Wastewater treatment systems earlier were limited to household treatment units and processes in Pluakdang Municipality. The operation and performance of septic tanks, soakaway pits and trenches is problematic, and discharges both of effluent and sludge had their impact on surface and ground waters. There was a need build a more effective and sustainable solution for sewage treatment. Sewer networks can safely collect and transport sewage to a central treatment facility, whereby substantial portions of degradable organic matter, harmful microorganisms, and nutrients are removed from water before discharge to the environment. A central treatment system can be operated, maintained and monitored reliably to meet the local water quality standards and enable certain types of reuse. However, transport of wastewater across boundaries not only affects local residents but also has adverse impact on nearby catchments and ecosystems [1], which have to be assessed and mitigated.
The Sustainable Development Goals by the United Nations call for action to promote prosperity while protecting the planet. At the Rio de Janeiro Earth Summit Rio Summit in 1992, local authorities were called on to communicate and act together with their residents, organisations, and companies on a Local Agenda 21 [2]. The concept of LA 21 is focused on sustainable development planning at the local level. Thailand has adopted the LA 21 plan of action, including the management of fresh water resources. Local government became responsible for the planning of systems for handling wastewater in their area. The introduction phase was very slow, and only 2.39% of the 7,852 Municipalities had implementations [3] in 2015, similarly to many developing countries [4].
Sustainable development planning has evolved to mean how the needs of present communities are met without compromising those of future generations. This has been translated to the three pillars of sustainability: economic, environmental, and social. Assessment and reporting in this manner links to triple bottom line reporting (TBL) [5]. TBL is predicated on a sound platform of knowledge and practice. Its implementation and practice leads to improved assessment and management of risk through a wider spectrum of economic, environmental, and social considerations, genuine proactive communication with key stakeholders, focus on long term economic, environmental, and social values important to local communities, and facilitating bench marking within sectors that consolidate knowledge and experience.
Development of infrastructure traditionally has relied upon assessment and selection with a strong bias towards costs and economic considerations. This process was expedient for additions and modification to capital works to accommodate population growth or provision of improved services in a region. In doing so, it presupposed that environmental and social attitudes and values would be reflected in legislation and policy making or more broadly within the political process that underpin them and the related budget formulation and execution [6]. What is nowadays being questioned is the adequacy of the risk assessments undertaken and whether they facilitate meaningful comparative or sensitivity analysis with methodology and techniques that are currently available and possible. Indeed, the widespread use of TBL in more developed countries, and the tangible benefits that are evident necessitate the transition. This is even more pronounced when external funding is provided by international multi-lateral agencies and donors [7]. Growing environmental concerns brought about by unbalanced development in Thailand necessitate significant and urgent improvements in planning and design processes [8].
The planning and design of wastewater treatment systems are complex processes in many cases, especially for large projects. Plant design necessitates multi-disciplinary technical expertise to find effective and practical solutions that meet the standards. Apart of technical requirements and economic performance, environmental impacts, plant operability and reliability, societal and even political aspects has to be considered in some form of a process known as Multiple-Criteria Decision Analysis (MCDA) [9]. In contrast to TBL that focuses on reporting of economic, social, and environmental gains and losses, MCDA provides a framework to organize information about compromises and trade-offs and, which facilitate a structured design or evaluation process, balanced consideration of alternatives, and leads to a sound and defensible choice by consensus. There are many methods of MCDA has been proposed in the literature that typically involve complex computations. Similarly, there is an abundance of software application, both commercially and freely available that cover various stages of the decision making process, from initial problem exploration to the selection of the final solution. Weistroffer and Li [10] review nearly 70 generic software packages for both multiple criteria design (i.e. optimisation) and multiple criteria evaluation (i.e. decision analysis) methods, indicating their widespread use in various industries. Nevertheless, most local administrative organizations (LAO) in low and lower-middle income countries [11], including Thailand [12] do not have adequate local technical expertise, institutional capacity, and financial resources, to employ sophisticated tools and methods. There are many previous studies that link various aspects, elements and activities of the development process to provide many benefits. Engineering design of wastewater treatment plants is normally carried out using commercial or proprietary software packages and typically using established models taken from the extensive available literature [13]. Linking plant process design to cost estimation databases [14] and computer aided drafting ensures prompt generation of bills of quantities [15] that enable better cost estimations and faster contract preparations. Integration of the design process enables early involvement of potential contractors in projects. Information sharing allows the exchange of ideas and capabilities, finding alternative solutions for details, better scheduling of works, thereby allowing cost and construction time savings [16]. Life Cycle Assessments (LCA) nowadays are mandatory for assessing
Considering the limitations of currently available solutions reported in the literature, herein a comprehensive and fully integrated framework is proposed to match the specific problem at hand, thereby enabling even small Municipalities to manage their relatively small wastewater treatment projects. To be successful, such a system must be simple, practical, and accessible to ensure transparency and accountability, providing a platform to obtain, understand, and incorporate the opinions, values and judgements of all stakeholders [25]. This manuscript presents the implementation and first application of this framework that enabled the relatively rapid and smooth planning, design, and comprehensive assessment of three wastewater treatment plant alternatives with 1,500 m3/day nominal capacity. Although the discussed WWTPs are intended for tropical climate and developing country environment, the insight obtained worth sharing because can also be used in many other settings and circumstances to provide the benefits described.
