Plastics have gained widespread usage due to their attractive properties, which include affordability, lightweight nature, strength, durability, corrosion resistance, and excellent thermal and electrical insulation capabilities [1]. Moreover, most types of plastic are not naturally biodegradable. Therefore, they are most likely to accumulate in the environment, increasing the threat to our planet [1]. Unlike handling organic waste, the lack of biodegradability makes it difficult to manage plastic waste [2].
Microplastics (MPs) are defined as any synthetic solid particle or polymeric matrix, with regular or irregular shape and a size ranging from 1 μm to 5 mm, of either primary or secondary manufacturing origin, which is insoluble in water [3]. Primary MPs refer to pellets that serve as raw material in the plastics industry. In contrast, secondary MPs comprise small fragments resulting from the degradation and fragmentation of larger plastic items [4].
MPs with particle sizes smaller than 5 mm can threaten the aquatic environment [5]. Some of the ways MPs reach the marine environment include mishandling of plastics, dumping plastic wastes directly in water, mismanaged landfills [6], wastewater treatment plant discharges, industrial and commercial activities, municipal waste collection [7], and agricultural activities [8]. Many processes are believed to transport MPs in the water, including surface drifting, currents, and wind [9].
The presence of MPs in the marine environment has received increased attention lately due to their harmful effects on the biota [10]. MPs can cause physical and/or chemical damage to the biota exposed to a high dose due to their small size and affinity for persistent organic pollutants [11]. MPs are believed to greatly affect marine life in coastal areas. Humans are indirectly affected as they consume marine organisms with ingested MPs, which can pose serious health issues [12]. Further, the presence of MPs in human food decreases its nutritional quality [13]. MPs can be an indirect source of toxicity and can be transported to antagonistic species. These include almost 700 species, from the largest whales to the tiniest zooplanktons [14]. Apart from the polymers, these pollutants may include certain additives such as plasticizers, dyes, flame retardants, metals, and UV filters that are sometimes found to be toxic [15]. MPs can adsorb hydrophobic organic contaminants and heavy metals, resulting in a toxic material mistaken for food by marine organisms [16]. Once ingested, the MPs may threaten marine biota through direct physical interaction, by blocking the digestive tract after ingestion or by transporting and leaching toxic substances [17]. These MPs, when ingested, can also result in a false sensation of satiation, impacting the fitness and reproduction of marine organisms [18].
Currently, MP pollution is spread everywhere across the seas and the oceans and is expected to accumulate in the marine environment if no mitigation plans are implemented. Nowadays, there are different ways in which pollution is detected. These methods include collecting water samples through pumping and using plankton nets, buckets, and samplers. However, in almost all studies, the primary sampling method uses neuston nets with a mesh size of 0.3 mm to 5 mm [19]. After collecting the water samples, they are often analyzed according to the National Oceanic and Atmospheric Administration (NOAA) method [20], in which water samples are filtered using multiple mesh sizes to remove large plastic pieces. After going through the mesh filtration process, the filter paper with the MPs is visually inspected under a microscope to count the number of particles present [20]. These methods are time and labour-intensive, so a reliable and efficient approach to detecting MP pollution is needed.
The application of sensing methods has gained considerable attention in recent years. Sensing techniques offer the advantages of rapid, non-destructive, and real-time analysis, enabling efficient and reliable monitoring of MPs. Remote sensing is obtaining information about the environment without physical contact through interpreting and processing images formed from the electromagnetic radiation reflected from features on the earth's surface [21]. Different objects reflect or emit electromagnetic energy at different wavelength ranges. This spectral response, known as a spectral signature, is used to identify objects through satellite images [22]. If unique spectral signatures are identified for MPs, remote sensing imagery would be an excellent tool to quantitatively determine the levels of MPs in the marine environment. Remote sensing potentially provides the necessary spatial and temporal coverage of the ocean surface for estimating the global abundance of near-surface plastics [15]. It also provides long-term, global monitoring of floating marine plastics [23]. Significant efforts are required to identify the spectral signatures of MPs in the water column to calibrate satellite signals, translate the signals into a format compatible with in-situ data, and correct various biases to achieve a successful application. Remote sensing tools are still not extensively adapted for detecting and monitoring MPs compared to other well-established pollutants. However, one study specifically investigated the application of remote sensing modelling in predicting the coastal accumulation of MPs emitted by the PO River in Italy [24]. They found that the sensing modelling approach aligns with the findings from the hydrodynamic modelling. However, hydrodynamic modelling revealed that the discharged particle amount is only semi-coupled to beaching rates [24].
The present study is a rare contribution to the existing literature as it addresses the challenges of detecting MPs using remote sensing tools. Currently, limited studies are focusing on this specific research area, and the present study provides valuable insights and lays the groundwork for further investigations into the application of remote sensing for MP detection. This research aimed to develop a method for detecting visible MP pollution (1-5 mm) in the coastal waters of Dubai with a monitoring potential. The objectives of the research are: (a) determine the spectral signature of synthetic MPs mixed with water samples using a Stellar-RAD® handheld spectrometer in the laboratory, (b) determine levels of MPs in water samples collected from the Dubai coast, and (c) use the determined spectral signature of MPs in the lab to determine levels of MPs in the Dubai coast from satellite imagery. The main objective of this study was to quantify the levels of MPs along the coast of Dubai through the analysis of water samples. No studies have evaluated levels of MPs in the coastal waters of Dubai, a research gap being addressed in this study. Since an extensive analysis of the spatial extent is missing and the detection tools for MP pollution that are available nowadays are inefficient for large areas, there is a need for robust remote detection techniques [15].
This study delves into determining microplastics (MPs) levels in coastal waters through field sampling campaigns, initially collecting water samples during two campaigns along the Dubai coast. Following this, the laboratory-based identification of spectral signatures of MPs is explored, involving an experimental setup with a Stellar-RAD® handheld spectrometer. The correlation between spectral reflectance and various factors, such as types, sizes, and total suspended solids (TSS), is investigated for six common types of MPs. Finally, the research extends to developing an empirical spectral model, utilizing Sentinel-2 imagery to comprehensively analyze and monitor MP pollution in coastal waters.
Water samples were collected for this study in two field campaigns on 15 January 2019 and 10 September 2020 along the coast of Dubai (Figure 1a) on the Arabian Gulf. In the first campaign, coastal water samples were collected from 80 locations on the coast, and in the second campaign, samples were collected from 10 locations. Samples were collected from various beaches along the Dubai coast: Jumeirah Beach, Mamzar Park Beach, Salt Beach, Kite Beach, FS Beach, JBPMTS Beach, JBR Beach, Mercato Beach, Soufouh Beach, and Black Palace Beach. Figure 1b shows a sample Sentinel-2 image of the study area, acquired on 13 January 2022.
