1 quantifies the impact category of Fine Particulate Matter Formation, which is primarily driven by electricity consumption. Electricity consumption remains the primary factor contributing to Fossil Resource Scarcity, as shown in Figure 2.
Contribution of the components of WWTPs to Fine Particulate Matter Formation
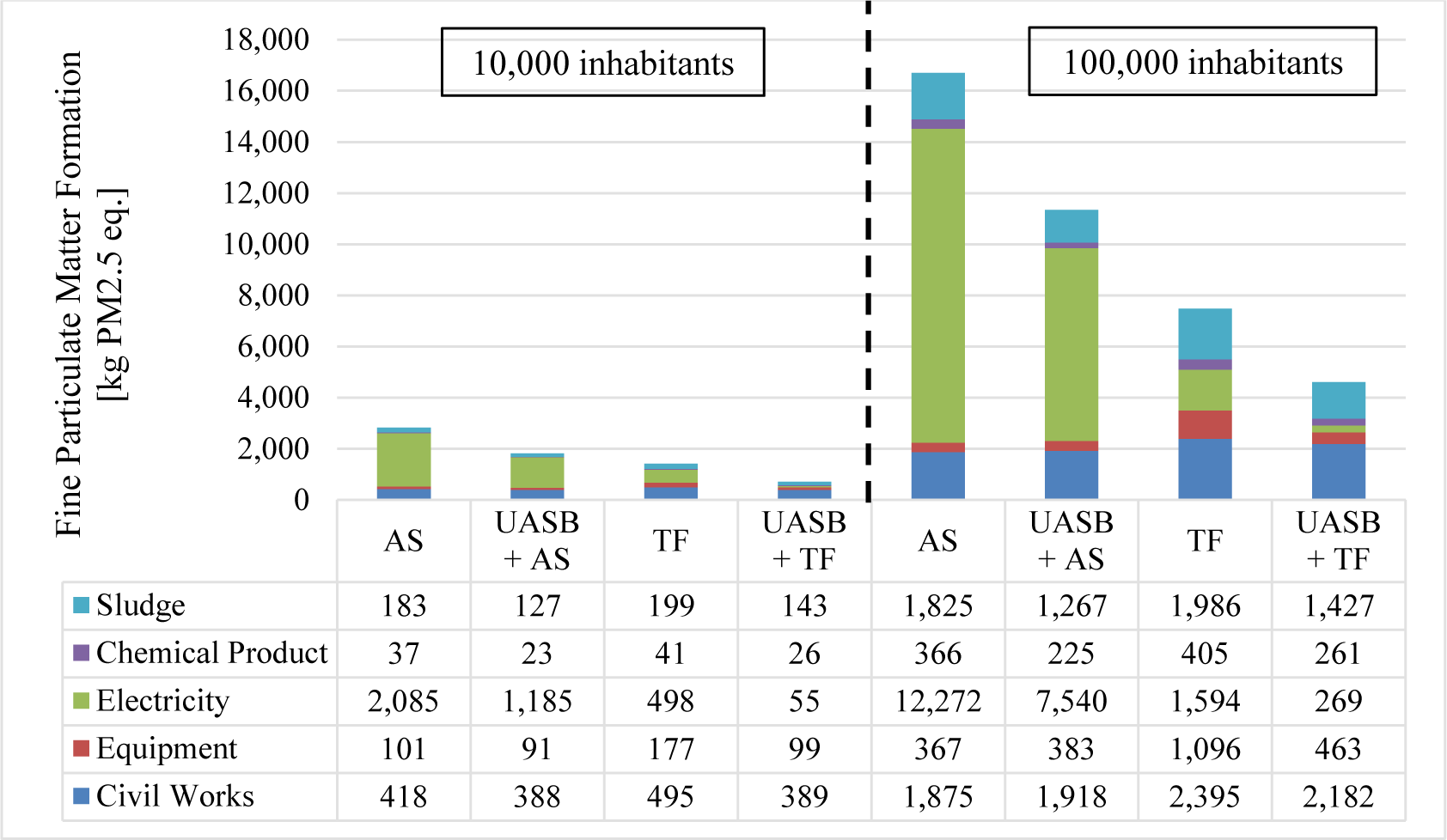
Contribution of the components of WWTPs to Fossil Resource Scarcity
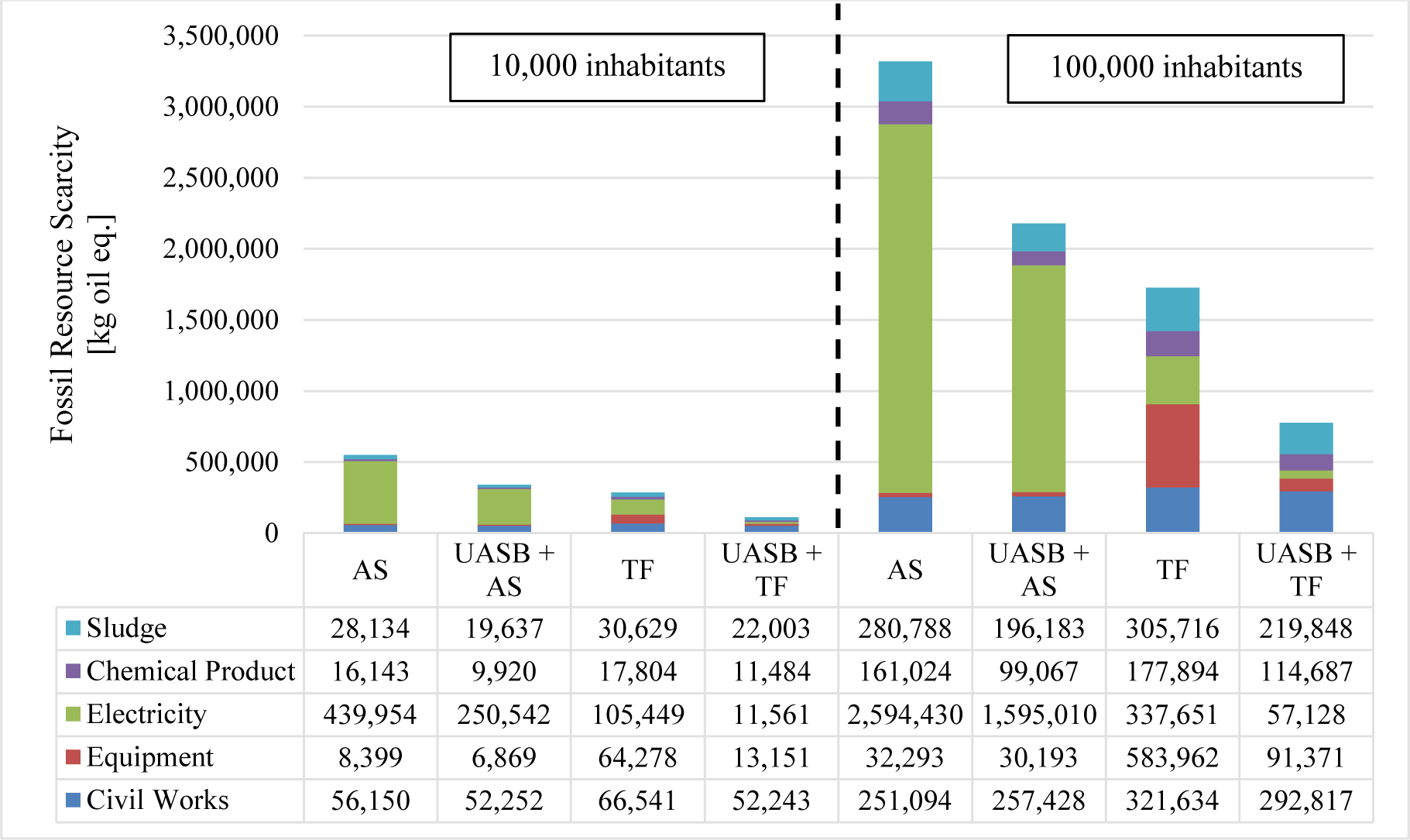
Electricity consumption is the primary factor for Global Warming, as shown in Figure 3, similar to previous impact categories. Figure 4 details that equipment and civil works are the primary sources of Human Carcinogenic Toxicity, unlike previous impact categories.
