Access to clean and safe drinking water is one of the world’s most daunting challenges [1] requiring urgent attention [2]. An estimated 785 million people in the world still lack access to a safe, clean and basic drinking water supply [2], [3]. Regrettably, contaminated drinking water poses a major health threat to human beings as it contains pathogens, which cause diseases like cholera, typhoid fever, and diarrhoea [4] and kills around 1.5 million people every year [5]. The problem is prevalent in developing countries and arid areas where safe water sources are scarce and people resort to using contaminated surface water sources [6]. The United Nations Development Programme (UNDP) reports that 71% of the global population, about 5.2 billion people, had safely-managed drinking water in 2015, while 844 million people still lacked even basic drinking water [7]. In the year 2017, it was reported that 206 million people used limited services, 435 million used unimproved sources, while 144 million still used surface water [3].
The Sustainable Development Goal (SDG) 6, therefore, advocates for the achievement of universal and equitable access to safe and affordable drinking water for all by 2030 [7]. Regionally, the Southern African Development Community (SADC) Water Policy (2005), among others, promotes equitable access to water of an acceptable quantity and quality in its water vision statements [8]. Furthermore, the Malawi National Water Policy (2005) aims at achieving the sustainable provision of water supply and sanitation services that are equitably accessible and used by individuals and entrepreneurs for socio-economic development at an affordable cost [9]. The promotion of cost-effective water disinfection methods is, therefore, an urgent need in developing countries to improve access to clean and safe water, especially for the low and medium-income households, which are most venerable to the outbreaks of waterborne diseases [10]. Such methods include Solar Water Disinfection (SODIS) [11] and the use of chlorine for water treatment [12]. The SODIS system has proved to be an inexpensive and effective way to treat water in many communities [13].
The SODIS is a simple, environmentally sustainable, low-cost method for treating drinking water at the household level for people that use microbiologically contaminated raw water as the only water available for all household chores including drinking [14]. Previous studies have shown that SODIS is an appropriate technology for water treatment in low and medium-income households [15] owing to its effectiveness against a wide range of waterborne pathogens, low-cost or zero-cost of technology in areas where transparent containers, typically Polyethylene Terephthalate (PET) bottles, are available [16]. Additional benefits are ease of use as it can be employed by any user with very little training, and its appropriateness in using available sunlight to reduce the microbial load of water without using any chemical additives, high technology or electrical supply [14], [17].
The method uses solar energy to kill pathogenic microorganisms that cause water-borne diseases [18], thereby improving the quality of drinking water [19]. The process involves filling a transparent bottle with untreated water and exposing them to sunlight energy for at least 6 hours [20]. It is mostly used in developing and disaster-stricken countries to improve access to safe water. The process is successfully applied in areas between latitudes 35°N and 35°S [11]. These areas receive high levels of solar radiation ranging from 800 to 1200 W/m2 during most of the year, which has the potential of raising water temperature to kill water-borne disease pathogens if captured using appropriate materials for the construction of the SODIS technology [11].
The SODIS system uses the synergy of the infrared and ultraviolet spectra of solar radiation (wavelength above 1000 nm and wavelength between 4 and 400 nm respectively) to increase bottle water temperature and inactivate microorganisms [4]. The disinfecting mechanisms employed are thermal inactivation achieved by raising bottle water temperatures above the microorganism metabolism temperatures [15]; photo-oxidative disinfection through the formation of highly reactive oxygen, oxygen-free radicals and hydrogen peroxide from the exposure of well-oxygenated water to sunlight [21]. The formed reactive species oxidize and damage the cells of a living organism [22]. Another mechanism is Deoxyribonucleic Acid (DNA) alterations, which are induced by the direct effect of ultraviolet rays of the sun. They occur when high-energy photons released by the sun are absorbed by microorganisms’ photosensitizers, which react with oxygen and the organism’s DNA to cause strand breakage, mutagenic effect and base changes, which are fatal to the organism [1]. These mechanisms together achieve a process known as solar disinfection.
Despite the proven efficacy of chlorination of water, the cost of chlorine, limited financial resources by households and unreliability of the chlorine supply chain in rural areas are major contributors to poor coverage and low adoption of the chlorination method in the rural areas of developing countries [10], [23] Households have also used boiling as a means of disinfecting contaminated water for drinking. However, the imparting of a flat taste and scarcity of firewood hinders the adoption of boiling as a water treatment method [14]. According to Acra [24], approximately one kilogram of wood is needed to boil one litre of water. Consequently, households prioritise sourcing firewood for cooking rather than boiling water only [25]. Although many developing countries produced various policies and strategies to foster the provision of water and sanitation services, especially in rural areas, the adoption rate of household water treatment and safe storage (HWTS) and point-of-use treatment are reported to be low [14]. Previous studies and reports on HWTS interventions showed that they are expensive, not easy to use and unsustainable [11]. For example, water treatment techniques like filtration, boiling and use of chlorine are perceived to be expensive in developed countries [13]. There is a need for the development of a cost-effective functioning model to address these challenges and diversify the water treatment and point-of-use treatment options already available in rural areas.