Coastal waters MPs sampling locations along the coast of Dubai (a) and Sentinel-2 image of the study area acquired 13 January 2022 (b)
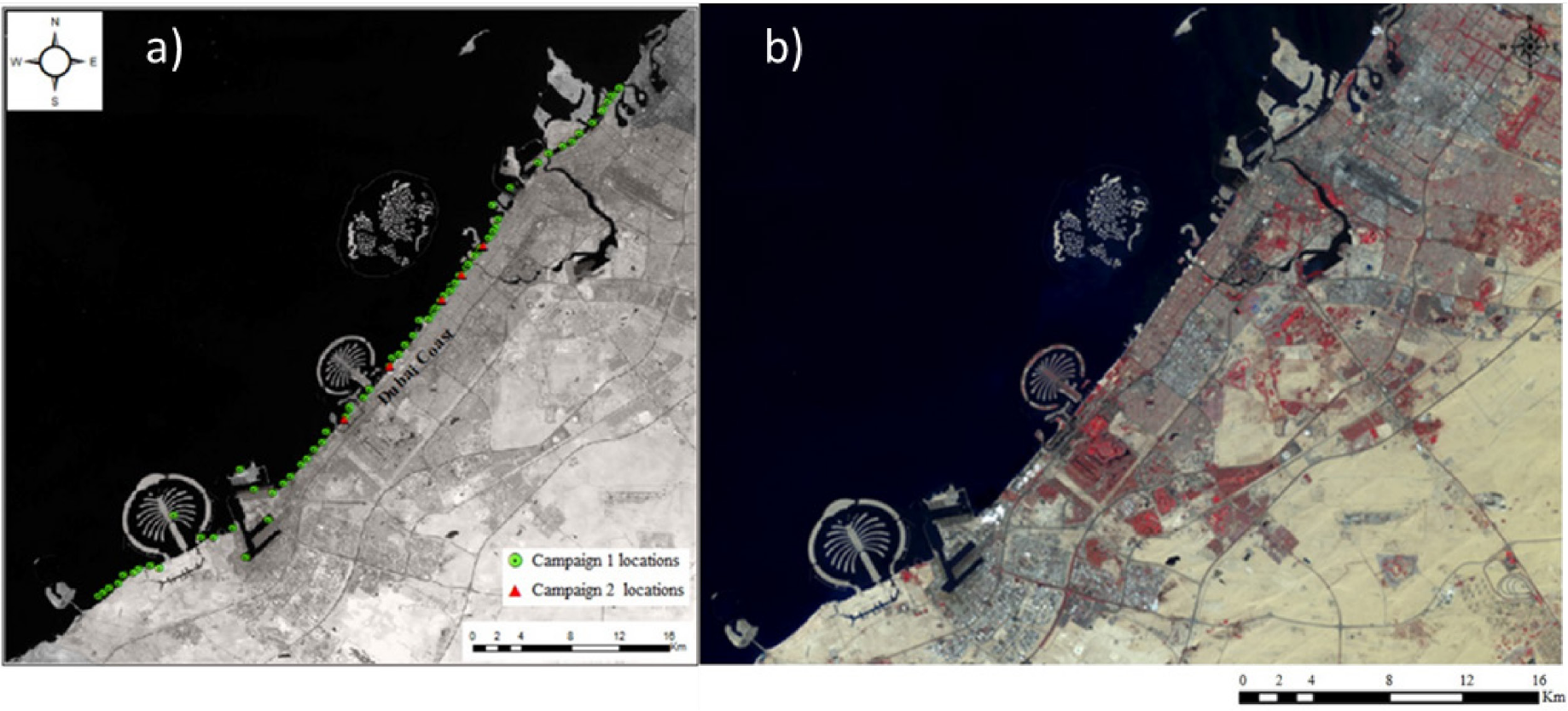
One litre of coastal water was collected from each location using glass beakers. The samples were collected during high tide and labelled according to the sample location's coordinates, date, and sampling time. Sampling was carried out at the surf (or swash) zone, which is believed to be where the MPs are generated [25]. The surface water grab samples were collected and transferred to the lab for further analysis.
The samples were then analyzed to determine the levels of MPs in water using the NOAA method for MPs enumeration in the marine environment [26]. Pretreatment of the samples was performed to eliminate organic and inorganic materials. The samples were rinsed with distilled water to remove salts and then passed through stainless steel mesh sieves to separate and discard any plastic particles greater than 5 mm in size. In the present study, there were no solids greater than 5 mm. Solids collected in the 0.3 mm sieve were transferred to a tared beaker using a spatula and were placed in a drying oven for 24 hours at 60 °C to ensure sample dryness, followed by measurement of the mass of the dried solids.
Wet Peroxide Solution (WPO) was then added to the solids, which were collected in the beaker, followed by adding 20 mL of hydrogen peroxide and heating the mixture to 75 °C on a hotplate. The resulting mixture was filtered using a vacuum filter and 0.45 µm pore size standard filter paper. The filter paper was transferred to a known mass of Petri dish using metal forceps for examination under the microscope with 40 X magnification. The final mass of the MPs on the filter paper was then measured. Subsample of the particles were subjected to final FTIR confirmation as previously described in another study [10].
The reference spectral signatures of the MPs produced in the laboratory were identified through an experimental setup prepared in the laboratory, as shown in Figure 2. A correlation was established by analyzing the spectral reflectance of water samples with synthetic MPs added to them using a Stellar-RAD® handheld spectrometer. The experimental setup included a spectrometer, glass beaker, and ultrapure water with synthetic MPs added to it. Six types of MPs were used in the experiments, including polyethylene (PE), polypropylene (PP), polyvinyl chloride (PVC), polystyrene (PS), polyurethane (PUR), and polyethylene terephthalate (PET), which are the most common types found in the marine environment [27].
Experimental setup for developing a correlation
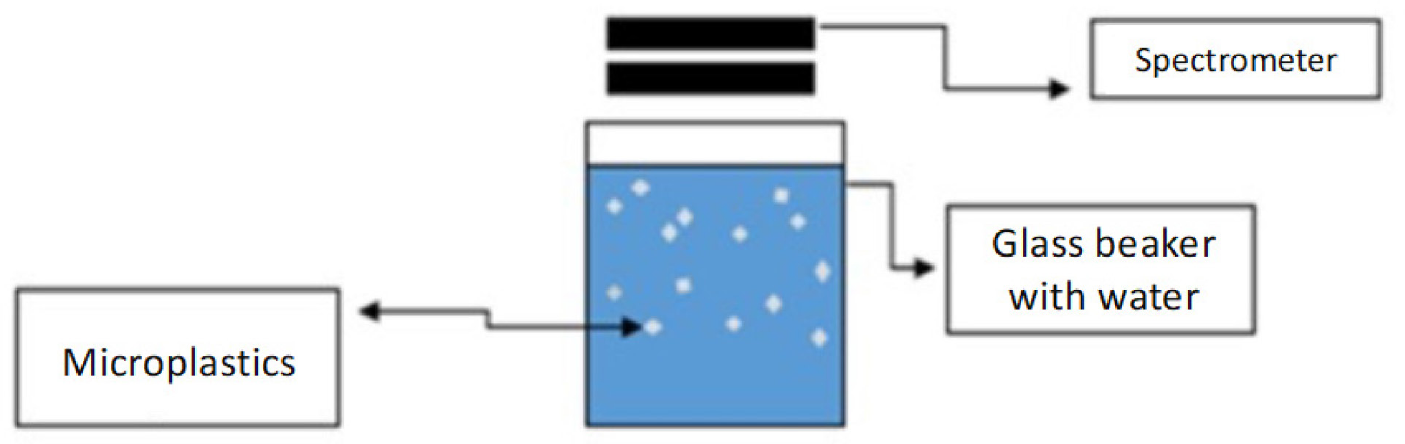
The Stellar-RAD® handheld spectrometer used in this experiment has a wavelength range of 350-1150 nm. Using a spectrometer with such a wavelength range was based on the outcomes of previous research studies. Moroni et al. [28] investigated spectral signatures and the absorption feature characteristics for two polymers, PET and PVC, acquired with a two-linear-spectrometer apparatus. They calculated wavelengths associated with the maxima of reflectance values that have been optimal for both polymers. These points are located at wavelengths of 970 nm, 1090 nm, 1320 nm, and 1580 nm.
The Stellar-RAD® handheld spectrometer is a research-grade spectrometer with a spectral resolution smaller than 1 nm. It was used to measure the spectral reflectance of the MPs in water by measuring the reflectance/emittance of the water with MPs mixed in it and placed in the glass beaker with water. The six types of MPs used in the experiment were created artificially by cutting the plastic sources into smaller than 5 mm pieces. The plastic sources for these six types of common MPs are the following: shampoo bottles from PE, drinking straws from PP, wire and cable sheathing from PVC, egg boxes from PS, shoe soles from PU, and water bottles from PET. All of these MPs are commonly found in coastal water [29].
In the present study, different sizes of plastic materials (5 mm, 2.5 mm, and 1.25 mm) were also used. To simulate real coastal water, which normally includes suspended sediments, different proportions of sand were added to the MPs in the experiment. The experiment was conducted for the six types of MPs mentioned above for three sizes for each type (Figure 3). The spectral reflectance of the clear water was first measured for reference. Literature shows that the TSS value at the surface of deeper water of the Arabian Gulf is about 260 mg/L [30]. Accordingly, for each size and type of the tested MPs, three different amounts of sand were added: 50, 150, and 260 mg to represent TSS in the study area. The used sand was a sediment commonly found in the Dubai coastal waters. For every part of the experiment, three trials were administered to ensure the robustness of the measured spectral reflectance. Therefore, a total number of 234 trials were conducted. These spectral responses were carefully examined and analyzed to develop correlations that will aid in identifying the spectral signatures of the tested types of MPs; however, these specific spectral responses were not directly utilized in the subsequent model development. Having different variables aside from the fixed factors (known volume of water in a glass beaker and a dark room) helped to consider all possibilities and develop a correlation that can help detect MPs. These spectral responses were carefully examined and analyzed to develop correlations that will aid in identifying the spectral signatures of the tested types of MPs; however, these specific spectral responses were not directly utilized in the subsequent model development.