Contribution of the components of WWTPs to Global Warming
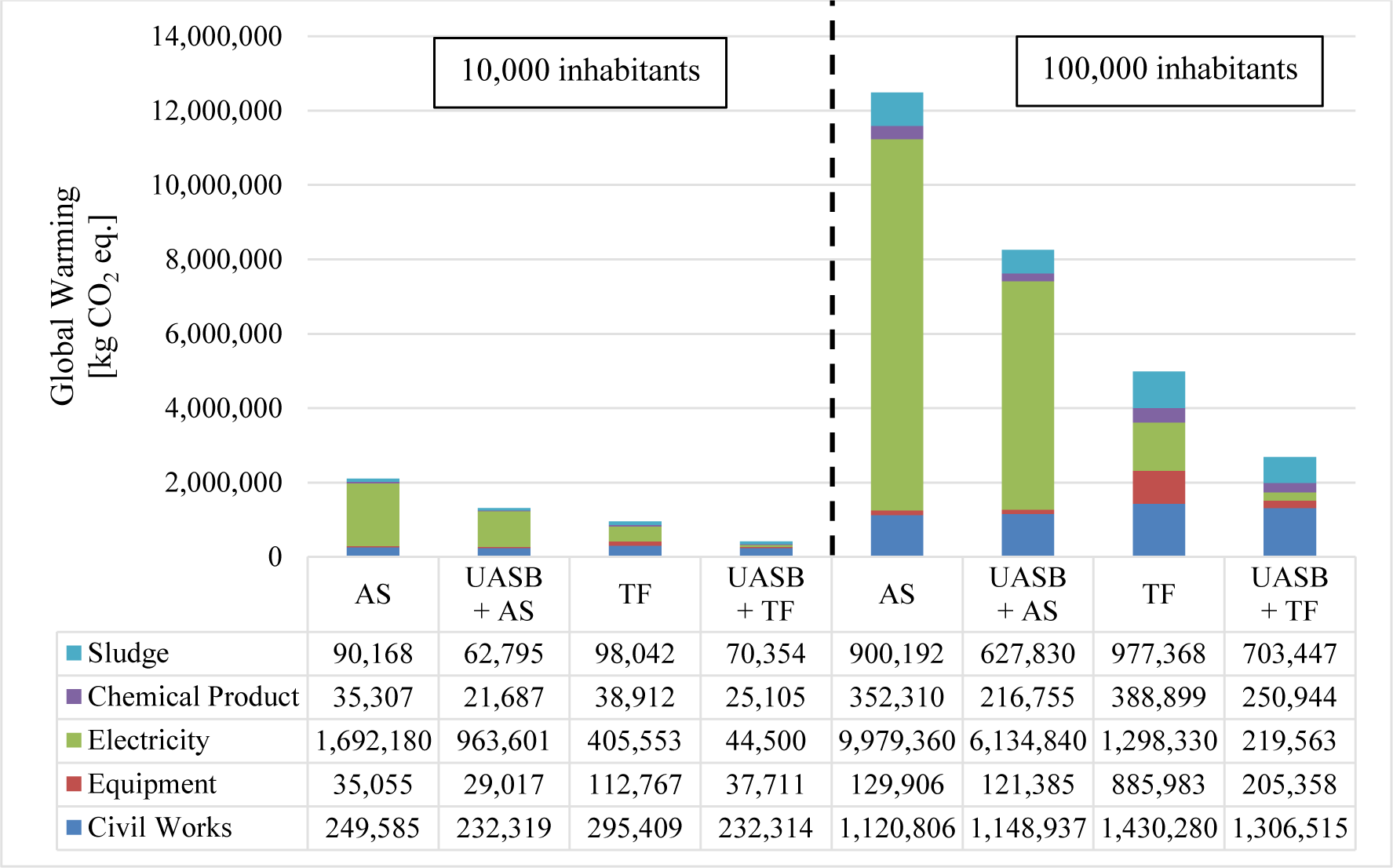
Contribution of the components of WWTPs to Human Carcinogenic Toxicity
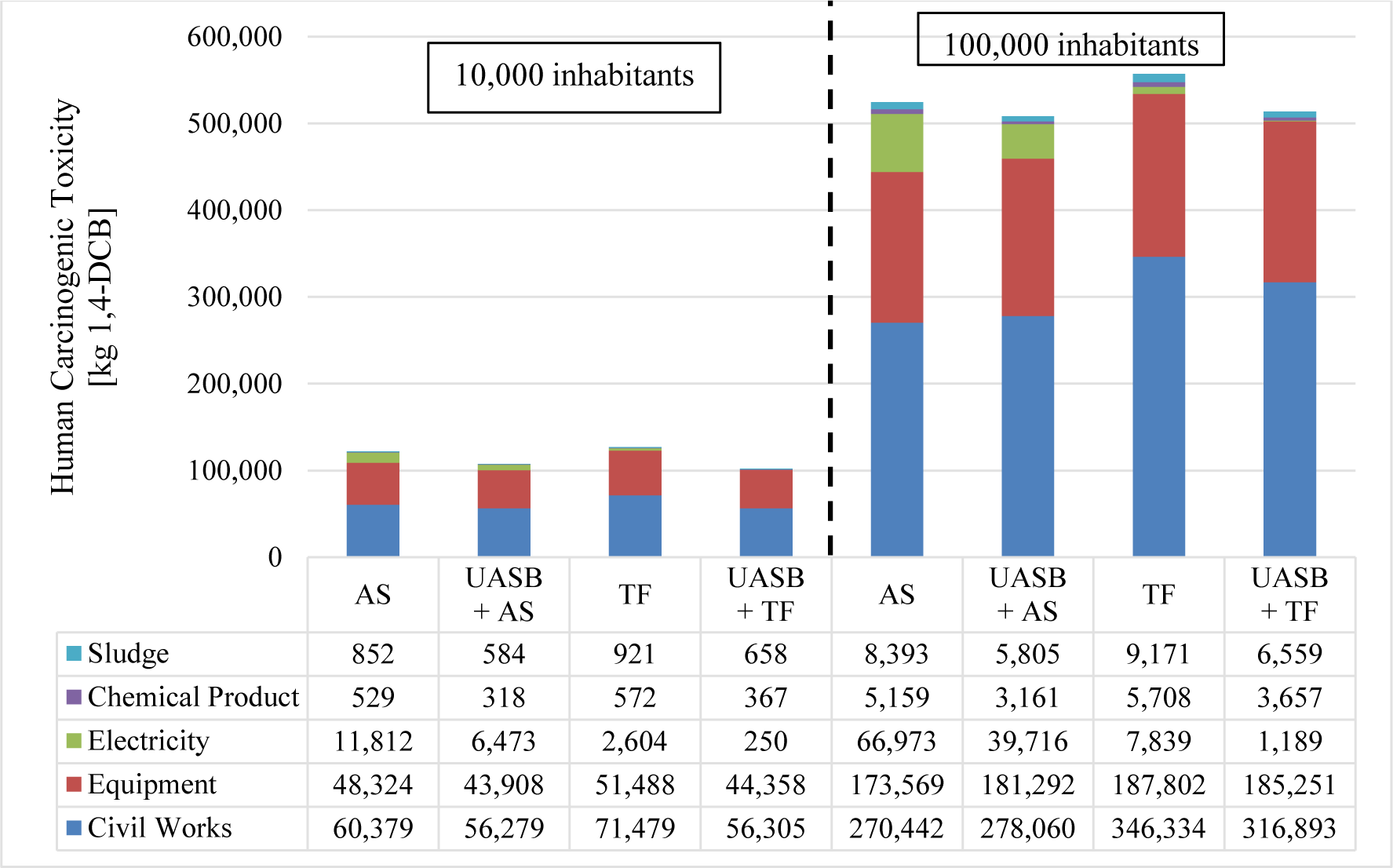
Figure 5 shows that electricity consumption is the main driver of the Land Use impact category. Electricity consumption remains significant in Ozone Formation – Terrestrial Ecosystem, but sludge management and civil works are also relevant, as shown in Figure 6.