This study, therefore, was aimed at designing and developing an enhanced prototype of a solar disinfection system for improved rural household water treatment. Specifically, the study was framed to design and construct a prototype of a SODIS for improved rural household water treatment. The effectiveness of the prototype in killing microorganisms was evaluated through thermal analysis and measuring the microbiological water quality parameters of both untreated and treated water. The research was approved by the Mzuzu University (Malawi), Research Committee with guidance from the Directorate of Research at the University. All the ethical considerations and research protocol were followed during the implementation of the study. Experiments and laboratory analyses were performed by the research team with guidance and support from experts at Mzuzu University.
In this section, we present and summarise the design and prototype of the disinfection system, its construction, thermal analysis, and system efficiency as well as the testing and economic feasibility of the disinfection system. The section also outlines the quality control, data management, and statistical analysis used in the study
Since the discovery of SODIS, several research and clinical trials have been undertaken by different researchers on its improvement and effectiveness such as Ismail et al. [26] and Swiss Federal Institutes of Science and Technology (EAWAG) Department of Water and Sanitation Developing Countries (SANDEC) [27]. For instance, the effectiveness of using small and large plastic containers, plastic bags, and PET bottles has been tested for purifying contaminated water [28] to meet drinking water requirements in low- and medium-income households [15]. Our design and prototype were based on three main SODIS concepts reported in literature namely the use of simple sunlight bottle exposure, [1] solar reflector incorporated SODIS system [24], and the Dual SODIS and solar still water purifier [26]. [Table 1] shows a matrix evaluation of the three concepts used as a basis in designing this enhanced SODIS prototype. Comparative analysis of the three concepts showed SODIS concept in the dual SODIS and solar was potentially the most effective and would perform better in rural areas of Malawi.
Matrix evaluation of concepts in relation to the study design**
Concept criteria | 1st Concept | 2nd Concept | 3rd Concept |
---|---|---|---|
Mode | Simple sunlight bottle exposure | Solar reflector incorporated SODIS | Dual SODIS and solar still water purifier |
Quantity of water treated per cycle | - | - | + |
Heat enhancements | - | + | + |
Ease of maintenance | + | + | - |
Time of exposure required per cycle | - | - | + |
Geographical area of operation | - | - | - |
Mode (basis) of disinfection | kills microorganism by the synergetic effect of solar Ultraviolet-A radiation and thermal heating | bottles in system half painted (in black) to enhance heating capacity and a solar reflector introduced to increase the amount of radiation incident | combined systems: solar IR & heat energy (no distillation) plus solar heat energy to drive evaporation, and ambient air to cool condenser films (distillation occurs). |
- relatively lower performance. + relatively higher performance. Source**” EAWAG and SANDEC [27]
The focus of the design was on improving the SODIS element in the dual water purification system, by incorporating features of the other two concepts to enhance thermal capacity. Furthermore, the third concept was chosen due to the ease of incorporating features of the other concepts for improved performance and its relative advantages over the other two concepts ([Table 1]).
[Figure 1] shows an improved SODIS system developed in this study with four main components, namely solar collector (A), wooden support structure and windshields (B) the transparent plastic sheet, and the heat storage chamber (C). The system was constructed using locally sourced materials including an iron sheet, 2L PET bottles, timber, plywood boards, soft nail wires, black paint, and clear transparent plastic paper. The PET bottles were selected for this system owing to the good transmitting property of light in the ultraviolet A (UV-A) and visible range spectrum, low-cost, durability, reusable and are locally available.
The SODIS prototype construction showing main components
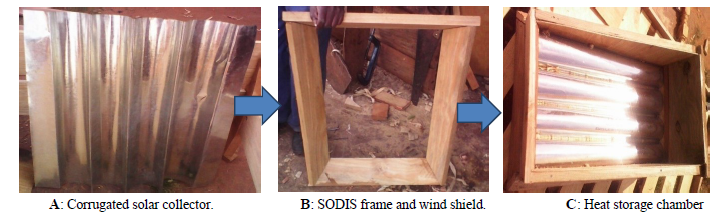
The SODIS prototype set-up (prototype) drawn using AutoCAD
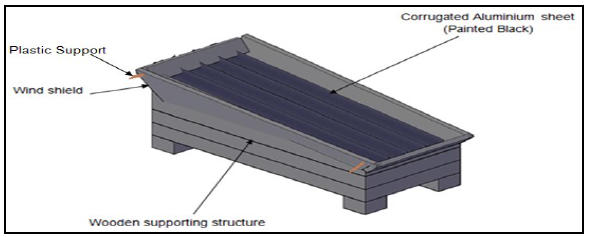
[Figure 2] shows the schematic view of the main parts of the SODIS system being constructed and assembled. The solar collector is an aluminium metal sheet shaped into a big parabolic corrugation to allow focus sunlight rays into the water bottles placed in the troughs. The corrugation and the bottles used in the system were half painted black to enhance heat absorption and hence the heating capacity of the system. The windshields were incorporated to reduce heat losses induced by the wind, while the transparent plastic sheet was used to trap the heat generated in the system. The wooden support structure also included a heat storage chamber, which was located underneath the solar collector. The heat storage chamber was incorporated to store heat used to buffer the effects of cloud cover and to minimize heat losses from the system due to changes in atmospheric conditions. The top side of the system was covered by transparent plastic paper to allow sunshine penetration and prevent debris from entering the top chamber. The prototype of the SODIS system was fabricated by a local carpentry artisan in Mzuzu City in Malawi with supervision by the researchers. The size of the collectors’ corrugation and the entire SODIS system were estimated and set using eq. (1):
(1)
where: T is the trough size, r is the radius of the 2L PET bottles used in the SODIS system and π is a constant taken as 22/7. Considering the measured radius of the PET bottles (r=0.095 m), the area of the solar collector was therefore found to be 0.495 m2.