The artificially created microplastics in the lab experiment
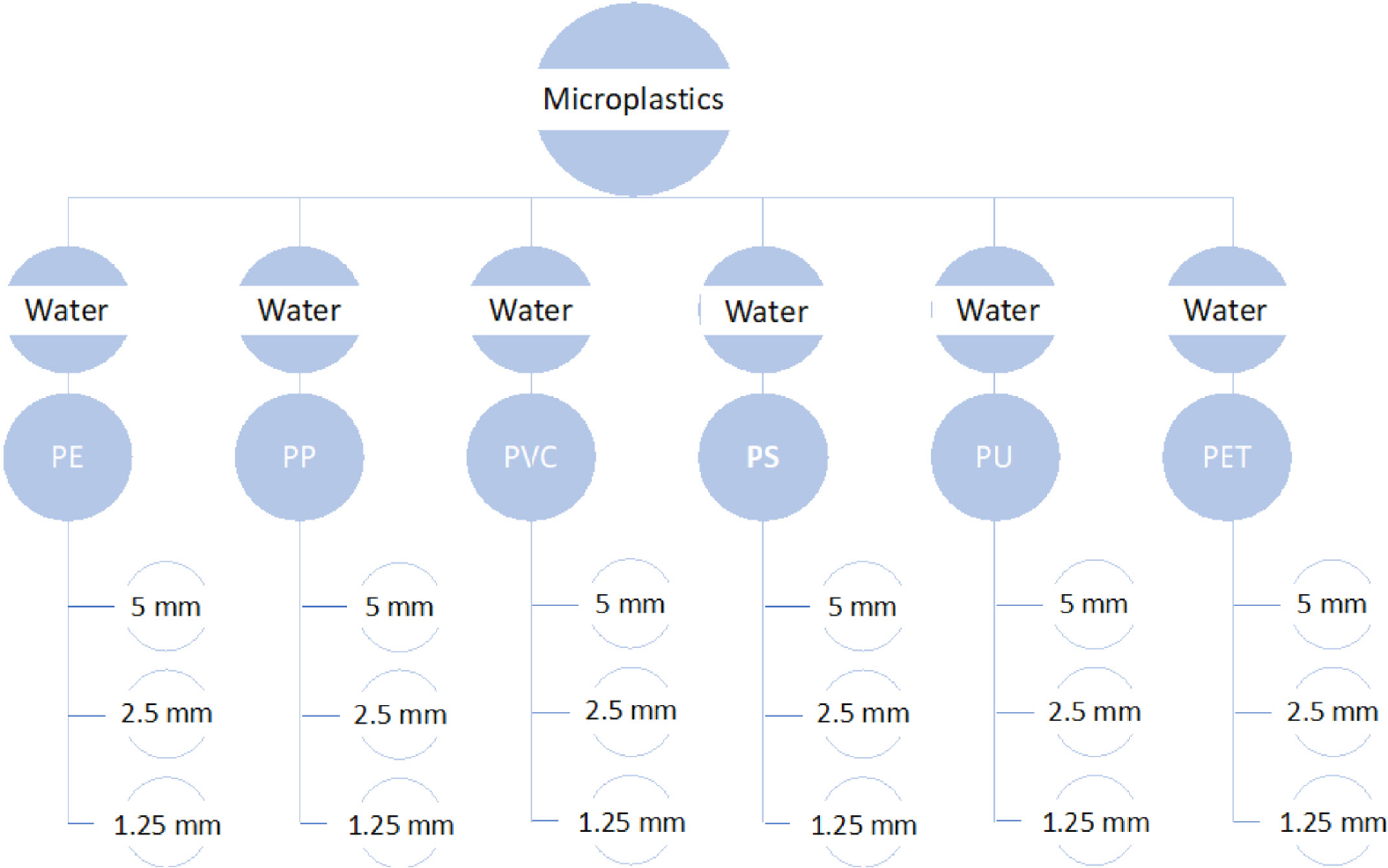
Each type of MP was added to 1 litre of water in the glass beaker. The artificially created MPs were mixed with deionized water in the laboratory inside a glass beaker. The purpose of using glass beakers and rod stirrers instead of plastic is to prevent them from being a source of contamination. The experiment was conducted in a dark place to prevent any interference from any other light source and ensure the accuracy of the results. Spectral responses were measured by the spectrometer for each type and size of MPs used in the experiment. These spectral responses were analyzed to develop the correlation, which was used to identify the spectral signatures of the tested types of MPs. The resulting spectra in these experiments were first smoothed using a 20 nm span average filter followed by derivative spectroscopy analysis to identify absorption features in the mixes created in the laboratory [31]. Then, the spectral reflectance curves for the mixes (water + MPs + TSS) were created.
Sentinel-2 Level-1C imagery was obtained on the two sampling days to develop an empirical spectral model for MPs in coastal water in the study area. Atmospheric correction (considering ozone, water vapour, and cirrus corrections) was applied to the two Sentinel-2 images used in the present study with the sen2cor tool provided by the European Space Agency (ESA) for processing of Sentinel-2 imagery [32]. The two bands used in this study (B5 & B9) were resampled using the sen2cor tool at 20 m resolution.
The following findings are derived from field sampling campaigns, laboratory-based spectral analysis and the development of an empirical spectral model. This exploration encompasses the identification of MP types, quantification of contamination levels, polymer composition analysis, and the implications of plastic pollution management, shedding light on the multifaceted nature of MP pollution in the coastal waters of Dubai.
The laboratory results of the analysis of the coastal water samples showed that all water samples were contaminated with MP particles. However, the contamination varies along different locations and at different beaches. The results have been reported in mg/L and items/L to quantify the extent of MP contamination in the study area. An average of 0.633 and 0.324 mg/L of MPs and 7.85 and 5.30 items/L along the coast of Dubai were found in the two campaigns, respectively. A study in Australia showed that the abundance of MPs in coastal waters is around 2.5 items/L; the higher contamination rate in the study area could be due to the factors discussed earlier [33]. Table 1, Table 2 and Table 3 show the levels of MPs in the samples in mg/L in the two field campaigns. Spectral reflectance analysis in the laboratory of the water samples of the two field campaigns showed absorption peaks in the spectral range 700-875 nm and reflectance peaks in the range 900-1150 nm.