Contribution of the components of WWTPs to Land Use
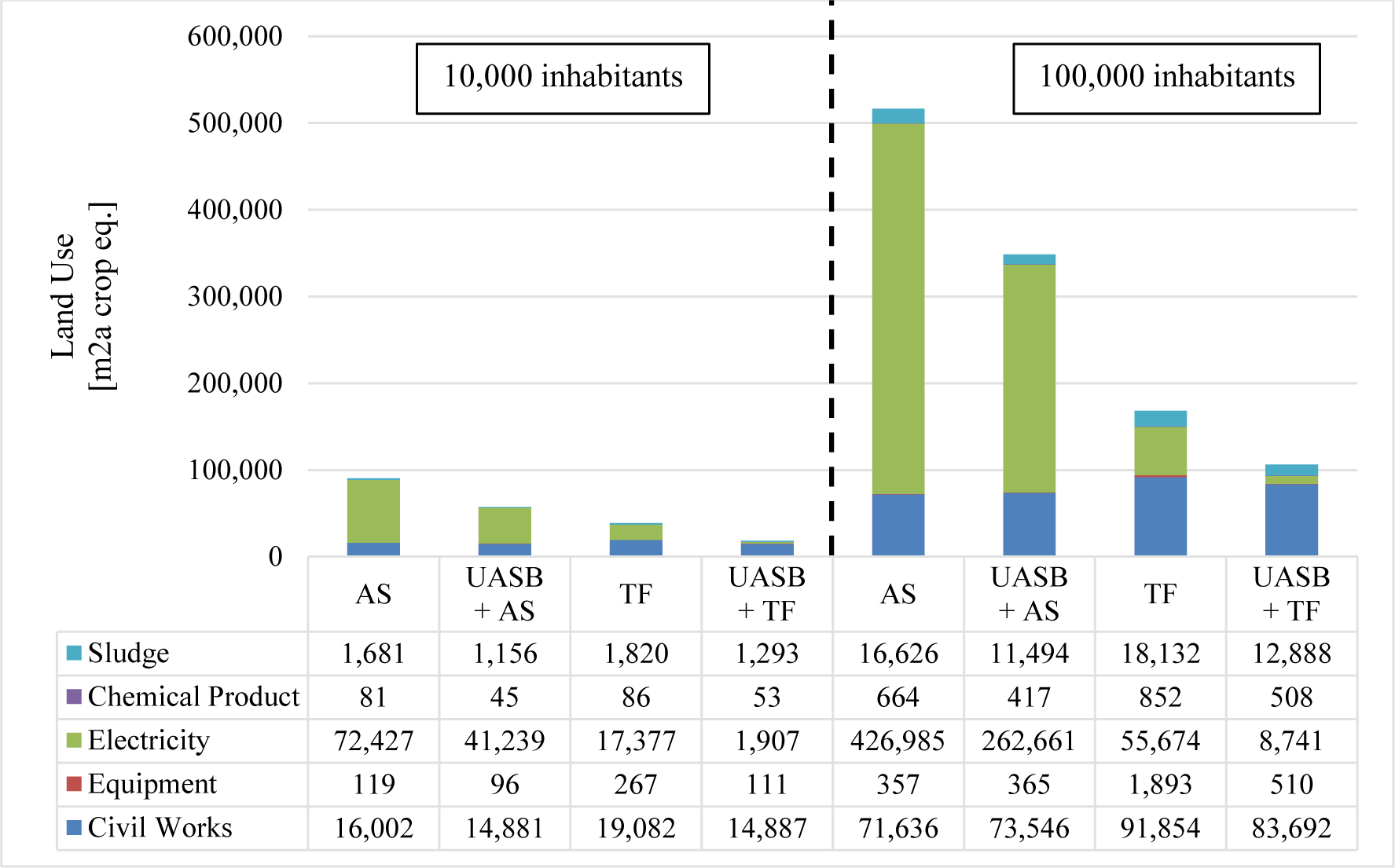
Contribution of the components of WWTPs to Ozone Formation – Terrestrial Ecosystem
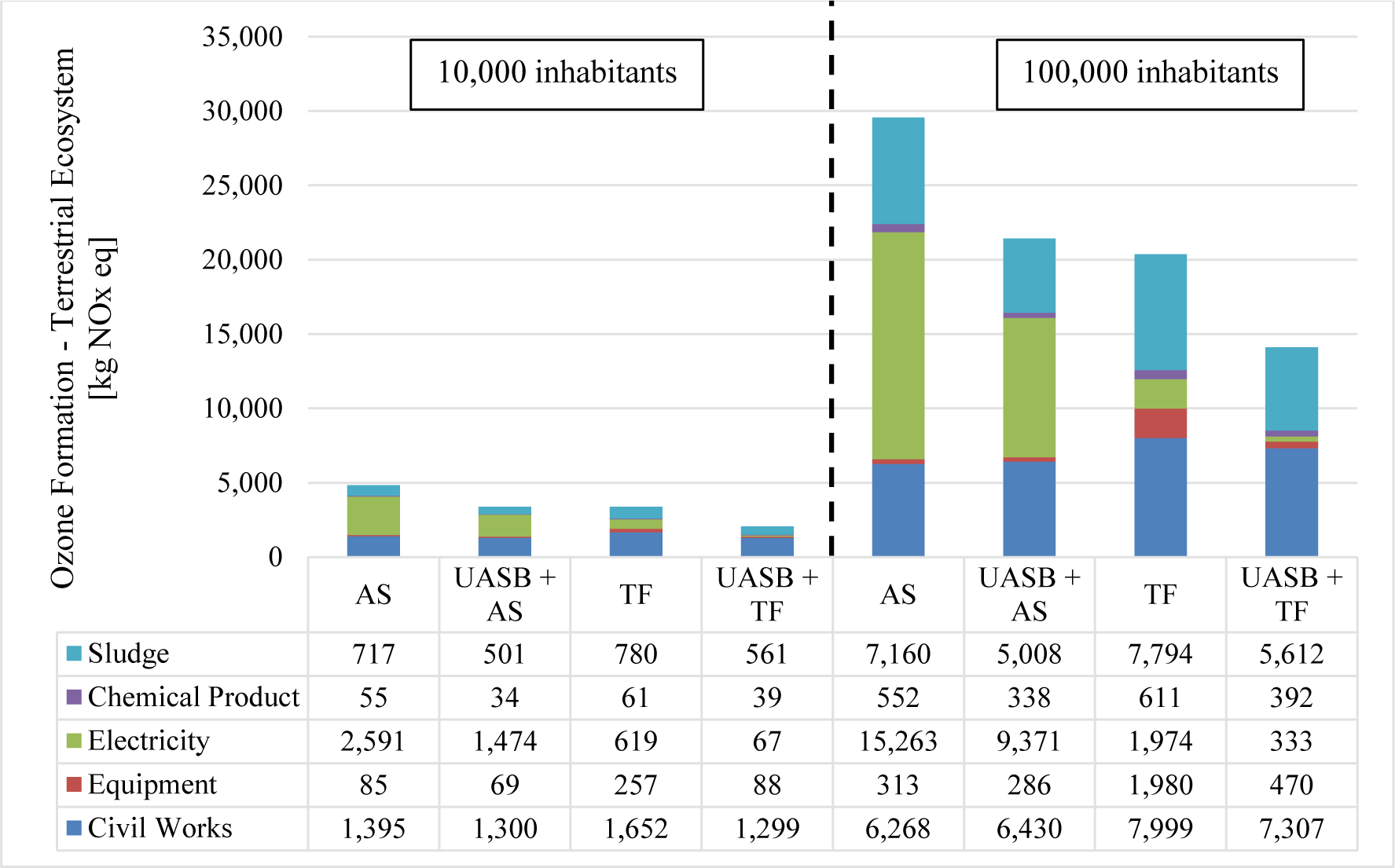
According to Figure 7, electricity consumption is the primary contributor to Terrestrial Acidification. Electricity consumption is even more prominent in Water Consumption, as shown in Figure 8.
Contribution of the components of WWTPs to Terrestrial Acidification
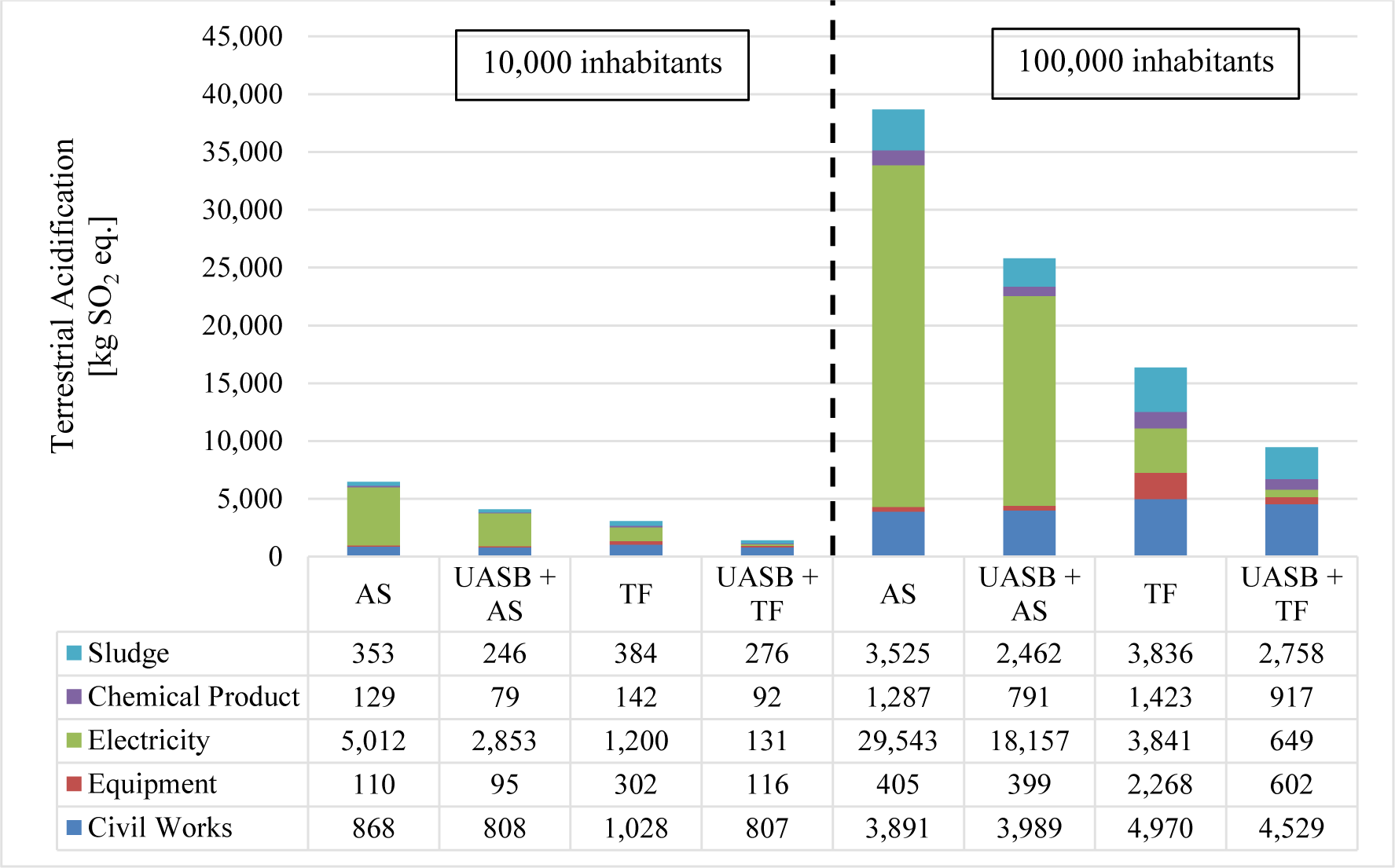
Contribution of the components of WWTPs to Water Consumption
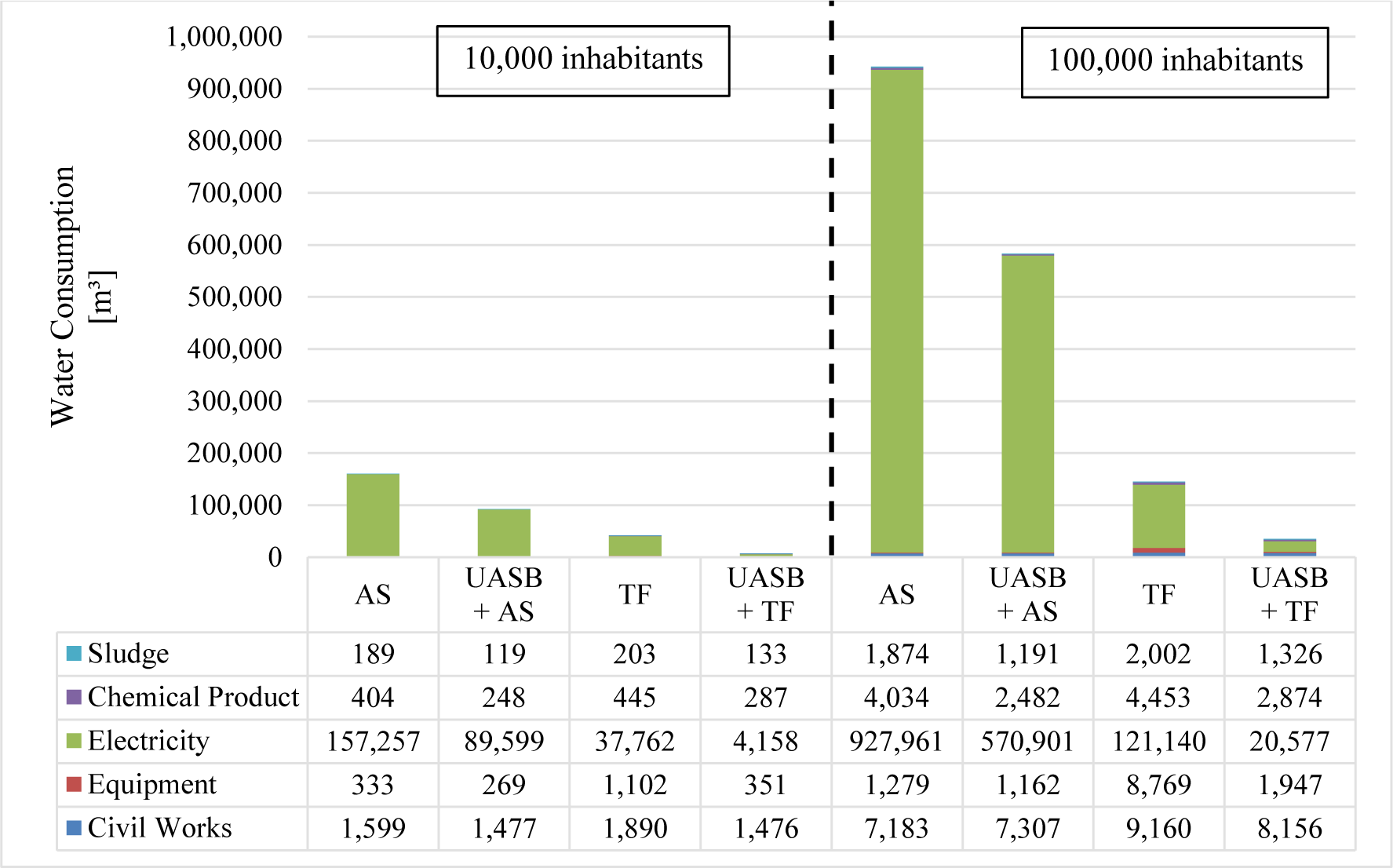
The figures above show that, for both scenarios, the UASB reactor followed by the trickling filter (UASB + TF) stands out as the solution with the fewest environmental impacts in all categories, except for Human Carcinogenic Toxicity in 100,000 inhabitants. The discrepancy is also worth noting when comparing USAB + TF to other WWTPs, except for Human Carcinogenic Toxicity, reaching reductions of 30.67% to 96.30%.
Results clearly show the UASB reactor's positive contribution to the technological routes since the UASB leads to lower impacts in all configurations studied. The UASB reactors promote reductions in the generation of environmental impacts in the following proportions: 32.15−49.54% for Fine Particulate Matter Formation, 34.39−61.21% for Fossil Resource Scarcity, 33.91−56.87% for Global Warming, 3.15−19.77% for Human Carcinogenic Toxicity, 32.50−52.76% for Land Use; 27.49−39.00% for Ozone Formation – Terrestrial Ecosystem; 33.25%−53.47% for Terrestrial Acidification, and 38.13−84.53% for Water Consumption. This fact is mainly due to the lower electricity consumption that the UASB reactors provided in the mentioned technological routes since electricity is one of the