The optimum angle of tilt or inclination (θ) of the SODIS system was set using eq. (2):
(2)
where β is the latitude of the area, Z is any integer between -10 and +10. Since Malawi lies between latitudes 9°22’S and 17°03’S and longitudes 33°40’E and 35°55’E [29], the 9° was taken as the design latitude parameter β, while +6 was chosen as the integer (Z) to be used and the optimum angle of tilt/inclination (θ) was therefore set at 15°.
The efficacy of the SODIS system is dependent on several variables including time of exposure, [1] solar intensity, the surface area of the collector [24], and the amount of water to be disinfected in the bottles [26]. Thermal analyses were performed by considering several parameters such as temperature measurements and heat energy exchange. The inside system temperatures and those in the ambient were determined by digital thermocouples and recorded the readings every 20 minutes. The temperature of water in the PET bottles was measured using a mercury thermometer. The heat energy that entered the system through the plastic into the water (Qf) was calculated from eq. (3) [30]:
(3)
where: m is the mass of water placed in the bottles, c is the specific heat capacity of water (4.187 kJ/kgoC), and ΔT is the change in water temperature given as , in which (ti) and (tf) are initial and final temperature readings, respectively.
The heat transfer rate (Qf rate) was thus estimated by using eq. (4):
(4)
where: Qf is the amount of heat that enters the plastic bottle and absorbed by the water as expressed in Equation 3, and t is the time for obtaining the heat increase.
The amount of heat energy received by the system is dependent on the latitude of an area, which affects the solar constant [26]. This parameter was determined using eq. (5):
(5)
where: Qy is the amount of heat energy received, I is the solar constant, and A is the collector surface area.
The amount of radiation from the sun lost (Qi) in SODIS system depends on properties of the material (in this case the transparent plastic paper) namely transmissivity (τ) and absorptivity (α) product is given by eq. (6) as used by Robaa [31]:
(6)
where: τ is the percentage of solar radiation passing through the plastic paper (also called transmissivity), and α is the percentage of solar radiation absorbed by the collector.
Regardless of the property of the material used there are always heat gains and losses. The heat gained or lost by the water was determined using the heat gain or loss coefficient (UL) as expressed in eq. (7) [32]:
(7)
where: Qi is the amount of radiation received, A is the collector surface area, and ΔT is the change in water temperature.
The efficiency η of the SODIS system was estimated using eq. (8) as used in Kandpur and Sudhir [33]:
(8)
where: ΔT is the change in water temperature observed in a short time interval t, A is the surface area of the collector, c is the specific heat capacity of the water, I is the solar irradiance constant of the particular area, and t is a time interval the temperature change was observed.
Literature shows that the heating efficiencies of the solar dryers or heaters vary widely depending on mass loaded in the system and the weather conditions of an area. In SODIS systems, typical efficiency values have been reported to fall within the ranges of 10 to 15% [34].
The coliforms are bacteria that originate mainly from the intestinal tract of warm-blooded animals. Their presence in any water source provides an indication of faecal contamination and the disease-causing microorganisms [35]. The effectiveness and efficiency of the developed SODIS system were determined by using contaminated raw water samples collected from rural areas of Chisenga and Chitipa (Malawi). The standard operating procedures and guidelines were followed to collect, preserve and transport the water samples used for SODIS system testing: Malawi Standards (MS) 682-1:2002 [36], MS 682-3:2002 [37], and American Public Health Association (APHA) [38]. Thus, adherence to standard protocol helped to prevent any cross-contamination and alteration of the samples. The samples were analysed for faecal and total coliform in triplicates in accordance with standard procedures [39]. The bacteria were analyzed using the membrane filtration method within a 24 h required period after sampling. Both the raw and treated water samples (100 mL) were filtered through a special membrane (0.20 µm pore size; 47 mm diameter). Petri dishes containing filter membranes and selective nutrient media (M-Lauryl Sulphate Broth) were incubated at 44.5 ± 0.5 °C for 24 h. The number of bacteria colonies obtained were expressed as colony-forming units (cfu) per 100 mL. The choice of using water samples from the two areas followed the results of a baseline study on the assessment of microbial contamination of drinking water sources where gross contamination of the drinking groundwater was reported [40]. The turbidity levels of the water samples were previously evaluated to be below 30 Nephelometric Turbidity Unit (NTU); hence the SODIS System testing with such water samples was practicable and in line with the literature [41]. Further, a comparison of inside system temperature to those in ambient was done to evaluate the efficacy of the enhancements made to the SODIS system.
Considering the market study and costs obtained for the different materials used to build the system, the cost of the SODIS system was evaluated. The social feasibility and product acceptability of the system was not evaluated due to the time and financial constraints of the study.