Microplastics contamination in mg/L of the samples of field campaign 1, which was collected on 29 January 2019; results from Jumeirah, Mamzar Park, FS, JBPMTS, and JBR Beaches
Sample Location |
Microplastics concentration by mass cm [mg/L] |
Sample Location |
Microplastics concentration by mass cm [mg/L] |
---|---|---|---|
Jumeirah Beach 3 Location 1 |
0.543 |
FS Beach Left Loccation 1 |
0.604 |
Jumeirah Beach 3 Location 2 |
0.986 |
FS Beach Left Loccation 2 |
0.725 |
Jumeirah Beach 3 Location 3 |
0.866 |
FS Beach Left Loccation 3 |
0.540 |
Jumeirah Beach 3 Location 4 |
0.624 |
FS Beach Left Loccation 4 |
0.604 |
Jumeirah Beach 3 Location 5 |
0.629 |
FS Beach Left Loccation 5 |
0.59 |
Mamzar Park Beach 1 Location 1 |
0.692 |
FS Beach Right Loccation 1 |
0.970 |
Mamzar Park Beach 1 Location 2 |
0.622 |
FS Beach Right Loccation 2 |
0.611 |
Mamzar Park Beach 1 Location 3 |
0.556 |
FS Beach Right Loccation 3 |
0.702 |
Mamzar Park Beach 1 Location 4 |
0.436 |
FS Beach Right Loccation 4 |
0.917 |
Mamzar Park Beach 1 Location 5 |
0.635 |
FS Beach Right Loccation 5 |
0.688 |
Mamzar Park Beach 2 Location 1 |
0.499 |
Jumeirah Beach 2 Location 1 |
0.523 |
Mamzar Park Beach 2 Location 2 |
0.402 |
Jumeirah Beach 2 Location 2 |
0.532 |
Mamzar Park Beach 2 Location 3 |
0.375 |
Jumeirah Beach 2 Location 3 |
0.532 |
Mamzar Park Beach 2 Location 4 |
0.563 |
Jumeirah Beach 2 Location 4 |
0.541 |
Mamzar Park Beach 2 Location 5 |
0.594 |
Jumeirah Beach 2 Location 5 |
0.416 |
Mamzar Park Beach 3 Location 1 |
0.583 |
JBPMTS Location 1 |
0.552 |
Mamzar Park Beach 3 Location 2 |
0.516 |
JBPMTS Location 2 |
0.480 |
Mamzar Park Beach 3 Location 3 |
0.348 |
JBPMTS Location 3 |
0.495 |
Mamzar Park Beach 3 Location 4 |
0.72 |
JBPMTS Location 4 |
0.617 |
Mamzar Park Beach 3 Location 5 |
0.672 |
JBPMTS Location 5 |
0.707 |
Mamzar Park Beach 4 Location 1 |
0.582 |
JBR Beach Location 1 |
0.559 |
Mamzar Park Beach 4 Location 2 |
0.43 |
JBR Beach Location 2 |
0.929 |
Mamzar Park Beach 4 Location 3 |
0.432 |
JBR Beach Location 3 |
0.950 |
Mamzar Park Beach 4 Location 4 |
0.911 |
JBR Beach Location 4 |
0.795 |
Mamzar Park Beach 4 Location 5 |
0.865 |
JBR Beach Location 5 |
0.458 |
Microplastics contamination in mg/L of the samples of field campaign 1, which was collected on 29 January 2019; results from Salt, Kite, Mercato, and Soufouh Beaches
Sample Location |
Microplastics concentration by mass cm [mg/L] |
Sample Location |
Microplastics concentration by mass cm [mg/L] |
---|---|---|---|
Salt Beach Location 1 |
0.584 |
Mercato Right Location 1 |
0.382 |
Salt Beach Location 2 |
0.905 |
Mercato Right Location 2 |
0.475 |
Salt Beach Location 3 |
0.885 |
Mercato Right Location 3 |
0.423 |
Salt Beach Location 4 |
0.325 |
Mercato Right Location 4 |
0.362 |
Salt Beach Location 5 |
0.79 |
Mercato Right Location 5 |
0.682 |
Salt Beach 2 Location 1 |
0.743 |
Mercato Left Location 1 |
0.619 |
Salt Beach 2 Location 2 |
0.477 |
Mercato Left Location 2 |
0.782 |
Salt Beach 2 Location 3 |
0.6.18 |
Mercato Left Location 3 |
0.606 |
Salt Beach 2 Location 4 |
0.707 |
Mercato Left Location 4 |
0.663 |
Salt Beach 2 Location 5 |
0.815 |
Mercato Left Location 5 |
0.727 |
Kite Beach 3 Location 1 |
0.856 |
Soufouh Beach Location 1 |
0.747 |
Kite Beach 3 Location 2 |
0.621 |
Soufouh Beach Location 2 |
0.789 |
Kite Beach 3 Location 3 |
0.541 |
Soufouh Beach Location 3 |
0.699 |
Kite Beach 3 Location 4 |
0.548 |
Soufouh Beach Location 4 |
0.668 |
Kite Beach 3 Location 5 |
0.644 |
Soufouh Beach Location 5 |
0.829 |
Microplastics contamination in mg/L of the samples of field campaign 2, which was collected on 10 Sep 2020
Sample Location |
Microplastics concentration by mass cm [mg/L] |
---|---|
Jumeirah Beach 1 |
0.9 |
Jumeirah Beach 2 |
0.7 |
Kite Beach 1 |
0.20 |
Kite Beach 2 |
0.41 |
Black Palace Beach 1 |
0.15 |
Black Palace Beach 2 |
0.5 |
JBR beach 1 |
0.1 |
JBR beach 2 |
0.13 |
Mercato Beach 1 |
0.06 |
Mercato Beach 2 |
0.09 |
Polymer composition of the subsample was performed using Fourier Transform Infrared (FT-IR) analysis. Analysis showed that different beaches have different types of MPs present. In Jumeirah Beach, PE and PP were present; in Kite Beach, PE and PP were present; Black Palace Beach was mostly contaminated with PP, PE, and PVC, whereas in JBR Beach, PE, PET, and PS were present. As for the Mercato Beach, PS, PET, PP, and PE were present. The densities of the MPs verify the types identified in the samples that correspond to each beach. PE and PP have lower densities (PE = 0.93 g/cm3, PP = 0.83 g/cm3), which is true for Mercato Beach and JBR Beach, which shows low contamination in mg/L as low-density MPs have low weight compared to higher-density MPs. As for the remaining beaches, higher-density MPs are present, reflected in the higher contamination in mg/L as the PVC and PS have higher densities (PVC = 1.16 g/cm3, PS = 1.04 g/cm3). The main source of this contamination could be coastal tourism since all these beaches receive thousands of people every day all year long. Also, all these beaches are close to fisheries and harbours, which is a possible source of these MPs.
Spectral analysis of various MP samples was conducted, taking into account their size, type, and total suspended solids (TSS). In the wavelength range of 700-850 nm, there is an evident relative increase in spectral reflectance with the increase in the concentration of the TSS; however, there is no clear difference in reflectance with the increase in TSS in the mixes beyond 850 nm as the reflectance peaks to about 50%.
The results indicate that varying the size and type of MPs and adding sand in varying amounts did not significantly alter the resulting spectral reflectance. Specifically, it was observed that adding sand did not affect the peak wavelength. The highest spectral reflectance is within the 1000-1200 nm wavelength range for all readings. It indicates that the spectrometer could detect the MPs easily at this wavelength range, which is not affected by the presence of suspended material (Appendix Figures A1, A2, A3, and A4). Figure 4 shows the reference spectral reflectance curves for the considered types of MPs and the specified sand mixes.