main factors responsible for generating environmental impacts. Also, there is an opportunity for these wastewater treatment technologies to reduce their impacts even further through the recovery and use of biogas to generate electricity to turn plants less dependent on external electricity consumption. Such a strategy becomes extremely important to mitigate most environmental impacts.
In this sense, it is important to mention that the environmental impacts generated by electricity consumption are linked to the characteristics of the energy matrix considered in this study: Brazil, more specifically, the southeastern grid. Therefore, the environmental impacts generated by electricity consumption can be greater or lesser in other countries, depending on the energy matrix of each location. That said, it is important to note the influence of the Brazilian energy matrix on Water Consumption since electricity predominates, as the energy matrix is mainly composed of hydroelectric plants, with water use for electricity generation being considered consumption. However, water cannot be removed from the water body.
Furthermore, results show that the construction phase cannot be neglected in LCAs of WWTPs. Except for Water Consumption, its generation of environmental impacts is relevant, especially in Human Carcinogenic Toxicity and in technological routes where energy consumption is lower, notably TF and UASB + TF, which makes it necessary to optimise the construction of these WWTPs to mitigate such impacts.
On the other hand, it was noted that the operating stage of wastewater treatment processes that consume more electricity, specifically AS and UASB + AS, contribute in a greater proportion to the generation of environmental impacts, except for Human Carcinogenic Toxicity. Thus, the focus should be on the operating stage to mitigate the environmental impacts generated by these types of WWTPs.
