The need for a renewable energy transition is nowadays undeniable [1]. Climate change, fossil fuel depletion and economic vulnerability are some of the main drivers of the model change [2]. The energy transition entails the expansion and integration of renewable and sustainable energy sources within existing energy supply systems, or the progressive replacement of existing systems for newer and more sustainable ones in an orderly manner [3], together with investment in green capital that enables the production of renewable energy [4].
Many rural regions in poor and developing countries lack reliable access to national power grids, and often have to rely on small- and medium-sized off-grid generators in order to obtain electricity, and biomass for heating and cooking applications [5]. . Fossil fuels are often the only modern primary energy resource for these communities to have the ability to use agricultural machinery, and for transportation and mobility. Otherwise, they have to rely on human and animal force for these tasks, which is a crippling limitation to their development [6]. Therefore, in order to promote sustainable growth in these communities, it needs to shy away from fossil fuels and rely on the local renewable energy sources.
Access to energy is one of the Millennium Development Goals (MDG), and a key factor to increase the quality of life of these communities [7]. In order to achieve the MDG, exploring and developing different renewable energy sources that are available to each of these communities can be a way for them to thrive in a post-peak oil scenario, and renewable solutions are to be preferred over fossil-fuel dependent infrastructure since electricity generation from renewable sources is increasingly efficient and competitive compared to fossil fuels [8]. As it is common for renewable energy development, the optimal solution is going to differ location to location.
In Ecuador, water resources are abundant. This has been by the Ecuadorian government to decarbonize the electric grid, and reduce fossil fuel dependency [9]. However, the approach has always been that of large hydropower facilities, while foregoing small, distributed generators for local use. In order to make the best use of this resource, the academy needs to actively participate in water management and to offer proposals for local electricity generation to guarantee sustainable social development [10].
This research proposes to design energy generation systems according to the context, that is, considering the availability of sustainable energy sources, materials, components or manufacturing technologies in the area where the system will operate. These context considerations must be kept in mind from the beginning of the project and throughout its execution.
A technical solution to profit energy from water is the use of artificial free-surface vortices to generate low and ultra-low power hydroelectric energy, a field that has experienced a special growth in the last two decades. This technology works on the principle of harnessing hydroelectric power from the high angular velocity generated in a vortex chamber [11].
Low head hydropower turbines are useful in situations where water is abundant, but a higher head can’t be obtained due to the lack of slope [12], as is the case of the Amazonian region of Ecuador. They are an increasingly popular solution to provide a reliable electricity supply to remote or otherwise isolated areas [13]. Due to their nature, the investment in infrastructure to install them is low, since this system works with hydraulic loads between 0.7 m - 2.0 m, without the need to generate water deposits through dams or other means to artificially obtain a higher head [14]. This makes them very competitive in terms of investment when compared to other micro-generator systems, such as solar PV or micro wind turbines [15].
They are categorized into two types, which are impulse and reaction turbines, each one suitable for different types of water flows and heads [16]. The main difference between the two types of turbines is the way water interacts with the rotor. Reaction turbines operate underwater and their torque comes to the potential energy created by the weight of water at the base of the head acting on one side of its blades [17]. Impulse turbines are not required to operate underwater, and they have an injector that project the water flow to the blades to generate the torque. This conceptual difference makes reaction turbines more common choice for low head systems. Considering the flow and head conditions for this project, Kaplan and Shaft type turbines were chosen as optimal solutions [18].
This technology is suitable to be applied to areas that have many rivers and sloping water channels [19]. These electricity generation systems can be arranged horizontally [20] or vertically [21], in this study the horizontal arrangement has been selected because a siphon will be included at the exit of the vortex.
To increase the flexibility of the system, it is designed in a modular fashion. This means that the concrete blocks that form the channel can be pre-built and brought to site for a quick installation, or built in place using formworks, according to factors such as the accessibility of the site. The construction using building blocks also means that the system can be relocated if required.