The laboratory-based (reference) spectral reflectance curve for the considered types of microplastics and the specified sand mixes
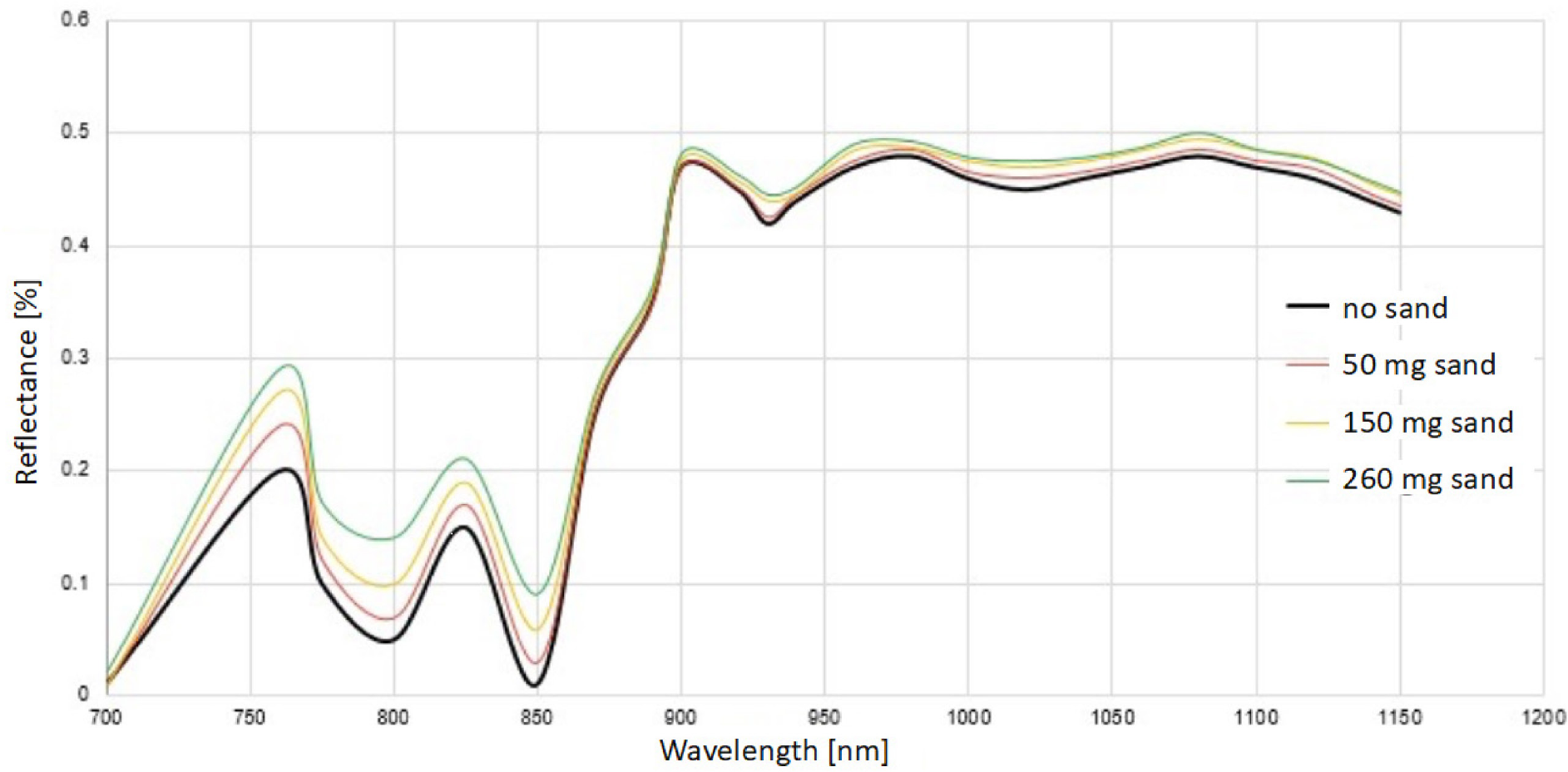
The sizes of MPs did not affect the outcomes, as the highest spectral reflectance remains within the identified wavelength range for all types and sizes of MPs. The results suggest that the MPs can be detected in the wavelength range 900-1200 nm (Appendix Figures A10, A11, and A12).
The spectral reflectance of the different types of plastic materials is measured, keeping the size and sand amounts constant. The different types of MPs are detected at a wavelength range of 900-1200 nm, which was identified as the spectral signature of these MPs in the study area. This spectral signature was used to select band B9 of the Sentinel-2 satellite to determine MP concentrations in the coastal water of Dubai (Appendix Figures A5, A6, A7, A8, and A9).
The summary of the laboratory results is presented in Table 4. PET showed a high reflectance in the range 900-1100 nm, PS in 900-1100 nm, PVC in 900-1150 nm, PP in 900-1100 nm and PE in 900-1100 nm. Accordingly, it was determined that the different types of MPs used in the laboratory experiments exhibited the highest reflectance in the wavelength range of 900-1150 nm. On the other hand, the three types have shown the highest absorption in the wavelength range of 700-850 nm. Different types of MPs were detected at the wavelength range 900-1150 nm, identified as the spectral signature of these MPs in the study area. The highest absorption at 700, 800, and 850 nm, and reflectance peaks at around 900, 985, and 1080 nm can be mapped to Sentinel-2 bands B5 (central wavelength: 705 nm) and B9 (central wavelength: 945 nm). Based on this, the highest absorption can be identified in band B5, while the highest reflectance can be detected in band B9. This spectral signature was used to select bands B5 and B9 of the Sentinel-2 satellite to develop spectral models for determining the concentrations of MPs in the study area.
Wavelength sensitivity of microplastics
Type of microplastics |
Wavelength range [nm] |
Similar Characteristics |
Sentinel-2 Satellite |
---|---|---|---|
Polyethylene terephthalate |
700−1100 |
Highest absorption at 700, 800, and 850 nm, and reflectance peaks at around 900, 985, and 1080 nm |
Bands B5−B9 |
Polypropylene |
800−1150 |
||
Polystyrene |
700−1100 |
||
Polyvinyl chloride |
800−1150 |
||
Polyethylene |
700−1000 |
Regression analysis was used to obtain the best-fit model correlating the sampled MP concentrations using the field data and the spectral reflectance of Sentinel-2 image bands B5 and B9 on 29 January 2019 at the sampling locations. Regression analysis was used to establish the correlation between the measured MP concentrations (N=90) and the spectral reflectance at bands B5, B9, B5×B9, and B5/B9. Sixty-five (N=65) data values from field campaign 1 were used to develop the empirical spectral model, and fifteen (N=15) values were used for model calibration. Table 5 shows the investigated Sentinel-2 band combinations and their corresponding R2 values.
The investigated Sentinel-2 band combinations and the corresponding R2 values
Wavelength sensitive to MPs in the study area |
Sentinel-2 bands combination |
R2 value [%] |
---|---|---|
⍴705 |
B5 |
25.67 |
⍴945 |
B9 |
54.20 |
⍴705×⍴945 |
B5×B9 |
33.80 |
⍴705/⍴945 |
B5/B9 |
87.30 |
The best-fit spectral MPs model can convert atmospherically corrected Sentinel-2's reflectance at bands B5 (705 nm) and B9 (945 nm) into MPs (mg/L) with an R2 value of 87.30% and RMSE of ±0.015 mg/L. The resulting empirical model is shown in eq. (1) and Figure 5.
(1)
where symbol MPs denotes the concentration of particles by mass in the coastal water in mg/L, and ⍴705 and ⍴945 are the central wavelengths of bands B5 and B9 of the Sentinel-2 sensor.
The best-fit spectral MPs model, which combines Sentinel-2's bands B5 and B9
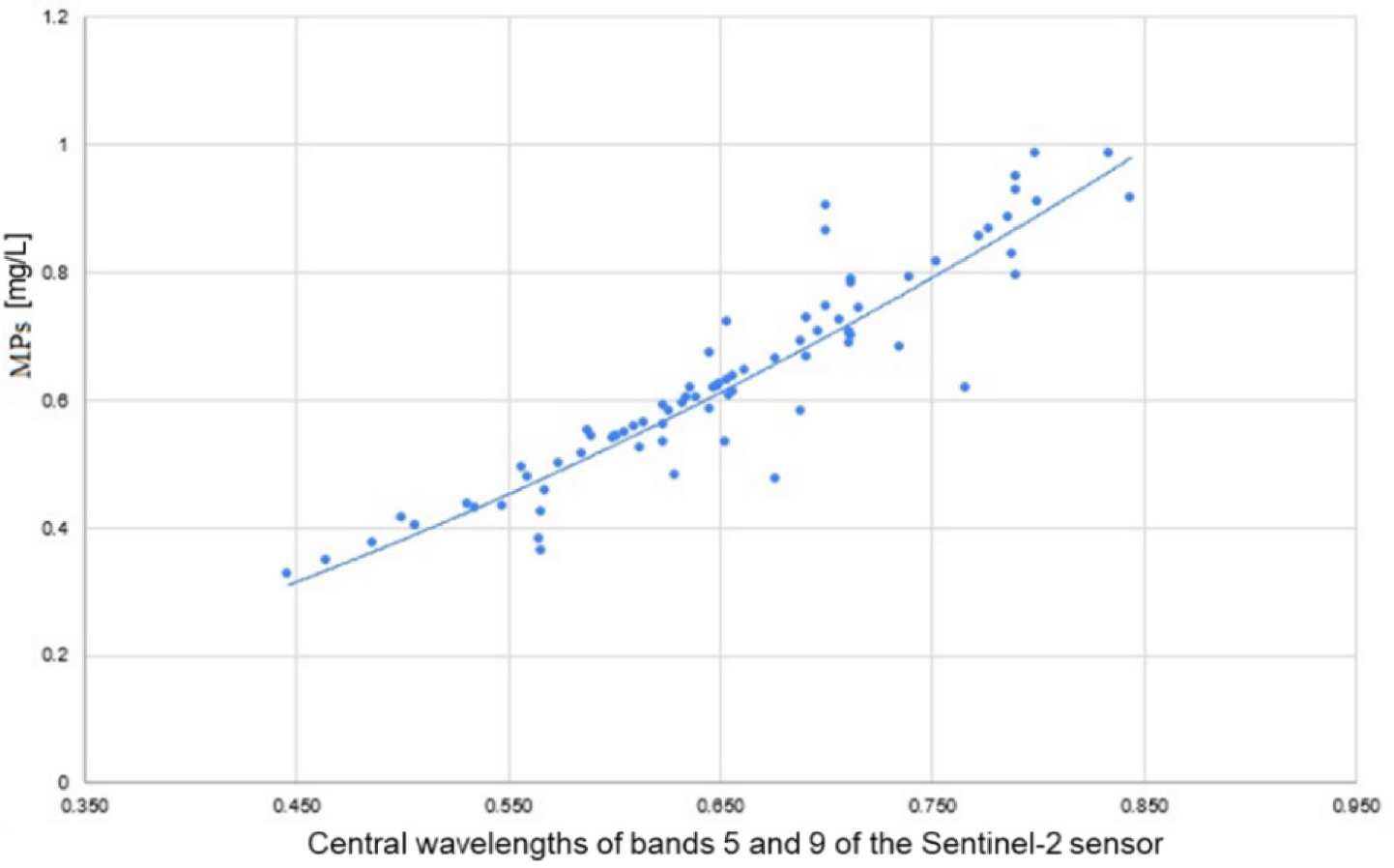
The resulting empirical spectral model in eq. (1) was then applied to the Sentinel-2 image on 29 January 2019 (bands B5 and B9) to obtain the levels of MPs in the water on the coast of Dubai, as shown in Figure 6.