It is also important to comment on the contribution of the TF equipment for the 100,000 inhabitants scale. The high environmental impact here is due to a large amount of packing medium consisting of plastic rings. Therefore, it would be pertinent that other studies use LCA to evaluate the different possibilities of packing medium and their environmental impacts to optimise this type of wastewater treatment technology.
Lastly, one can notice the predominance of civil works for generating environmental impacts for all wastewater treatment technologies in all scenarios, except for TFs in both scales and the impact categories: Fossil Resource Scarcity and Water Consumption because of the packing medium. The main processes of civil works that contribute to the generation of environmental impacts in all the WWTPs in the considered scenarios are the production of concrete, reinforcing steel and diesel consumption in building machinery. Furthermore, there are also significant contributions due to transport, low-alloyed steel, and sand.
While the processes of equipment contribute generally to a lesser extent, some cases stand out, notably packing medium for TFs, stainless steel, and reinforcing steel. Therefore, strategies aimed at optimising civil works would be the most relevant to reduce the environmental impacts of WWTPs.
At the operational stage, it can be observed that electricity consumption leads to high negative impacts in most categories, especially those routes with high electricity demand (AS and UASB + AS). On the other hand, in WWTPs that consume less electricity, mainly UASB + TF, sludge management is highlighted for the generation of environmental impacts, except for Water Consumption.