The design process included the analysis and simulation of the electrical energy conversion system, which was later validated against real data measured from the prototype [22].
The use of vortex and siphon hydropower plants presented in this document can be a support system for overcoming energy sustainability problems and boosting the renewable energy transition [23]. Therefore, the main objective of this study is to design and manufacture an underwater turbine with a gravitational vortex-siphon, which is flexible and modular and allows the use of low-head hydraulic resources in the territory. The exact range of this project in terms of head and flow is 1 m to 2 m and 0.01 m3/s to 1 m3/s respectively. Finally, this document demonstrates the results of the design, installation and field measurements of a vortex-siphon Underwater Turbine Generator UTG.
The system was designed with a stage-based method to design machines and products [24], [25]. The stages shown in the [Figure 1].
Design method
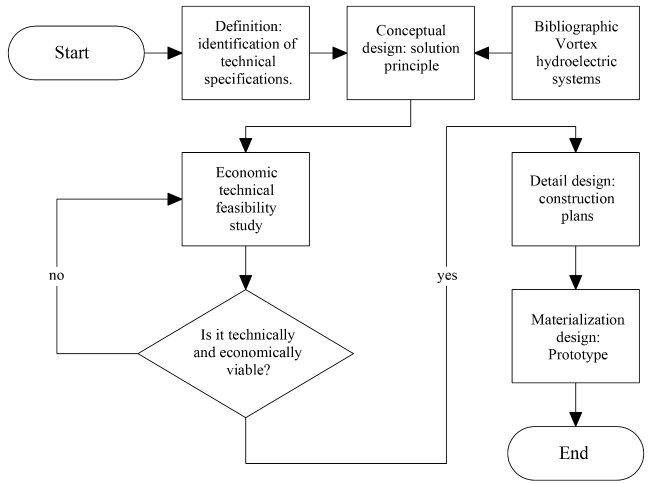
The studied Underwater Turbine Generator system employs the flowing water energy of the artificial irrigation ditches of the hydraulic laboratory of the Universidad Técnica Particular de Loja, Ecuador. The gravitational vortex with a siphon uses the concentrated rotational energy from the centre of a whirlpool which consists of a vertical axis turbine which is located at the outlet of the vortex for energy production and a siphon which serves to increase the head [Figure 2].
System configuration
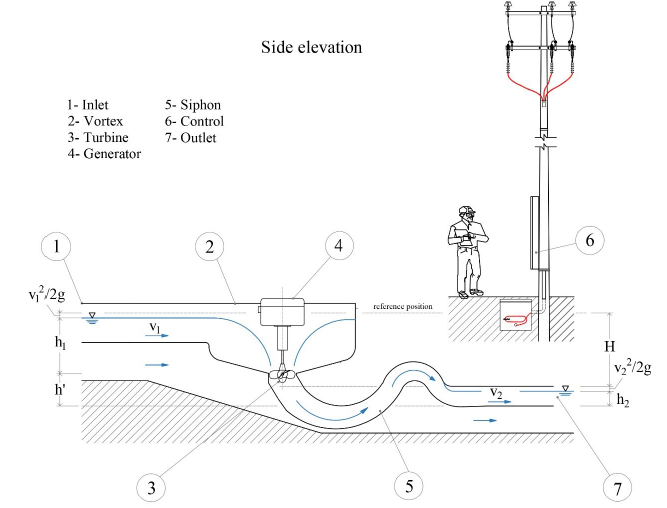
[Table 1] shows the system specifications, having an inflow rate of about 0.8 m3/s is constructed and it approximately results in a vortex of 3 m3 of water circulating inside the tank which has a diameter of 2.0 m and a head of 2 m.