The spatial distribution of MPs estimated with the model on 29 January 2019; the bars show the sampled and modelled MPs levels at the sampling locations
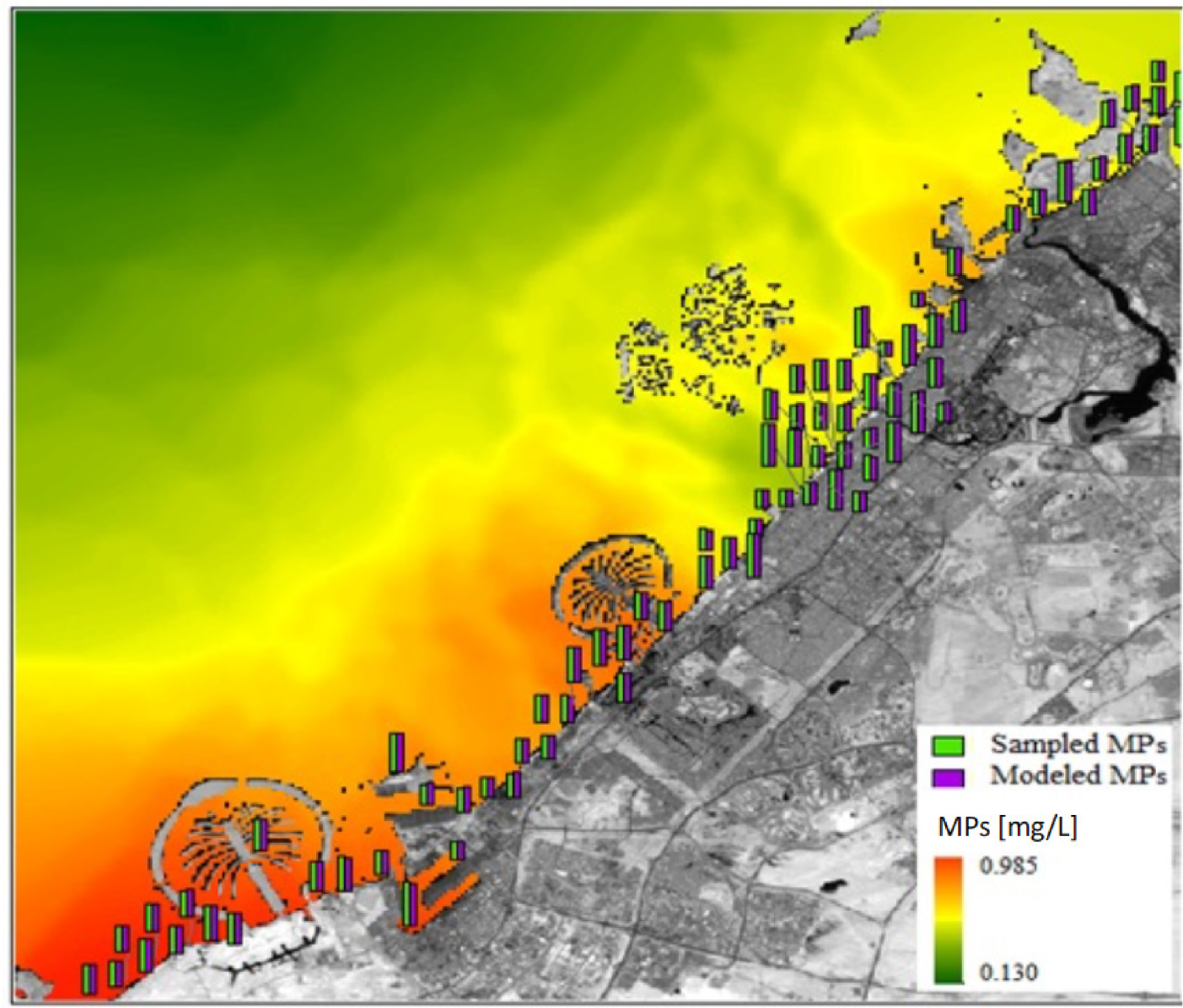
The figure shows that the concentrations of MPs are higher in the nearshore zone and inland water bodies than in offshore and open gulf water areas. This spatial distribution pattern strongly suggests that human activities in coastal regions play a significant role in the elevated presence of MPs. These findings are consistent with previous studies highlighting the impact of urbanization, industrial activities, and improper waste management practices on coastal MP pollution [34]. This type of pollution monitoring model can be useful to identify the specific source of MPs and the potential management plan of the plastic disposal system.
Identifying hotspots with higher MP concentrations provides crucial information for targeted intervention and mitigation strategies. Coastal management authorities can utilize this information to prioritize and implement measures to reduce the input of MPs into nearshore waters. For instance, implementing stricter regulations on waste disposal and effective waste management practices can help minimize the release of plastic debris into water bodies [35]. Additionally, raising public awareness about the impact of plastic pollution and promoting responsible consumption and recycling habits can reduce the overall load of MPs entering coastal environments [36].
To demonstrate the usefulness and viability, the resulting empirical model was used to obtain the spatial distributions of MPs in the area for September 2018, 2019, 2020, and 2021 (September marks the beginning of the autumn season in the UAE). The spatial distributions of MPs generated with the model in 2018-2021 are shown in Figure 7.