In all WWTPs, disposal in sanitary landfills is the main contributor to sludge management impacts for Fine Particulate Matter Formation, Ozone Formation - Terrestrial Ecosystem, and Terrestrial Acidification. Disposal in sanitary landfills and transport contributed similarly to Fossil Resource Scarcity, Global Warming, Human Carcinogenic Toxicity, and Water Consumption. Finally, there is Land Use, in which transport was the main contributor. The disposal in sanitary landfills contributed positively since the processes of the Ecoinvent database, which represent the dispositions of soil and waste in inert material landfills and sanitary landfills, respectively, consider that their locations can be transformed into natural areas, such as forests.
It is important to remember that the results were obtained considering a lifespan of 20 years, so if the time horizon were increased, the environmental impacts of the operational phase would be even greater and more relevant than those of the construction phase. Finally, results show that direct proportionality cannot be applied to estimate the environmental impacts of WWTPs with similar technological routes but different capacities. The impact categories considered showed that a 10-fold increase in scale does not automatically lead to a 10-fold increase in the respective impact categories. Larger WWTPs likely gain scale with their units and equipment, reducing their environmental impacts.
Regarding CAPEX and OPEX of WWTPs (Figure 9 and Figure 10), it can be seen that, in both scenarios, the presence of UASB reactors in the technological routes contributed positively to the reduction of costs (25.03−38.68%), both in construction (5.93−37.40%) and in operation (35.43−49.68%). It is also observed that the WWTPs with activated sludge had higher operating costs, mainly due to electricity consumption. In contrast, the WWTPs with trickling filters had the highest construction costs, mainly due to the acquisition of the packing medium, in this case, plastic rings (1,880.68 R$⸱m−3).
Detailed CAPEX and OPEX of wastewater treatment plants for 10,000 inhabitants
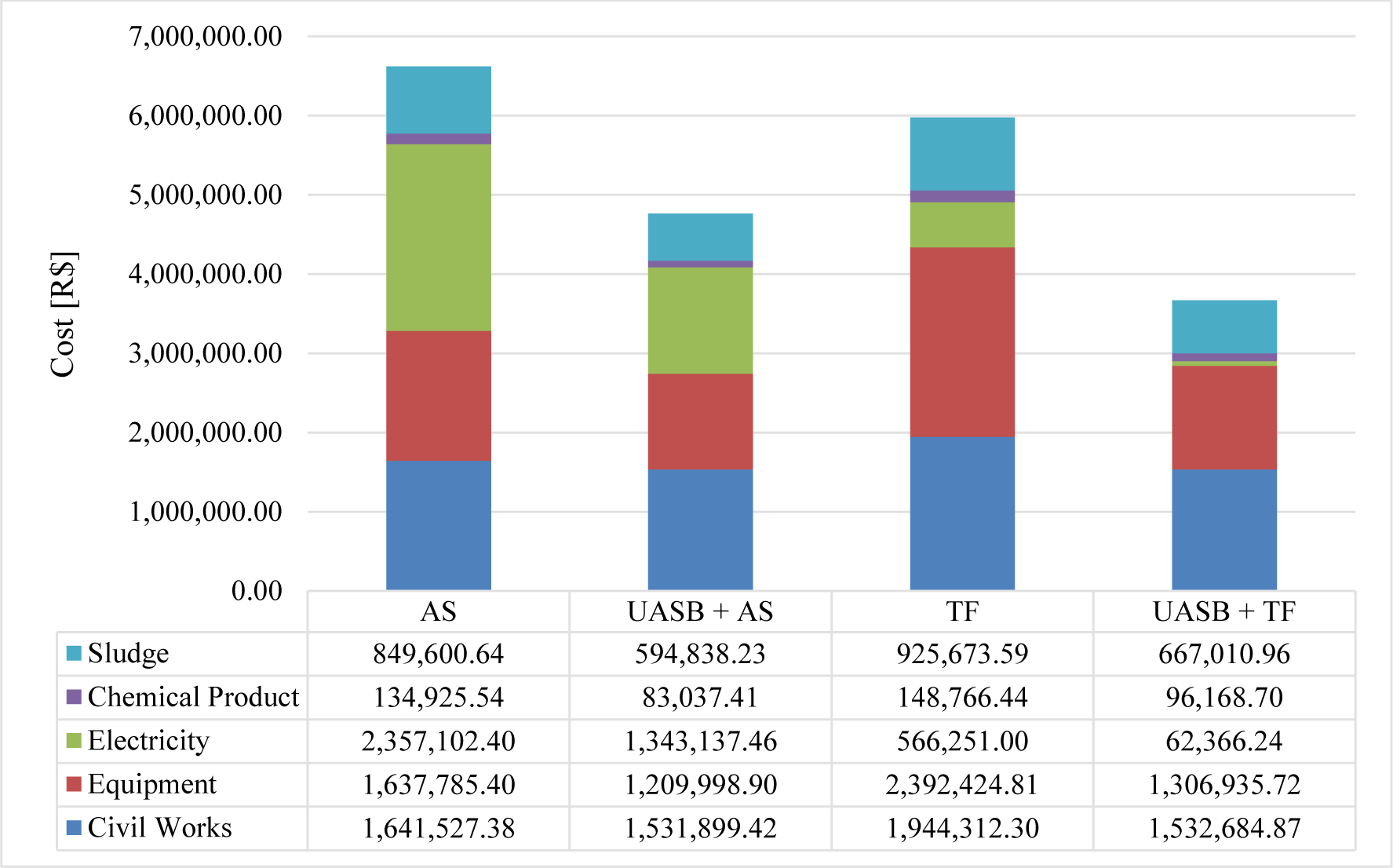
In this context, the technological route comprising the UASB reactor followed by the trickling filter (UASB + TF) stood out since its total costs were the lowest in both scenarios. Although its CAPEX is not the lowest of the alternatives, because of the packing medium's costs, its OPEX is smaller since it consumes little electrical energy in its processes. Considering both scenarios, the total costs of this wastewater treatment plant achieved savings ranging from 21.25% to 44.64%.