To ensure quality control and assurance, distilled water samples (<0.5 NTU) were used as controls in the microbiological water quality analyses. One set of the distilled water samples were spiked with coliforms while the other set was not spiked. The control samples were used to check the effects of turbidity on the disinfection efficiency of the system. These control samples were prepared and run concurrently in the SODIS system with the raw and untreated water samples. The raw water samples were collected in triplicate to improve the accuracy and reliability of the results. Prior to their reuse, the PET bottles were disinfected and cleaned with a dilute hypochlorite solution and washed thoroughly with a detergent. Before filling with the water samples, the bottles were further rinsed with distilled thrice followed by the water used in the experiments.
One-way Analysis of Variance (ANOVA) and t test were used to determine the variation and correlation of the bacterial contamination and disinfection efficiency of the water samples. To ensure the accuracy of the thermocouples used in temperature measurements, calibration using a mercury thermometer was done. The data and descriptive statistics such as averages, ranges, and standard deviations were performed using MINITAB and Microsoft Excel (2013). The temperature variation was analyzed by checking trends from graphs drawn using Microsoft Office Excel (2013). The microbiological quality results of the treated and untreated water were compared with the MS678 [42] and World Health Organisation (WHO) drinking water guidelines [43].
This section discusses the results on the disinfection system performance, the thermal analysis and efficiency of the system as well as the economic feasibility and comparative benefits of the system. A comparison with other studies conducted elsewhere is also given to demonstrate the significance of our developed disinfection system.
The following sub-sections give a discussion on the disinfection system performance. The results and discussion on ambient and the disinfection system temperature variations for the two days of testing are also provided. Furthermore, the subsequent sections give the results and discussion on the application to real water samples and the effectiveness of the SODIS system in water disinfection.
A. Ambient and the disinfection system temperature variations. The system was tested for two days – the first day on 13th September 2014 (10:33 am- 14: 33 pm) and the second day on 25th November 2014 (9:33 am - 13:33 pm). [Figure 3] and [Figure 4] show the line graph of temperature recording for the first and second day of SODIS system testing, respectively.
On the first day, the temperature inside the system rose from --25.3 to a maximum of 64.3 °C ([Figure 3]). The maximum temperature difference between the temperature inside and those in the ambient were- 36.4 °C. The mean water temperature inside the bottle rose from 24.3 °C to 66 °C. The temperature rise inside the system was attributed to the thermal enhancement in the system. A 50 °C temperature is recommended for disinfection and such the system surpassed this threshold, meaning it was effective [27] . On the second day, the inside system temperature rose from an initial minimum value of 26.5 °C to a maximum temperature of 69.7 °C ([Figure 4]). The maximum temperature difference between the inside SODIS system and the surrounding was- 36.4 °C. The mean bottle water temperature rose from an initial temperature of 24.8 °C to 68.7 °C. In this SODIS design, the corrugated aluminium sheets and the PET bottles were painted black to enhance heat absorption. This promoted the heating capacity of the entire system, and this is consistent with previous research studies conducted elsewhere notably Ubomba-Jaswa et al. [44], Berney et al. [45], and Atikul [46]. The transmission properties and characteristics of the PET plastic bottles used in this study are similar to those reported by Dejung et al. [47].
Line graph of temperature recording on the first testing day
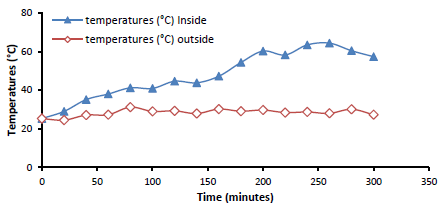
Line graph of temperature recordings on the second testing day
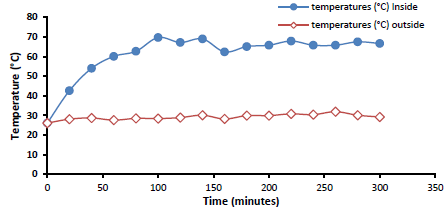
B. Application to real water samples. To evaluate the effectiveness of the SODIS system in water disinfection, microbiological water quality analyses were performed using shallow well water samples obtained from Chitipa. The specific areas where water samples were collected were Chisenga (C1a, C1b, C1c), Magomero (M2a, M2b, M2c) and Namkuntha (N3a, N3b, N3c). [Figure 5] shows the microbiological characteristics of the water samples used for SODIS system testing.
The system efficacy and effectiveness were evaluated after 3, 4 and 5 hours of exposure regimes in the SODIS prototype. [Table 2] and [Table 3] show a summary of the disinfection time for coliforms in the water samples. It is evident that almost all the coliform organisms (total coliform and feacal coliform) were killed within a period of 3 and 5 hours of exposure as indicated in both the results. After exposure to sunlight for 3 hours, the SODIS treatment of the samples generally resulted in nil total coliform count (0 cfu/ 100 mL) except for one from Magomero (1 cfu/100 mL) ([Table 2]; [Table 3]). This was attributed to poor handling of the sample resulted from human errors. Generally, the SODIS system successfully reduced the number of bacteria under study to the levels required for drinking water. Similar results where SODIS treatment was used to kill and inactivate microorganisms in water have been reported extensively in literature including in Nepal by Rainey and Harding [48], and in rural coastal Bangladesh by Islam et al. [46].