The initial phase of project focused on establishing and clarifying the needs, requirements and obtaining essential data.
The design capacity of the WWTP was established with regard to existing population number and forecasted growth over 10 + 10 years horizons, including expected commercial and light industrial development in the Municipality. After examining the local geographical, hydrological, and sewerage conditions, discussing preferences and other relevant details with the client, three types of alternative technologies were scoped and accepted for inclusion. One of the initial suggestions (activated sludge treatment) was replaced with a more advanced (and slightly disparate) technology that attained a significant local market share (about 80 working plants) and actively marketed to prospective clients. Discussions with authorities also confirmed the implementation of an artificial wetland system to improve water quality in recipient canal and the nearby Nong Pla Lhai Reservoir located downstream.
For developing country conditions, natural technologies for wastewater treatment such as pond systems, land treatment, and constructed wetlands are especially advantageous [26]. Pond (also called lagoon) systems primarily use physical and biological processes to treat wastewater [27] while land treatment systems utilise soil and plants, without significant need for reactors, labour, energy and chemicals [28]. Typical wetland solutions are constructed filtration systems with defined filter material (gravel and sand with topsoil) that are planted with aquatic vegetation, such as cattails and reeds. In such systems, wastewater flows through the filter material and the treatment is achieved by a combination of chemical, physical, and biological processes [29]. The presence of vegetation improves the treatment efficiency, producing an effluent suitable for various reuse applications like for irrigation of non-alimentary crops [30]. At present, there are many thousands of stabilization ponds and constructed wetlands in operation all over the world, with ability to treat municipal [31], agricultural [32] and industrial [33] wastewaters in diverse climatic conditions [34].
The objective of the project was to design an affordable, robust and sustainable wastewater treatment solution for a small community of peri-urban setting. To accomplish this aim, an integrated design paradigm was used wherein interdependent engineering/scientific (technical), financial (cost), environmental and community engagement aspects provide interactive blocks (modules) for design management.
There are comprehensive (SUMO, BioWin, STOAT, etc.) software simulators available for wastewater treatment technology design. Similarly, life cycle analyses are assisted with tools like GaBi, OpenLCA and supporting cost databases. Such tools have sophisticated features to suit research needs but remain mostly unused for small projects due to input data scarcity in the design stage, and default parameters unsuitable for hot climate conditions. For these reasons, a simple toolset was developed to cater for the project needs. Although initially consideration was given to using engineering packages (MATLAB, Mathcad), for simplicity Microsoft Excel was used. This spreadsheet has a vast and trained user base, and extensive list of features to allow advanced calculations, optimisation, database management (SQL), programmability for workflow automation and team work using workspaces. The next section briefly presents the essential components of this integrated system.
The environmental module is based upon the ISO 14040:2006 Environmental management ‒ Life cycle assessment ‒ Principles and Framework standard with justified simplifications to match the scale and nature of the project.
Figure 1 illustrates a stabilization pond system comprising screening and grit removal (common to all systems), followed by anaerobic pond, facultative pond, and polishing (or maturation) pond units. The second system in Figure 2 is biological contact oxidation (BCO) system, also called hybrid (or integrated) integrated fixed film / activated sludge system. The system-specific units are the contact oxidation ponds or tanks. In essence, the specific BCO technology uses compressed air and submerged diffusors for aeration, and plastic media carrier elements for accommodating biofilm.