System specifications
Diameter turbine: d | 0.48 [m] |
Diameter vortex: D | 2.00 [m] |
Height max: H | 2.00 [m] |
The angular velocity ω and torque T were measured. The turbine output was obtained by:
(1)
(2)
where is the hydraulic power, H is the hydraulic head, γ is the specific weight of the water, is the flow rate. The effective head H is defined by the following as shown in [Figure 2]:
(3)
In addition, the turbine efficiency η was calculated by:
(4)
Generators convert mechanical energy into electrical energy. The electric power η was calculated by:
(5)
Results presented in this chapter are based on the design of an underwater turbine generator. Furthermore, the experimental measurements of energy generation are presented, these results are examined on annual level in terms of costs and production capacities.
The proposed underwater turbine was designed using Computer-Aided Design (CAD). The complexity of the machine – number of parts, weight and complexity of assembly was reduced before building the prototype. Additionally, an analysis of interference in software engineering was performed to check tolerances.
[Figure 3] shows the 3D configuration. The shapes, dimensions and finishing properties of all parts and components of the UTG are defined.
3D design of UTG
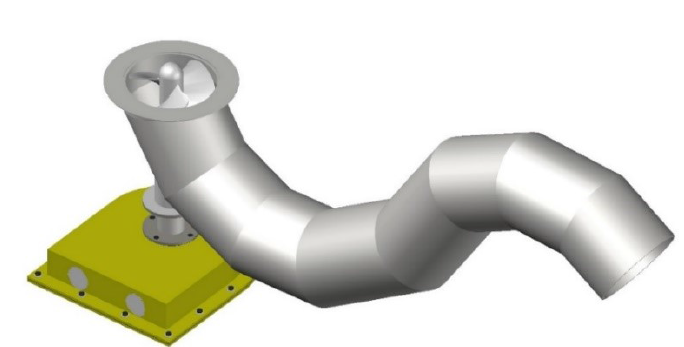
The turbine-generator module with a siphon was installed at the output of vortex to evacuate water. [Figure 4] shows the set employed. In this case, the siphon was used to pull in water from the turbine toward ditches or channels outside the plant.
Turbine with siphon module
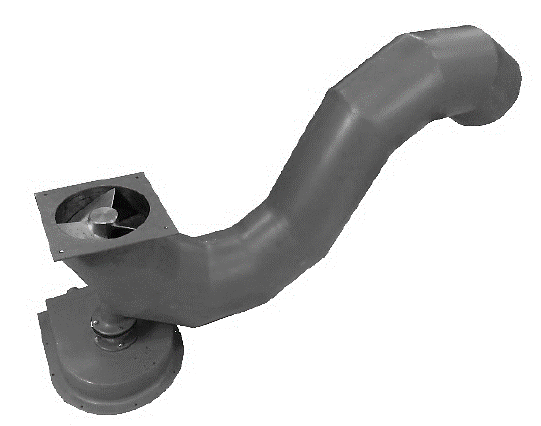
The generator was implemented with the following specifications: 3-phase, rated power of 7.5 kW, rated line voltage of 220 V, rated frequency of 60 Hz, 2 poles, rated rotational speed of 1800 min-1. In this case, a small pilot exciter was used in order to make the excitation generator completely independent of any external power sources.
The rotor side Passive Rectifier Insulated Gate Bipolar Transistor (PR-IGBT) and Grid Side Inverter Insulated Gate Bipolar Transistor (GSI-IGBT) have been designed to be connected loads, [Figure 5].
General structure of control
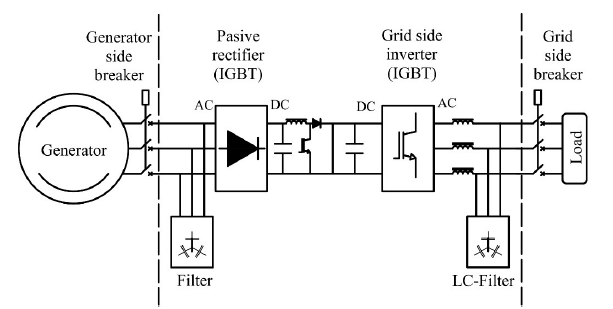
Additionally, a monitoring and control system for the electric variables was installed in order to optimize the microgrid and help its integration to the power system [26].