According to literature the thermal inactivation of E. coli has been reported to normally occur at temperatures above 40 °C [45],[49]. In this study, this minimum temperature of 40 °C was reached after about 80 minutes of water exposure during the first and second days of testing, respectively ([Figure 3] and [Figure 4]). The system reached the required temperature to kill all the microorganisms present. The results in the current study are consistent with a study by Ubomba-Jaswa et al. [44] who reported on complete bacteria inactivation temperatures between 45 and 55 °C after 5 hours of exposure of the water samples in SODIS.
Microbiological characteristics of the water samples for DAY 1 and DAY 2. Samples from Chisenga (C1a, C1b, C1c), Magomero (M2a, M2b, M2c) and Namkuntha (N3a, N3b, N3c). For DAY 1, other sets of distilled water samples were spiked with coliforms
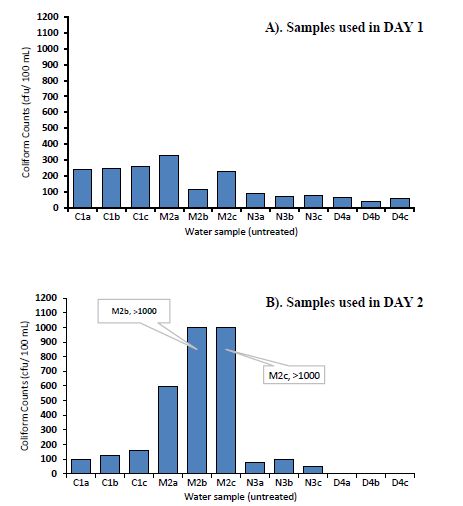
Disinfection time for coliforms in the water samples: all counts are in cfu/100 mL units
Name of well and sample number | ||||||||||||
---|---|---|---|---|---|---|---|---|---|---|---|---|
Time elapsed | Chisenga | Magomero | Namkuntha (Ipota) | Distilled water (control) | ||||||||
Sample 1 | Sample 2 | Sample 3 | Sample 1 | Sample 2 | Sample 3 | Sample 1 | Sample 2 | Sample 3 | Sample 1 | Sample 2 | Sample 3 | |
Raw (0 hrs) | 240 | 248 | 262 | 332 | 114 | 228 | 88 | 72 | 80 | 68 | 40 | 56 |
3 hrs | bd | bd | bd | 1 | bd | bd | bd | bd | bd | bd | bd | bd |
4 hrs | bd | bd | bd | bd | bd | bd | bd | bd | bd | bd | bd | bd |
5 hrs | bd | bd | bd | bd | bd | bd | bd | bd | bd | bd | bd | bd |
bd: below detection
Disinfection time for coliforms in the water samples: all counts are in cfu/100 mL units
Name of well and sample number | ||||||||||||
---|---|---|---|---|---|---|---|---|---|---|---|---|
Time elapsed | Chisenga | Magomero | Namkuntha (Ipota) | NRWB Tap water (control) | ||||||||
Sample 1 | Sample 2 | Sample 3 | Sample 1 | Sample 2 | Sample 3 | Sample 1 | Sample 2 | Sample 3 | Sample 1 | Sample 2 | Sample 3 | |
Raw (0 hrs) | 100 | 124 | 160 | 596 | TNT | TNT | 80 | 96 | 52 | bd | bd | bd |
3 hrs | bd | bd | bd | bd | bd | bd | bd | bd | bd | bd | bd | bd |
4 hrs | bd | bd | bd | bd | bd | bd | bd | bd | bd | bd | bd | bd |
5 hrs | bd | bd | bd | bd | bd | bd | bd | bd | bd | bd | bd | bd |
bd: below detection
A t-test was run for only the number of total coliforms after 3 hours because all the other values did not have a variance with the standards set by the WHO and Malawi Bureau of Standards (MBS). The t-test was set to assess if the water treated using the prototype met the WHO and MBS Total coliforms set standards in which a t of 1.00 was obtained, which with 8 degrees of freedom was significant at the 0.347 level. This, therefore, showed that the water was safe for drinking and that the prototype was effective in water treatment.
The effectiveness of a SODIS technology is measured by its thermal conversion of solar energy to sufficiently raise the water temperature to kill the pathogens [28] , which constitute one of the acute health risks of using water that is contaminated in low and middle-income households [50]. The optical properties of the materials used in the construction of the SODIS play a significant role in the thermal performance of the SODIS [15] . This section provides the values obtained from the calculation done using the equations outlined earlier and summarized in [Table 4] and [Table 5].
The heat energies that entered the system through the plastic into the water (Qf) during the first and second day of testing were 2,095.18 and 2,205.71 kJ, respectively. Although these values differed slightly attributed to changes in sunlight intensity, the differences were not significant (p > 0.05). The rates of heat transfer obtained in this study were 0.20 and 0.21 kW for the first and second day of testing, respectively. The heat gains or loss rates, on the other hand, were found to be 12.91 and 12.26 W ([Table 4]).