The spatial distribution of MPs estimated with the spectral model on 11 Sep 2018 (a), 11 Sep 2019 (b), 10 Sep 2020 (c), and 10 Sep 2021 (d)
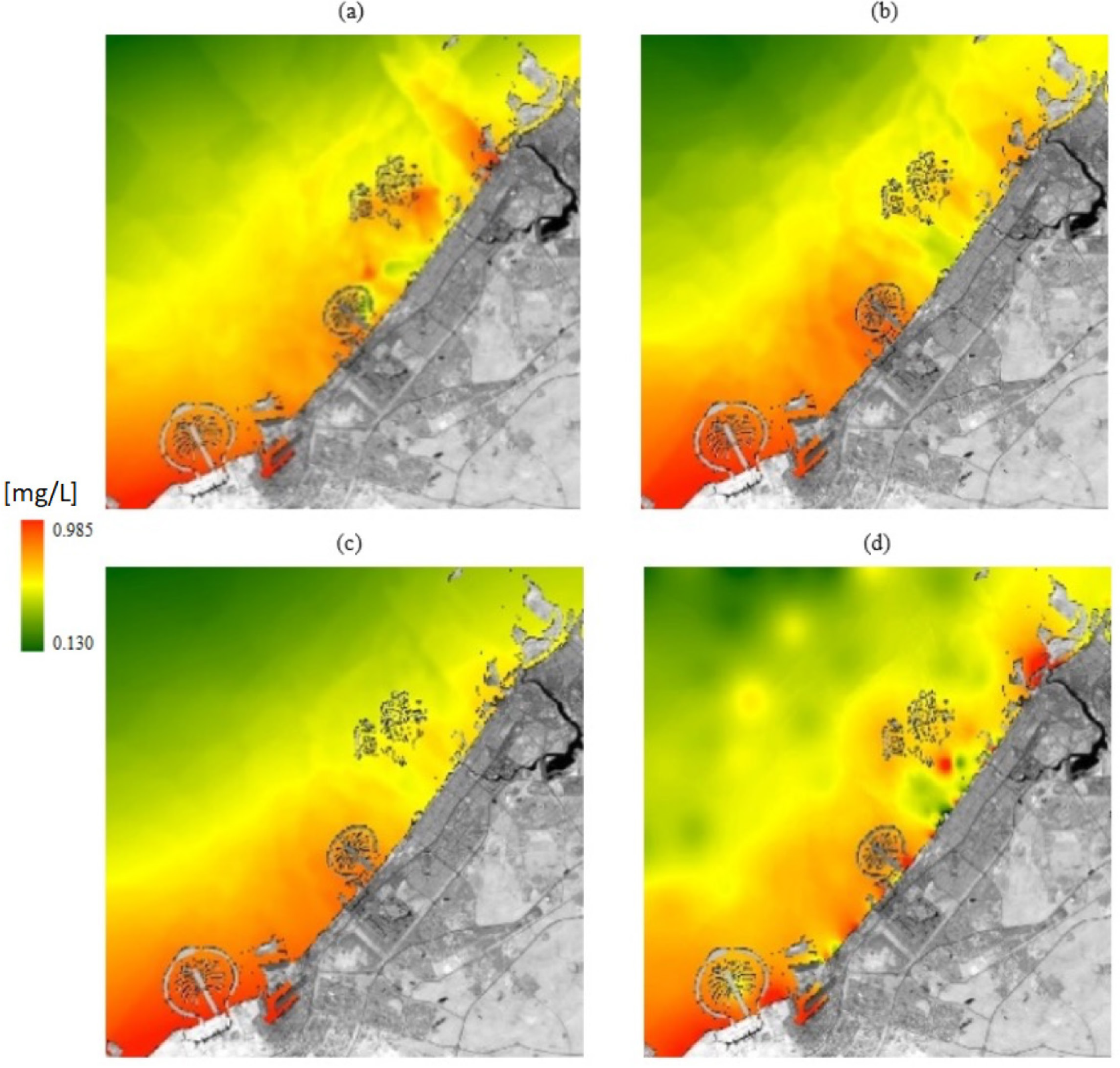
The MP distributions show high concentrations on the west coast of the Emirate, consistent with the locations of many busy beaches in this part of Dubai and the location of the Port of Jebel Ali, the busiest port in the region. This part of the Arabian Gulf is characterized by a 1-16 m deep bathymetry, west-to-east surface currents, and circulation processes with a seasonal averaged velocity field of about 0.2 m/s [37], which can also explain the resulting spatial patterns of MPs generated by the model. MP concentrations appear lower in 2020 and 2021 than in previous years due to the lack of coastal activities (i.e., beaches) during the pandemic, which decreased the MP concentrations.
While remote sensing offers promising potential for detecting MPs, several current limitations must be considered. The present study considers six different polymer types: PE, PP, PVC, PS, PUR, and PET, and it may not capture the full spectrum of polymer type variations. Furthermore, MPs come in various shapes, sizes, colours, and compositions, which can result in diverse spectral signatures and should be considered for future studies. Regarding the choice of the size range for inclusion in the present study - visible MPs (1-5.0 mm), the decision was influenced by the method validation process, which involved spectrophotometric measurements of absorbance of the samples prepared in the laboratory. The method's validation primarily focused on larger MPs, and in preparing these particles, the macro plastics were cut into smaller pieces. Physical difficulties were associated with cutting within a smaller MP range (<1 mm) that might be considered in future studies.
Furthermore, the spatial resolution of remote sensing imagery may not be sufficient to accurately detect and distinguish very small MPs. In this case, higher-resolution imagery is needed. There is a lack of standardized protocols and calibration procedures for MP remote sensing. Ensuring consistency in calibration methods and standardization across different remote sensing platforms is crucial for the accurate and reliable detection of MPs. Remote sensing techniques may encounter challenges in distinguishing MPs from other natural and anthropogenic materials present in the environment. Validation through ground truthing and cross-referencing with in situ sampling is necessary to verify and validate remote sensing results.
By addressing these limitations and conducting further research along these suggested lines, a better understanding of MP pollution in the coastal waters of Dubai and the development of more effective strategies for its management and mitigation can be performed.
This study aimed to evaluate MP contamination along the Dubai coast using remote sensing techniques. Water samples with synthetic MPs mixed with sand were created and analyzed to identify the spectral reflectance of MPs. The results showed that the MPs and the sand mixes exhibit high absorption in the wavelength range 700-850 nm and relatively high reflectance in the range 900-1150 nm, which were matched with Sentinel-2's bands B5 and B9, respectively. Two field campaigns were carried out for sampling MPs using grab samples along the Dubai coastline where the waves break, campaign 1 on 29 January 2019 and campaign 2 on 10 September 2020. Two samples were collected from each location to eliminate bias and have a representative sample of the sampling location. These samples were taken to the laboratory and analyzed using the NOAA method to manually extract the MPs. A 40 X magnification microscope was used to identify the number of MPs in each sample, followed by weighing MPs in each sample. Samples analysis showed an average of 0.633 and 0.324 mg/L of MPs and 7.85 and 5.30 items/L along the coast of Dubai in field campaigns 1 and 2, respectively.
An empirical spectral model of MPs was developed based on the reflectance values at bands B5 and B9. Sixty-five (N=65) data values from field campaign 1 were used to develop the empirical spectral model, and fifteen (N=15) values were used for model calibration. The model was further validated using field campaign 2 data. The R2 value for the best-fit model was computed as 87.30%, and the RMSE was found to have a value of ± 0.015 mg/L. The results suggest that bands B5 and B9 of Sentinel-2 imagery can be used in the model to obtain the levels of MPs in the coastal water column of the study area and similar areas around the world. The model was successfully used to obtain the annual spatial distributions of MPs in the study area in 2018, 2019, 2020, and 2021, respectively. The resulting distributions are consistent with the locations of the busiest beaches and the Port of Jebel Ali and the physical properties of this part of the Arabian Gulf, characterized by west-to-east circulation forces and with a relatively slow velocity field. The outcome of this study can potentially facilitate cost-effective and timely monitoring of MP pollution in coastal water, which is essential in environmental and management processes. It may help eliminate some of the challenges of monitoring MP pollution on large coastlines and understand the effect of different natural and anthropogenic pollution events.
The authors would like to thank Mr. Riyad Temam for his help and support in conducting the experiments.
cm |
Concentration by mass |
[mg/L] |
MPs |
Concentration of microplastic particles by mass |
[mg/L] |
ρ |
Wavelength |
[nm] |
Abbreviations |
||
MPs |
Microplastics |
|
NOAA |
National Oceanic and Atmospheric Administration |
|
UV |
Ultraviolet |
|
PE |
Polyethylene |
|
PP |
Polypropylene |
|
PVC |
Polyvinyl Chloride |
|
PS |
Polystyrene |
|
PUR |
Polyurethane |
|
PET |
Polyethylene Terephthalate |
|
FT-IR |
Fourier Transform Infrared |
|
ESA |
European Space Agency |
|
TSS |
Total Suspended Solids |
|
WPO |
Wet Peroxide Solution |
|
UAE |
United Arab Emirates |
Biodegradability of plastics ,International Journal of Molecular Sciences , Vol. 10 (9), 2009, https://doi.org/https://doi.org/10.3390/ijms10093722
, Catalytic pyrolysis of mechanically non-recyclable waste plastics mixture: Kinetics and pyrolysis in laboratory-scale reactor ,J Environ Manage , Vol. 296 , 2021, https://doi.org/https://doi.org/10.1016/j.jenvman.2021.113145
, Microplastics: Finding a consensus on the definition ,Mar Pollut Bull , Vol. 138 , 2019, https://doi.org/https://doi.org/10.1016/j.marpolbul.2018.11.022.