The controller is designed to adjust the turbine speed to extract the maximum power from the water resource. The PI controller is usually designed for this purpose according to the estimated parameters either offline or online, and the observed disturbance torque is fed forward to increase system robustness [27]. But this type of typical controller is not enough to serve the purpose efficiently. The general structure of control shown in [Figure 6].
General structure of control
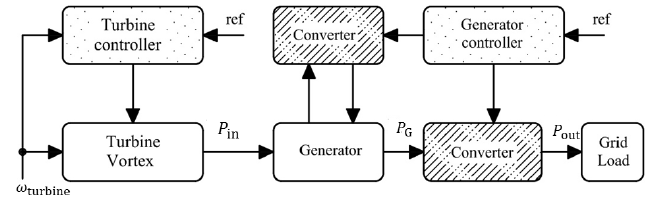
The measurements were made with the AR6 network analyser from the company CIRCUITOR [28]. Our study case will experiment with a usual setting of 220 V at 60 Hz.
The following graphs show measures of different variables like the voltage, intensity and frequency, over a period of 5 days.
Voltage measurements
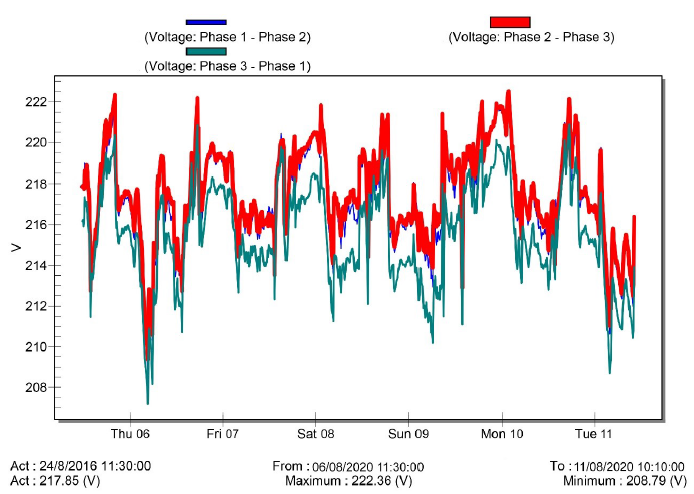
The voltage has so far rarely deviated more than 2.56% from 220 V. The measurements shown in [Figure 7].
Intensity measurements
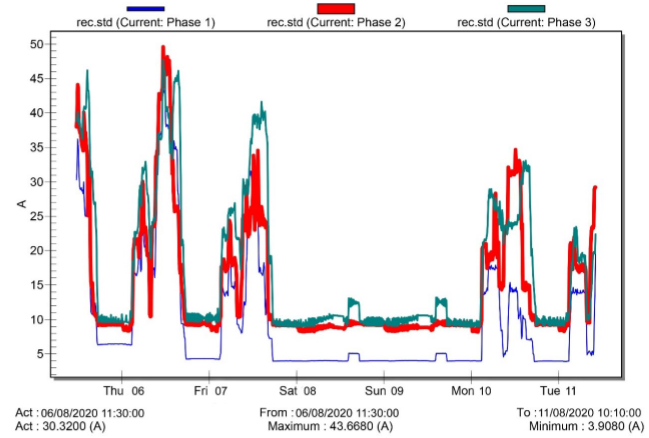
The intensity maximum under the load was 43A in phase 2, as shown in the [Figure 8]. In addition, it is observed that when loads are applied, the voltage and frequency values remain at acceptable levels.
[Figure 9] shows the frequency over a period of 5 days. The frequency stays within a range of 60 Hz ± 0.1 Hz.