Thermal analysis of the SODIS on first day of testing the prototype
Variable determined for DAY 1 of testing | Constant or calculated figures |
---|---|
Solar constant I for Mzuzu | 823 W/m2 |
Heat entering the system: , the values found where m = 12 kg, c = 4.187 kJ/kgoC, | 2,095.18 kJ |
The rate of heat transfer: , , | 0.2 kW |
The heat received by the system from the sun: where I was found to be 823 W/m2, 0.0495 m2 | 407.8 W |
Incorporating transmissivity and absorptivity product to get Qi | 26.65 W |
The heat gain or loss rate was calculated by | 12.91 W |
Thermal analysis of the SODIS on the second day of testing the prototype
Variable determined for DAY 2 of testing | Constant or calculated figures |
---|---|
Solar constant I for Mzuzu | 823 W/m2 |
Heat entering the system: , the values found where m = 12 kg, c = 4.187 kJ/kgoC, ΔT = (68.9-25.0)oC | 2,205.71 kJ |
The rate of heat transfer: , where , | 0.21 kW |
The heat received by system from the sun: where I was found to be 823 W/m2, 0.0495 m2 | 407.8 W |
Incorporating transmissivity and absorptivity product to get Qi | 26.65 W |
The heat gain or loss rate was calculated by | 12.26 W |
The efficiency of the system (η) was determined using eq. (8) as the mass m was 12 kg, c was 4.187 kJ/kgoC, I was 823 W/m2(J/m2s), t was 5 minutes (300 seconds) and there was a minute temperature change 2.5 oC.
The SODIS system performed fairly well on a sunny day as it was on the second day of testing. Its efficiency was 10.2% which was fairly good compared to the very best achieved efficiency of 15% reported in the literature [44].
The SODIS are designed to be effective (in providing safe water), available, affordable and acceptable to the low and medium-income households, which are mostly affected by outbreaks of waterborne diseases [10] . The SODIS system developed in this study has several benefits over other points of use water treatment options such as Water Guard, chlorination and boiling water treatment commonly used in rural areas of Malawi. The cost of the SODIS system was US$ 32.45 compared to the retail price of Water Guard, which was at US$ 0.32/L during the time of this study. This implies that a household has to buy at least 80 bottles of Water Guard to reach the system’s cost of water treatment achieved by the SODIS developed in this study. Furthermore, the use of Water Guard may put the household at risk if the household has no reliable source of income, unlike incurring the cost of purchasing the SODIS system once and for all. In addition, the supply chain of water guard in rural areas in Malawi is challenging, requiring households to travel to urban centres to source it, which in turn increases the cost per unit of disinfected water. The SODIS system is also better than boiling using biomass, either in wood or crop residue form as people spent time for biomass collection. The distance for collecting biomass for thermal applications at the household level in Malawi has increased over time. The supply chains of agricultural residues that can be used for boiling water are seasonal and have many competing uses [51], which exacerbate the sustainability challenge of the agricultural residues supply chain for water heating. In addition, if burnt in a room, biomass produces a lot of soot or smoke [52], which is a risk factor for lower respiratory functions causing a majority of deaths among children [53]. Furthermore, the use of biomass contributes to environmental degradation as it is estimated that a kilogram of wood is required to boil 1 litre of water. People also dislike the taste that boiling treatment method of water imparts on the water [24] . A study done in Chikwawa – Malawi on the use of Water Guard to treat water showed several residents did not use it due to cost implications [54]. Several studies in the country showed that the majority of people in the rural areas use untreated contaminated water fetched from shallow wells, rivers [55] and groundwater (boreholes) [56]. Use of the SODIS in treating the contaminated water can have positive health benefits among users in the country. This has been demonstrated in other countries such as in Bangladesh [46] , Cameroon [14] and Bolivia [57], where use of the solar disinfection system is being promoted.
The efficacy and effectiveness of the system, auger well with the goals and targets of the SDG 6, the SADC Water Policy (2005) and Malawi National Water Policy (2005). The developed SODIS system, if properly put to use can help promote and improve access to safe and affordable drinking water for all – especially in the rural areas. Since the system does not require use of any form of fuel or electricity, it can significantly help communities treat water for home use. Furthermore, use of the system can help conserve the forests and other forms of energy. The previous SODIS studies were performed in Yaounde, Cameroon [14], Nepal [48], Bolivia . [47] and many other places worldwide, have demonstrated the significance of solar disinfection. The results of the current study has also demonstrated the need for use of SODIS in rural areas where access to clean and potable water is a challenge. The Malawi National Energy Policy (2018) aims at “increasing access to affordable, reliable, sustainable, efficient and modern energy for every person in the country”. This policy is also in tandem with SDG 7, “promoting the use of affordable and clean energy” [7]. The use and promotion of clean and sustainable energy (solar) in the disinfection system to treat water are, therefore, in line with the aims and goals of the Malawi national energy policy and water policy as well the SDG 7.
This study designed and constructed a prototype of a solar disinfection system for improved rural household water treatment. Its effectiveness in killing microorganisms was evaluated through thermal analysis and measuring the microbiological water quality parameters. The systematic analysis and combination of previous and known basic design concepts resulted in improving the prototype of the disinfection system. The prototype was very effective in disinfecting contaminated water from all the shallow wells after exposure in the disinfection system for at least 3 hours. The treated water using the SODIS met both WHO (2008) and MBS (2005) microbiological set standard of 0 cfu/100 ml. Thermal analysis showed that the enhancement made to the system under study significantly improved the performance of the system. The prototype raised the inside system temperature by a threshold of at least 10 °C and this significantly reduced inactivation time to 3 hours compared to ordinary disinfection systems that take about 6 hours.