Detailed CAPEX and OPEX of wastewater treatment plants for 100,000 inhabitants
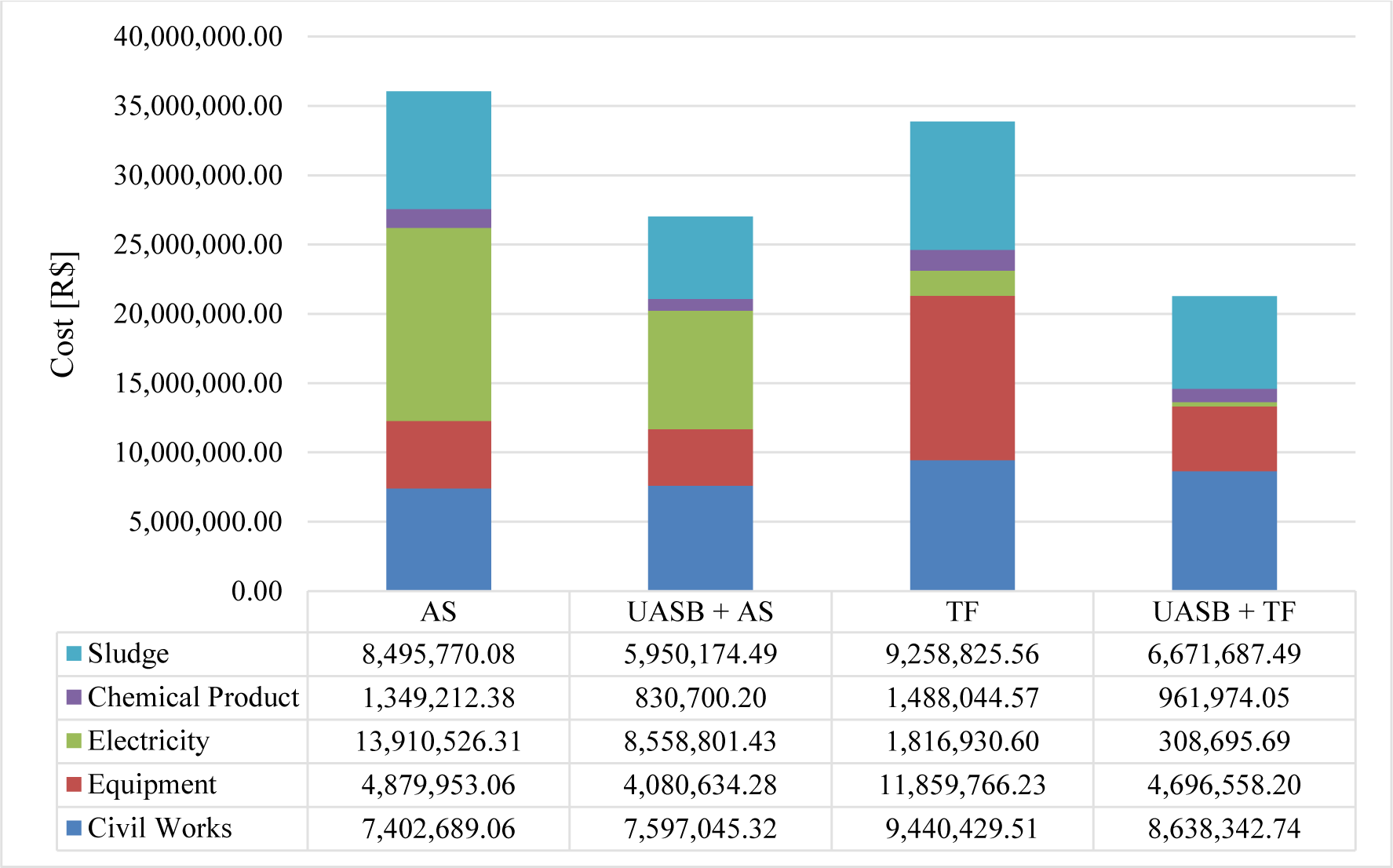
Another point to be noted is the percentage of CAPEX contribution in the total costs of smaller WWTPs, being much more representative than in larger ones. This fact may be due to the gain in the scale of the units and equipment of large WWTPs. However, it is important to remember that the lifespan considered for the WWTPs was 20 years, so if longer lifespans were considered, the percentage of CAPEX contribution to total costs would be lower.
Based on Figure 9 and Figure 10, it is noted that chemical product consumption contributes little to the OPEX of the WWTPs with activated sludge (AS and UASB + AS). On the other hand, in the WWTPs where the consumption of electricity is not so expressive (TF and UASB + TF), the chemical product appears as a slightly more important contributor. This result is because chemical product costs are directly related to the sludge costs since the same polyelectrolyte dosage was adopted for the WWTPs. Therefore, chemical product consumption was higher when there was a greater sludge generation.
In this scenario, it is understood that the positive contribution of UASB reactors in the flowcharts of the WWTPs refers to the reduction of electricity consumption (38.47−88.99%) and sludge management (28.97−31.12%). It also reduces the volumes of the units after the reactors and eliminates some units and equipment of sludge treatment, leading to a reduction in construction (5.93−37.40%) and operating costs (35.43−49.68%). These advantages align with those described in [5], [6].
Lastly, another point worth mentioning is that direct proportionality cannot be applied to estimate the costs of WWTPs with similar technological routes but different capacities. CAPEX and OPEX showed that the cost increase is not a linear correlation with the plant scale. As previously mentioned, larger WWTPs likely gain scale with their units and equipment, reducing their costs. This statement cannot be applied to sludge and chemical costs since sludge generation and polyelectrolyte consumption were calculated based on similar factors, independent of the WWTPs' capacities. Such factors are important to modularising wastewater treatment plants, a common practice. The results of this study show that a cost-benefit study is necessary before applying this practice.
Given the above and Table 2, the technological route that obtained the best performance both in the environmental and economic scope in both scenarios studied was the UASB reactor followed by a trickling filter (UASB + TF). On the other hand, activated sludge (AS) had the worst environmental and economic performance in both scenarios studied.
Environmental and economic impacts per inhabitant of wastewater treatment plants
Impact per Inhabitant | 10,000 inhabitants | 100,000 inhabitants | ||||||
---|---|---|---|---|---|---|---|---|
AS | UASB + AS | TF | UASB + TF | AS | UASB + AS | TF | UASB + TF | |
Fine Particulate Matter Formation [kgPM2.5eq⸱inhab.−1] | 0.28 | 0.18 | 0.14 | 0.07 | 0.17 | 0.11 | 0.07 | 0.05 |
Fossil Resource Scarcity [kg oil eq⸱inhab.−1] | 54.88 | 33.92 | 28.47 | 11.04 | 33.20 | 21.78 | 17.27 | 7.76 |
Global Warming [kgCO2eq⸱inhab.−1] | 210.23 | 130.94 | 95.07 | 41.00 | 124.83 | 82.50 | 49.81 | 26.86 |
Human Carcinogenic Toxicity [kg 1,4-DCB⸱inhab.−1] | 12.19 | 10.76 | 12.71 | 10.19 | 5.25 | 5.08 | 5.57 | 5.14 |
Land Use [m²a crop eq⸱inhab.−1] | 9.03 | 5.74 | 3.86 | 1.83 | 5.16 | 3.48 | 1.68 | 1.06 |
Ozone Formation − Terrestrial Ecosystem [kg NOx eq⸱inhab.−1] | 0.48 | 0.34 | 0.34 | 0.21 | 0.30 | 0.21 | 0.20 | 0.14 |
Terrestrial Acidification [kg SO2 eq⸱inhab.−1] | 0.65 | 0.41 | 0.31 | 0.14 | 0.39 | 0.26 | 0.16 | 0.09 |
Water Consumption [m³⸱inhab.−1] | 15.98 | 9.17 | 4.14 | 0.64 | 9.42 | 5.83 | 1.46 | 0.35 |
CAPEX [R$⸱inhab.−1] | 327.93 | 274.19 | 433.67 | 283.96 | 122.83 | 116.78 | 213.00 | 133.35 |
OPEX [R$⸱inhab.−1] | 334.16 | 202.10 | 164.07 | 82.55 | 237.56 | 153.40 | 125.64 | 79.42 |
Regarding the UASB reactor followed by activated sludge (UASB + AS) and trickling filter (TF), the first obtained the best economic performance, and the second achieved the best environmental performance in both scenarios. Therefore, the choice of wastewater treatment technology should consider the trade-offs among environmental and economic impacts.