Frequency measurements
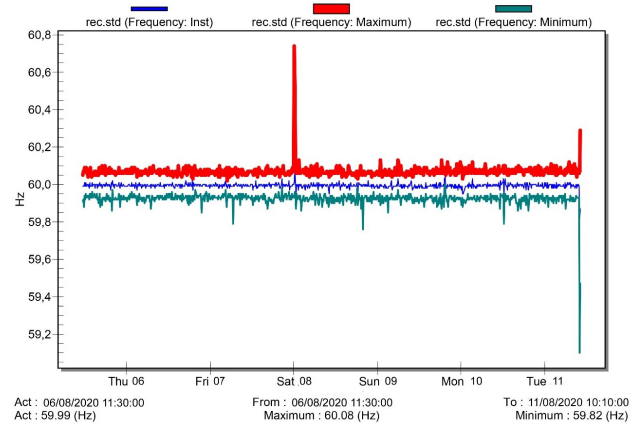
The system behaves quite well in normal operating conditions. Protections for under-speed and over-speed are automatically triggered. If the system speed fluctuates, the injected active power fluctuates accordingly.
According to the field measurements, the torque T decreases and the number of revolutions per minute n increases (see [Figure 10]). The total flow of the turbine is 0.8 to 1 m3/s with a head difference of 1 to 2 m respectively. It gives approximately 7.5 kW of useful electrical energy with an efficiency of 35 to 37%, at the rotational speed 1100 min-1 to 1300 min-1 respectability.
Torque and under water turbine output
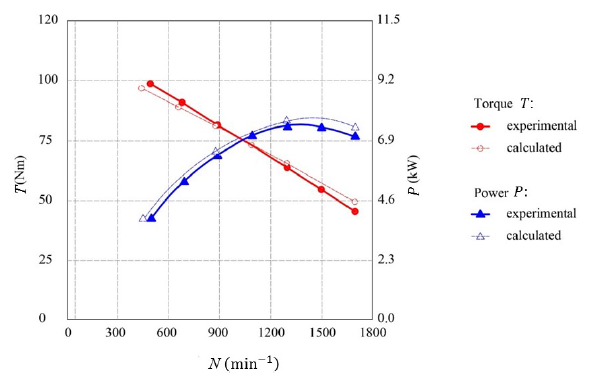
A comparison between the experimental and calculation results for this under water turbine in relation to its performance is shown in [Figure 11]. Under water turbine efficiency η show maximum values at the rotational speed . The maximum experimentally determined efficiency is approximately 0.37.
Under water turbine efficiency and effective head
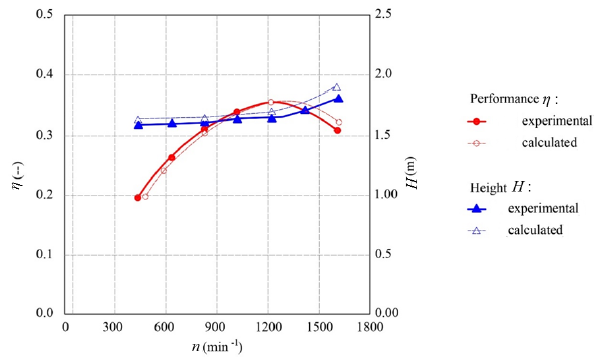
In this section, an evaluation of the cost was made and compared with the savings generated by electricity generation in order to determine its profitability. [Table 2] shows the costs associated with the construction of the system.
Comparison between theory and experiment
Description | Cost [$] |
Under water turbine | 3 000 |
Canal and vortex | 6 000 |
Electrical components | 5 000 |
TOTAL | 14 000 |
Electric power of a hydro power plant is calculated by flow rate, head and total efficiency factor. Therefore, the annual working capacity can be estimated by the electric power and the equivalent full load hours.
In [Table 3], the monthly generation is shown. Using 2019 data, it was determined that the generation of the hydroelectric group was 52 727 kWh with 4157 hours of operation. On the other hand, it can be seen that in the months of June to October the low generation due to the dry season.