Basing on the current findings, the following recommendations can be drawn: (a) the study used bacteria to determine if the water was safe after treatment. However, there is need to check other organisms such as protozoa and spore-forming cysts to confidently declare the water treated under the disinfection system is safe: (b) to the user of the system, longer exposure times is required for cloudy weather conditions, to achieve the required reduction of coliforms in water, (c) government and other organisation should promote use of solar disinfection systems to help improve access to safe drinking water and reduce health risks among residents in rural areas.
- , Evaluating solar disinfection for point-of-use water treatment in non-tropical climates, 2002
- WHO | World Health Organization, 2020, https://www.who.int/
- ,
World Health Organization and the United Nations Children’s Fund (UNICEF), Progress on Drinking Water, Sanitation and Hygiene: 2017 Update and SDG Baselines; WHO : Geneva, Switzerland , 2017 - , Solar disinfection of drinking water, 2003
- ,
Diarrhoeal disease , 2020 Molecular characterization of bacterial isolates and physicochemical assessment of well water samples from hostels at Osekita, Iworoko-Ekiti, Ekiti State ,Am. J. Microbiol. Res , Vol. 6 (1),pp 22–32 , 2018
, - Sustainable Development Goals | United Nations Development Programme, 2020, https://www.undp.org/sustainable-development-goals
- Regional Water Policy. Sadc-2005, 2005, https://www.sadc.int/files/1913/5292/8376/Regional_Water_Policy.pdfTitle
- , Malawi National Water Policy. Ministry of Irrigation and Water Development, Cap. Print. Press, 2007
Microbiological evaluation of 5 L-and 20 L-transparent polypropylene buckets for solar water disinfection (SODIS) ,Molecules , Vol. 24 (11),pp 2193 , 2019
, Solar water disinfection (SODIS): a review from bench-top to roof-top ,J. Hazard. Mater , Vol. 235 ,pp 29–46 , 2012
, Capability of 19-L polycarbonate plastic water cooler containers for efficient solar water disinfection (SODIS): Field case studies in India, Bahrain and Spain ,Sol. Energy , Vol. 116 ,pp 1–11 , 2015
, Community challenges when using large plastic bottles for Solar Energy Disinfection of Water (SODIS) ,BMC Public Health , Vol. 16 (1),pp 1–8 , 2016
, Health gains from solar water disinfection (SODIS): evaluation of a water quality intervention in Yaounde, Cameroon ,J. Water Health , Vol. 8 (4),pp 779–796 , 2010
, Accelerating the process of solar disinfection (SODIS) by using polymer bags ,J. Chem. Technol. Biotechnol , Vol. 92 (2),pp 298–304 , 2017
, Roof-harvested rainwater for potable purposes: application of solar collector disinfection (SOCO-DIS) ,Water Res , Vol. 43 (20),pp 5225–5235 , 2009
, The Meanings of Water: Socio-Cultural Perceptions of Solar Disinfected (SODIS) Drinking Water in Bolivia and Implications for its Uptake ,Water , Vol. 12 (2442), 2020
, SODIS manual: Guidance on solar water disinfection ,Eawag, Switz , 2016
, Validation of a solar-thermal water disinfection model for Escherichia coli inactivation in pilot scale solar reactors and real conditions ,Chem. Eng. J , Vol. 331 ,pp 831–840 , 2018
, Solar Disinfection in a Community-Scale Water Treatment System: An Analysis of Design Parameters for Humanitarian Engineering and System Sustainability ,Int. J. Serv. Learn. Eng. Humanit. Eng. Soc. Entrep , Vol. 8 (1),pp 88–101 , 2013
, - , , Brock biology of microorganisms, 20032003
Intracellular mechanisms of solar water disinfection ,Sci. Rep , Vol. 6 (1),pp 1–10 , 2016
, - , Status of National Household Water Treatment and Safe Storage Policies in Selected Countries- Results of global survey and policy readiness for scaling up. World Health Organisation-WHO. Geneva 27, Switzerland, WHO/HSE/WS, 2012
Solar disinfection of drinking water and oral rehydration solutions , 1984
, Cost-effectiveness of water quality interventions for preventing diarrhoeal disease in developing countries ,J. Water Health , Vol. 5 (4),pp 599–608 , 2007
, Design and development of a dual solar water purifier ,Int. J. Adv. Sci. Eng. Technol. Res , Vol. 2 (1),pp 8–17 , 2013
, - ,
Solar water Disinfection: A guide for the application of SODIS. Swiss Federal Institutes of Science and Technology (EAWAG) Department of Water & Sanitation Developing Countries (SANDEC) Report No 06/02, Switzerland ,ITDG Publ , 2002 Detrimental vs. beneficial influence of ions during solar (SODIS) and photo-Fenton disinfection of E. coli in water: (Bi)carbonate, chloride, nitrate and nitrite effects ,Appl. Catal. B Environ , Vol. 270 ,pp 118877 , 2020, https://doi.org/10.1016/j.apcatb.2020.118877