, Microplastic pollution in lakes and lake shoreline sediments - A case study on Lake Bolsena and Lake Chiusi (central Italy) ,Environmental Pollution , Vol. 213 , 2016, https://doi.org/https://doi.org/10.1016/j.envpol.2016.03.012
, Leachability of microplastic from different plastic materials ,J Environ Manage , Vol. 294 , 2021, https://doi.org/https://doi.org/10.1016/j.jenvman.2021.112995
, Sources of microplastics pollution in the marine environment: Importance of wastewater treatment plant and coastal landfill ,Mar Pollut Bull , Vol. 146 ,pp 608–618 , 2019, https://doi.org/https://doi.org/10.1016/J.MARPOLBUL.2019.06.066
, Municipal solid waste (MSW)landfill: A source of microplastics? -Evidence of microplastics in landfill leachate ,Water Res , Vol. 159 , 2019, https://doi.org/https://doi.org/10.1016/j.watres.2019.04.060
, Linking effects of microplastics to ecological impacts in marine environments ,Chemosphere , Vol. 264 , 2021, https://doi.org/https://doi.org/10.1016/j.chemosphere.2020.128541
, Transport and characterization of microplastics in inland waterways ,Journal of Water Process Engineering , Vol. 38 , 2020, https://doi.org/https://doi.org/10.1016/j.jwpe.2020.101640
, Evaluation of microplastics in beach sediments along the coast of Dubai, UAE ,Mar Pollut Bull , Vol. 150 , 2020, https://doi.org/https://doi.org/10.1016/j.marpolbul.2019.110739
, Consistent Transport of Terrestrial Microplastics to the Ocean through Atmosphere ,Environ Sci Technol , Vol. 53 (18), 2019, https://doi.org/https://doi.org/10.1021/acs.est.9b03427
, A simple model of wind-blown tidal strandlines: How marine litter is deposited on a mid-latitude, macro-tidal shelf sea beach ,Mar Pollut Bull , Vol. 137 , 2018, https://doi.org/https://doi.org/10.1016/j.marpolbul.2018.10.024
, Plastics and microplastics: A threat to environment ,Environmental Technology and Innovation , Vol. 14 , 2019, https://doi.org/https://doi.org/10.1016/j.eti.2019.100352
, Marine litter as habitat and dispersal vector ,Marine Anthropogenic Litter , 2015, https://doi.org/https://doi.org/10.1007/978-3-319-16510-3_6
, Transportation fate and removal of microplastic pollution – A perspective on environmental pollution ,Case Studies in Chemical and Environmental Engineering , Vol. 2 , 2020, https://doi.org/https://doi.org/10.1016/j.cscee.2020.100015
, Organic contaminants sorbed to microplastics affect marine medaka fish early life stages development ,Mar Pollut Bull , Vol. 154 , 2020, https://doi.org/https://doi.org/10.1016/j.marpolbul.2020.111059
, Microplastics as a threat to coral reef environments: Detection of phthalate esters in neuston and scleractinian corals from the Faafu Atoll, Maldives ,Mar Pollut Bull , Vol. 142 , 2019, https://doi.org/https://doi.org/10.1016/j.marpolbul.2019.03.043
, Microplastics in ballast water as an emerging source and vector for harmful chemicals, antibiotics, metals, bacterial pathogens and HAB species: A potential risk to the marine environment and human health ,Marine Pollution Bulletin , Vol. 149 , 2019, https://doi.org/https://doi.org/10.1016/j.marpolbul.2019.110525
, An airborne remote sensing case study of synthetic hydrocarbon detection using short wave infrared absorption features identified from marine-harvested macro- and microplastics ,Remote Sens Environ , Vol. 205 ,pp 224–235 , 2018, https://doi.org/https://doi.org/10.1016/j.rse.2017.11.023
, Laboratory methods for the analysis of microplastics in themarine environment: Recommendations for quantifying synthetic particles in waters and sediments. NOAA Technical Memorandum NOS-OR&R-48. ,NOAA Technical Memorandum , 2015
, Comparison of microplastic pollution in different water bodies from urban creeks to coastal waters ,Environmental Pollution , Vol. 246 , 2019, https://doi.org/https://doi.org/10.1016/j.envpol.2018.11.081
, Using a forensic science approach to minimize environmental contamination and to identify microfibres in marine sediments ,Mar Pollut Bull , Vol. 95 (1), 2015, https://doi.org/https://doi.org/10.1016/j.marpolbul.2015.04.044
, Wind-Driven Dynamics of Beach-Cast Wrack in a Tide-Free System ,Open Journal of Marine Science , Vol. 04 (02), 2014, https://doi.org/https://doi.org/10.4236/ojms.2014.42009
, Coastal accumulation of microplastic particles emitted from the Po River, Northern Italy: Comparing remote sensing and hydrodynamic modelling with in situ sample collections ,Mar Pollut Bull , Vol. 138 , 2019, https://doi.org/https://doi.org/10.1016/j.marpolbul.2018.11.045
, Life in the ‘plastisphere’: Microbial communities on plastic marine debris ,Environ Sci Technol , Vol. 47 (13), 2013, https://doi.org/https://doi.org/10.1021/es401288x
, Ups and Downs in the Ocean: Effects of Biofouling on Vertical Transport of Microplastics ,Environ Sci Technol , Vol. 51 (14), 2017, https://doi.org/https://doi.org/10.1021/acs.est.6b04702
, The plastic in microplastics: A review ,Marine Pollution Bulletin , Vol. 119 (1), 2017, https://doi.org/https://doi.org/10.1016/j.marpolbul.2017.01.082
, PET and PVC separation with hyperspectral imagery ,Sensors (Switzerland) , Vol. 15 (1),pp 2205–2227 , 2015, https://doi.org/https://doi.org/10.3390/s150102205
, Persistence of plastic litter in the oceans ,Marine Anthropogenic Litter ,pp 57-72 , 2015, https://doi.org/https://doi.org/10.1007/978-3-319-16510-3_3
, The variation of total suspended sediments due to the change in sea water depth, tidal phase and elevation of sea water sample collection in khor sabiya inlet of the arabian Gulf, Kuwait ,Journal of Coastal Research , 2011, https://doi.org/https://doi.org/10.2112/SI61-001.41
, Dangerous hitchhikers? Evidence for potentially pathogenic Vibrio spp. on microplastic particles ,Mar Environ Res , Vol. 120 , 2016, https://doi.org/https://doi.org/10.1016/j.marenvres.2016.07.004
, A new analytical approach for monitoring microplastics in marine sediments ,Environmental Pollution , Vol. 184 , 2014, https://doi.org/https://doi.org/10.1016/j.envpol.2013.07.027
, New techniques for the detection of microplastics in sediments and field collected organisms ,Mar Pollut Bull , Vol. 70 (1–2), 2013, https://doi.org/https://doi.org/10.1016/j.marpolbul.2013.03.009
, Microplastics as contaminants in the marine environment: A review ,Marine Pollution Bulletin , Vol. 62 (12), 2011, https://doi.org/https://doi.org/10.1016/j.marpolbul.2011.09.025
, Occurrence and distribution of microplastics in marine sediments along the Belgian coast ,Mar Pollut Bull , Vol. 62 (10), 2011, https://doi.org/https://doi.org/10.1016/j.marpolbul.2011.06.030
, High Quantities of Microplastic in Arctic Deep-Sea Sediments from the HAUSGARTEN Observatory ,Environ Sci Technol , Vol. 51 (19), 2017, https://doi.org/https://doi.org/10.1021/acs.est.7b03331
, Response to the Letter to the Editor Regarding Our Feature ‘are We Speaking the Same Language? Recommendations for a Definition and Categorization Framework for Plastic Debris ,Environmental Science and Technology , Vol. 53 (9), 2019, https://doi.org/https://doi.org/10.1021/acs.est.9b02238
,