Annual generation
Month | hours/month | Generation [kWh] |
January | 682 | 8 866 |
February | 504 | 6 552 |
March | 496 | 6 448 |
April | 420 | 5 148 |
May | 310 | 3 828 |
June | 210 | 2 730 |
July | 248 | 3 224 |
August | 186 | 1 813 |
September | 180 | 2 340 |
October | 279 | 3 627 |
November | 270 | 3 315 |
December | 372 | 4 836 |
TOTAL | 4157 | 52 727 |
The annual savings were determined knowing that the rate of electric energy in Ecuador is 0.058 $/kWh:
Maintenance and operating expenses are subtracted from the total cost generated. Therefore, the net flow of savings per year is:
The Pay-Back was determined. It is the minimum value of the period necessary for the return of an investment:
The return on investment would be realized in an average of 14 years.
The profitability of the installation is around 7.5% per year, assuming that the electric company buys all the energy generated by the system:
This paper introduces a low power Underwater Turbine Generator that was developed to produce local and renewable electricity that can help in the path of the energy transition. The system was designed to provide an affordable access to a renewable electricity supply to rural or isolated communities in Ecuador and other Andean countries, using the ubiquitous hydric resources that characterize the region. It also reduces the dependency of these communities on fossil fuels or the labour intensive biomass.
In order to ensure the accessibility and affordability of the system, it was designed using technology and materials that are available to rural communities. Furthermore, it has a modular design that allows it to be quickly built and deployed, while also providing flexibility both in power generation capabilities, and adaptability to the site. At the end of the use, the system can be uninstalled leaving very little impact.
In normal operating conditions, the efficiency for head differences larger than 1.5 m is 30%. The total flow of the turbine is 0.8 to 1 m3/s with a head difference of 1.5 to 2 m respectively. It gives 7.5 kW of useful electrical energy with an efficiency of 35 to 37%, with good supply quality and stability delivered to its loads. A good use of the system can achieve more than 5000 hours at full load, just like a conventional hydroelectric plant.
A prototype of the system was built in the laboratory of the Universidad Técnica Particular de Loja. The test runs produced the expected results in terms of output and quality.
This research also produced a model of the Underwater Turbine Generator. This model was validated using empirical readings of the test runs of the prototype, and can be used to assess and predict electricity outputs in settings other than the laboratory. It was coded using open software, so that the code can be accessed by the users.
Future energy scenarios on a different scale of implementation are suggested. Furthermore, this type of design can be reproduced in another context in order to promote the decentralized generation and sustainable growth between the territory and its resources. Underwater power generator based on gravity vortex siphon generate green electricity to fund themselves and make a contribution to environmental protection.
The Grand Challenge of the Energy Transition ,Front. Energy Res , Vol. 1 (2), 2013, https://doi.org/10.3389/fenrg.2013.00002
, Depletion of fossil fuels and anthropogenic climate change—A review ,Energy Policy , Vol. 52 ,pp 797-809 , 2013, https://doi.org/10.1016/j.enpol.2012.10.046
, The coming sustainable energy transition: History, strategies, and outlook ,Energy Policy , Vol. 39 (11),pp 7422-7431 , 2011, https://doi.org/10.1016/j.enpol.2011.09.009
, Climate change and the transition to a low carbon economy – Carbon targets and the carbon budget ,Econ. Model , Vol. 84 ,pp 367-376 , 2020, https://doi.org/10.1016/j.econmod.2019.04.026