, Development of renewable energy technologies in Malawi , 2013
, - , General Chemistry, Cengage Learn. Boston, 2017
Validation of the existing models for estimating global solar radiation over Egypt ,Energy Convers. Manag , Vol. 50 (1),pp 184–193 , 2009
, Solar radiation estimates from common parameters ,Sol. energy , Vol. 11 (3–4),pp 170–172 , 1967
, Proposal for new world standard for testing solar cookers ,J. Eng. Sci. Technol , Vol. 4 (3),pp 272–281 , 2009
, - , , Solar dryers: their role in post-harvest processing, 1985
- ,
Guidelines for Drinking Water Quality, 4th ed.; Incorporating the First Addendum; World Health Organization (WHO): Geneva, Switzerland , 2017 - , Water Quality—Sampling Part 1—Guidance on the Design of Sampling Programmes and Sampling Techniques; MS 682-1:2002; Malawi Bureau of Standards (MBS): Malawi, Blantyre, 2002
- , Water Quality—Sampling. Part 1—Guidance on the Preservation and Handling of Water Samples; MS 682-3:2002; Malawi Standards Board; Malawi Bureau of Standards-MBS: Malawi, Blantyre, 2002
- , Standard Methods for the Examination of Water and Wastewater; American Public Health Association (APHA): Washington, DC, USA; American Water Works Association (AWWA): Washington, DC, USA; Water Environment Federation (WEF): Washington, DC, USA, 2005
- , Method 1603: Escherichia coli (E. coli) in Water by Membrane Filtration Using Modified membrane-Thermotolerant Escherichia coli Agar (Modified mTEC); U.S. Environmental Protection Agency (EPA): Washington, DC, USA, EPA-821-R-09-007, 2009
- , Designing and Prototyping of Solar Disinfection System for Improved Rural Household Water Treatment, Undergraduate Thesis. Mzuzu University. Malawi, 2016
Optimizing the solar water disinfection (SODIS) method by decreasing turbidity with NaCl ,J. Water, Sanit. Hyg. Dev , Vol. 2 (2),pp 87–94 , 2012
, - , Malawi Standards-MS 678, Drinking Water quality – control and surveillance of water in public supply networks, Malawi Bureau of Standards, Blantyre, Malawi, 2005
- , World Health Organization, Guidelines for Drinking Water Quality, third ed., vol. 1. World Health Organization, Geneva, 2017
Solar disinfection of drinking water (SODIS): an investigation of the effect of UV-A dose on inactivation efficiency ,Photochem. Photobiol. Sci , Vol. 8 (5),pp 587–595 , 2009
, Efficacy of solar disinfection of Escherichia coli, Shigella flexneri, Salmonella Typhimurium and Vibrio cholerae ,J. Appl. Microbiol , Vol. 101 (4),pp 828–836 , 2006
, Effectiveness of solar disinfection (SODIS) in rural coastal Bangladesh ,J. Water Health , Vol. 13 (4),pp 1113–1122 , 2015
, Effect of solar water disinfection (SODIS) on model microorganisms under improved and field SODIS conditions ,J. Water Supply Res. Technol , Vol. 56 (4),pp 245–256 , 2007
, Drinking water quality and solar disinfection: effectiveness in peri-urban households in Nepal ,J. Water Health , Vol. 3 (3),pp 239–248 , 2005
, Solar disinfection of drinking water contained in transparent plastic bottles: characterizing the bacterial inactivation process ,J. Appl. Microbiol , Vol. 84 (6),pp 1138–1148 , 1998
, Material selection and prediction of solar irradiance in plastic devices for application of solar water disinfection (SODIS) to inactivate viruses, bacteria and protozoa ,Sci. Total Environ , Vol. 730 ,pp 139126 , 2020
, A synergetic integration of bioenergy and rice production in rice farms ,Renewable and Sustainable Energy Reviews , Vol. 75 ,pp 58–67 , 2017, https://doi.org/10.1016/j.rser.2016.10.051
, Determining the enablers and barriers for the adoption of clean cookstoves in the middle belt of Ghana—A qualitative study ,Int. J. Environ. Res. Public Health , Vol. 16 (7), 2019, https://doi.org/10.3390/ijerph16071207
, Drivers and barriers to clean cooking: A systematic literature review from a consumer behavior perspective ,Sustainability (Switzerland) , Vol. 10 (114322),pp MDPI AG ,pp 21 , 2018, https://doi.org/10.3390/su10114322
, Household use of water guard for treating drinking water in Chikwawa district, Southern Malawi ,Malawi J. Appl. Sci. Innov , Vol. 1 ,pp 2–9 , 2017
, Evaluation of groundwater quality in rural-areas of northern Malawi: Case of Zombwe Extension Planning Area in Mzimba ,Phys. Chem. Earth , Vol. 93 , 2016, https://doi.org/10.1016/j.pce.2016.03.013
, Assessment of drinking water quality and rural household water treatment in Balaka District, Malawi ,Phys. Chem. Earth , Vol. 100 , 2017, https://doi.org/10.1016/j.pce.2016.10.006
, Solar drinking water disinfection (SODIS) to reduce childhood diarrhoea in rural Bolivia: a cluster-randomized, controlled trial ,PLoS Med , Vol. 6 (8),pp e1000125 , 2009
,