This article aimed to comparatively evaluate the environmental and economic impacts of wastewater treatment plants commonly used in Brazil through environmental life cycle assessment and life cycle costing.
It can be concluded that the positive contribution of UASB reactors in the flowcharts of wastewater treatment plants to reduce the generation of environmental and economic impacts, contributing positively to the reduction of the generation of environmental impacts (3.15−84.53%, depending on the impact category) and the reduction of costs (25.03−38.68%).
It is also understood that the technological route that obtained the best environmental and economic performance is the UASB reactor, followed by a trickling filter. In contrast, the one that achieved the worst performance was activated sludge.
Finally, it is inferred that linearly estimating the environmental and economic impacts of similar wastewater treatment technologies is impossible when the capacities are different.
Comprehensive life cycle inventories of alternative wastewater treatment systems ,Water Res , Vol. 44 (5),pp 1654–1666 , 2010, https://doi.org/https://doi.org/10.1016/j.watres.2009.11.031
, - https://pesquisa.in.gov.br/imprensa/jsp/visualiza/index.jsp?data=18/03/2005&jornal=1&pagina=58&totalArquivos=192 , 2005,
- https://pesquisa.in.gov.br/imprensa/jsp/visualiza/index.jsp?data=16/05/2011&jornal=1&pagina=89&totalArquivos=132 , 2011,
- https://www.sema.rs.gov.br/upload/arquivos/201707/19110149-355-2017-criterios-e-padroes-de-emissao-de-efluentes-liquidos.pdf , 2017,
- , Treatment of Domestic Wastewater (In Portuguese), 2017
Biological Wastewater Treatment in Warm Climate Regions Volume One , Vol. 1 , 2005, https://doi.org/https://doi.org/10.2166/9781780402734
, Biological Wastewater Treatment in Warm Climate Regions Volume Two , Vol. 2 , 2005, https://doi.org/https://doi.org/10.2166/9781780402703
, Selection of an appropriate wastewater treatment technology: A scenario-based multiple-attribute decision-making approach ,J Environ Manage , Vol. 113 ,pp 158–169 , 2012, https://doi.org/https://doi.org/10.1016/j.jenvman.2012.08.025
, Environmental and cost life cycle assessment of different alternatives for improvement of wastewater treatment plants in developing countries ,Science of the Total Environment , Vol. 660 ,pp 57–68 , 2019, https://doi.org/https://doi.org/10.1016/j.scitotenv.2018.12.386
, Life cycle assessment applied to wastewater treatment: State of the art ,Water Res , Vol. 47 (15),pp 5480–5492 , 2013, https://doi.org/https://doi.org/10.1016/j.watres.2013.06.049
, Sustainability assessment of sludge and biogas management in wastewater treatment plants using the LCA technique ,Ambiente e Agua - An Interdisciplinary Journal of Applied Science , Vol. 14 (5),pp 1 , 2019, https://doi.org/https://doi.org/10.4136/ambi-agua.2371
, - Sustainable development goals, 2021, https://www.un.org/sustainabledevelopment
- Thematic Diagnosis - Water and Sewer Services - Overview, 2022, https://arquivos-snis.mdr.gov.br/DIAGNOSTICO_TEMATICO_VISAO_GERAL_AE_SNIS_2022.pdf
- , , 2020
Overview of sewage treatment in the South, Southeast and Midwest regions of Brazil: most employed technologies , Vol. 66 (213),pp 5–19 , 2018, https://doi.org/https://doi.org/10.4322/dae.2018.028
, Contribution for improving the design, construction and operation of UASB reactors treating sewage – Part 1: Topics of Interest , Vol. 66 (214),pp 5–16 , 2018, https://doi.org/https://doi.org/10.4322/dae.2018.038
, Holistic analysis of urban water systems in the Greater Cincinnati region: (1) life cycle assessment and cost implications , Vol. 2 ,pp 1–11 , 2019, https://doi.org/https://doi.org/10.1016/j.wroa.2018.100015
, - Life Cycle Costing - state of the art report’, ICLEI – Local Governments for Sustainability, 2017, http://www.sppregions.eu/fileadmin/user_upload/Life_Cycle_Costing_SoA_Report.pdf
- , Environmental management – Life cycle assessment – Principles and framework, 2006
- , Environmental management – Life cycle assessment – Requirements and guidelines, 2006
- , Buildings and constructed assets – Service life planning – Part 5: Life-cycle costing, 2017
The application of life cycle assessment (LCA) to wastewater treatment: A best practice guide and critical review ,Water Res , Vol. 184 ,pp 116058 , 2020, https://doi.org/https://doi.org/10.1016/j.watres.2020.116058
, Life cycle cost analysis of wastewater treatment: A systematic review of literature ,J Clean Prod , Vol. 310 ,pp 127549 , 20202021, https://doi.org/https://doi.org/10.1016/j.jclepro.2021.127549
, Life cycle assessment of wastewater treatment in developing countries: A review ,Water Res , Vol. 153 ,pp 63–79 , 2019, https://doi.org/https://doi.org/10.1016/j.watres.2019.01.010
, - , Hydraulic and sanitary engineering design for wastewater treatment plants (In Portuguese), 2011
- , Wastewater Engineering: Treatment and Resource Recovery, 2013
- Wastewater Treatment and Discharge, 2019, https://www.ipcc-nggip.iges.or.jp/public/2019rf/pdf/5_Volume5/19R_V5_6_Ch06_Wastewater.pdf
- , Biological Treatment of Solid Waste, 2006 IPCC Guidelines for National Greenhouse Gas Inventories - Volume 5: Waste, 2006
Performance of activated sludge processes and reliability-based design ,Water Pollution Control Federation , Vol. 51 (12),pp 2841–2857 , 1979
, Reliability analysis of wastewater treatment plants ,Water Res , Vol. 42 ,pp 4–5 ,pp 1182–1194 , 2008, https://doi.org/https://doi.org/10.1016/j.watres.2007.09.001
, - , Septic tank - Units for treatment and disposal of liquid effluents - Project, constrution and operation (In Portuguese), 1997
- , Design of sewer network - Procedure (In Portuguese), 1986
- Normative Deliberation COPAM/CERH-MG No. 08 (In Portuguese), 2022, http://www.siam.mg.gov.br/sla/download.pdf?idNorma=56521
- , Atlas de Esgoto: Limpeza de Bacias Hidrográficas, 2017
- Population Estimates, 2020, https://www.ibge.gov.br/estatisticas/sociais/populacao/9103-estimativas-de-populacao.html?=&t=resultados
- , Termo de referência para elaboração de plano municipal de Saneamento Básico, 2018
Evaluation of different practices to estimate construction inventories for life cycle assessment of small to medium wastewater treatment plants ,J Clean Prod , Vol. 245 ,pp 118768 , 2020, https://doi.org/https://doi.org/10.1016/j.jclepro.2019.118768
, - Brazilian National System of Costs Survey and Indexes of Construction - SINAPI, 2021, https://www.caixa.gov.br/poder-publico/modernizacao-gestao/sinapi/Paginas/default.aspx
- Tariffs, 2021, https://www.aneel.gov.br/tarifas
- General Price Index – Market (IGP-M), 2021, https://portal.fgv.br/noticias/igp-m-resultados-2021