, Energy, Electricity and Socioeconomic Transformation of Rural Regions in Developing Countries ,Energy Sources, Part B Econ. Planning, Policy , Vol. 6 (4),pp 384-394 , 2011, https://doi.org/10.1080/15567240802458849
, Lessons learned from rural electrification initiatives in developing countries: Insights for technical, social, financial and public policy aspects ,Renew. Sustain. Energy Rev , Vol. 102 ,pp 35-53 , 2019, https://doi.org/10.1016/j.rser.2018.11.035
, Electricity, income, and quality of life ,Soc. Sci. J , Vol. 53 (1),pp 33-39 , 2016, https://doi.org/10.1016/j.soscij.2014.12.009
, Integrated modelling of variable renewable energy-based power supply in Europe ,Energy , Vol. 123 ,pp 173-188 , 2017, https://doi.org/10.1016/j.energy.2017.01.115
, Managing the Water-Energy Nexus within a Climate Change Context—Lessons from the Experience of Cuenca, Ecuador ,Sustainability , Vol. 11 (21), 2019, https://doi.org/10.3390/su11215918
, Challenges for a sustainable management of Ecuadorian water resources , Vol. 6 (6),pp 101–106 ,
, Performance and Flow Field of a Gravitation Vortex Type Water Turbine ,Int. J. Rotating Mach , Vol. 2017 ,pp 2610508 , 2017, https://doi.org/10.1155/2017/2610508
, Ultra-low-head hydroelectric technology: A review ,Renew. Sustain. Energy Rev , Vol. 78 ,pp 23–30 , 2017, https://doi.org/10.1016/j.rser.2017.04.086
, Sustainable and economical small-scale and low-head hydropower generation: A promising alternative potential solution for energy generation at local and regional scale ,Appl. Energy , Vol. 188 ,pp 378-391 , 2017, https://doi.org/10.1016/j.apenergy.2016.12.012
, Preliminary experimental study on multi-stage gravitational water vortex turbine in a conical basin ,Renew. Energy , Vol. 145 ,pp 2516-2529 , 2020, https://doi.org/10.1016/j.renene.2019.07.128
, Operation, performance and economic analysis of low head micro-hydropower turbines for rural and remote areas: A review ,Renew. Sustain. Energy Rev , Vol. 43 ,pp 40-50 , 2015, https://doi.org/10.1016/j.rser.2014.11.045
, Low head pico hydro turbine selection using a multi-criteria analysis ,Renew. Energy , Vol. 61 ,pp 43-50 , 2014, https://doi.org/10.1016/j.renene.2012.06.020
, - , Chapter 2 - Main Equipment, 2019
Local installation methods for low head pico-hydropower in the Lao PDR ,Renew. Energy , Vol. 44 ,pp 439-447 , 2012, https://doi.org/10.1016/j.renene.2012.01.089
, Design and analysis of a portable spiral vortex hydro turbine for a Pico Hydro Power Plant ,IOP Conf. Ser. Mater. Sci. Eng , Vol. 732 (1), 2020, https://doi.org/10.1088/1757-899X/732/1/012051
, Horizontal vortex single chamber hydroturbine ,Rev. Fac. Ing. Univ. Antioquia ,pp 150-162 , 2016
, Artificial Vortex (ArVo) power generation - An innovative micro hydroelectric power generation scheme ,2013 IEEE Global Humanitarian Technology Conference: South Asia Satellite (GHTC-SAS) ,pp 53–57 , 2013, https://doi.org/10.1109/GHTC-SAS.2013.6629888
, Power Conditioning System for a Vortex Turbine based Micro Hydro Power Plant ,2018 3rd IEEE International Conference on Recent Trends in Electronics, Information & Communication Technology (RTEICT) ,pp 2583–2588 , 2018, https://doi.org/10.1109/RTEICT42901.2018.9012262
, Integration of Water and Energy Planning to Promote Sustainability ,J. Sustain. Dev. Energy, Water Environ. Syst , Vol. 7 , 2018, https://doi.org/10.13044/j.sdewes.d6.0246
, - , , The Mechanical Design Process, 2003
- , , Diseño y desarrollo de productos: enfoque multidisciplinario, 2004
Monitoring and control of hydro power plant ,IFAC Proc. Vol , Vol. 40 (8),pp 44-55 , 2007, https://doi.org/10.3182/20070709-3-RO-4910.00007
, Automatic generation control and monitoring the mechanism of micro hydro power plant with impulse turbine and synchronous generator ,2016 2nd International Conference on Robotics and Artificial Intelligence (ICRAI) ,pp 175–179 , 2016, https://doi.org/10.1109/ICRAI.2016.7791249
, - , , Eficiencia en el uso de la Energía Eléctrica, 2010