Stabilization pond system
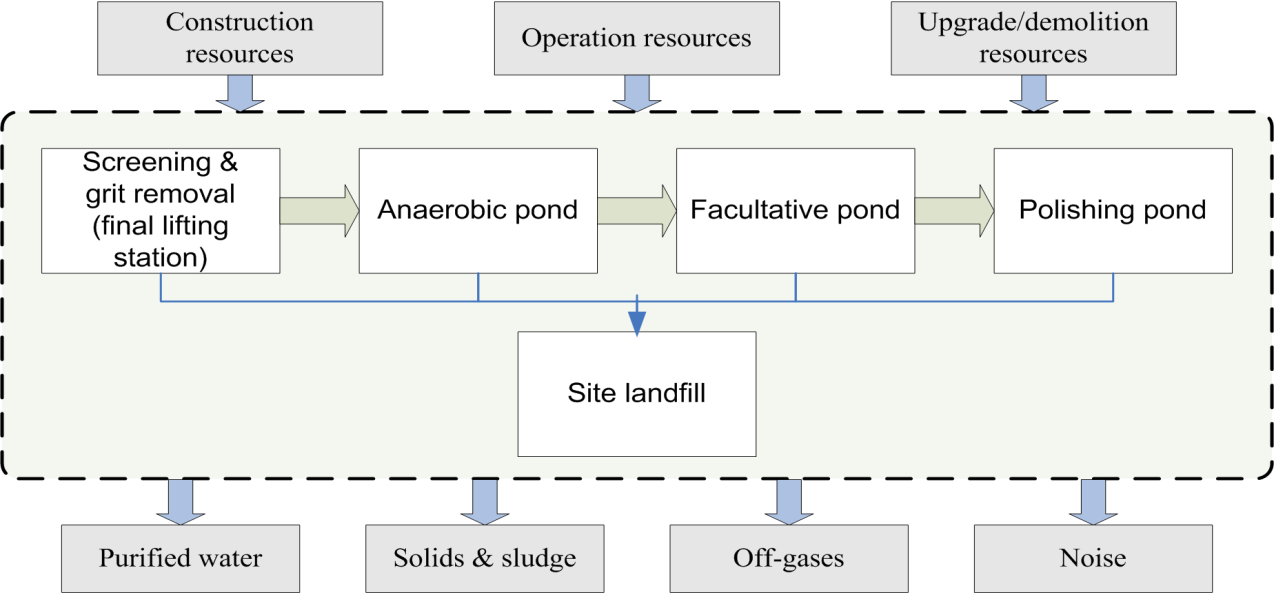
Biological contact oxidation system
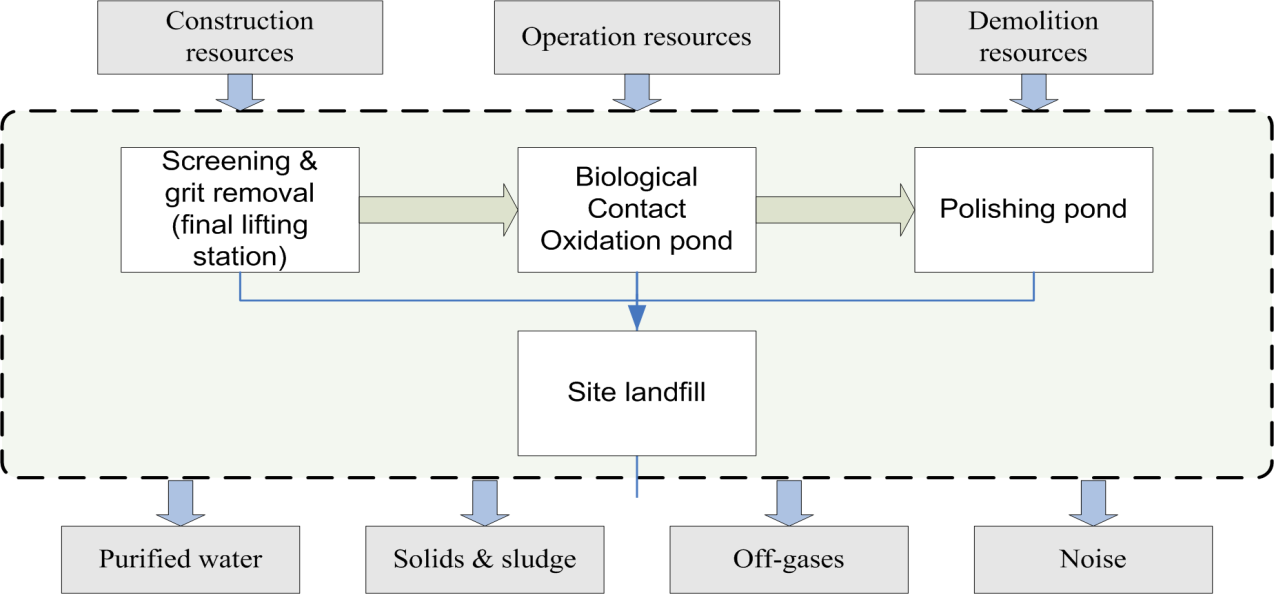
The aerated pond system is not illustrated here because it is very similar to the stabilization pond system. The essential difference is that one or more ponds – except the polishing pond(s) ‒ are aerated, typically using surface aerators with draft tubes supported by floating pontoons. Due to the artificial aeration system used in aerobic units, such systems have reduced footprint and/or provide better quality effluents than stabilization ponds.
The set of criteria for assessing the alternative treatment systems is presented in Table 1.
Set of indicators used in this study
Criteria |
Indicator |
Unit |
Economic |
Capital costs Operational/maintenance costs User costs |
[USD/m3 day] [USD/m3 day] [USD/m3 day] |
Environmental & technical |
Energy use GHG emission Biochemical oxygen demand (BOD) Suspended solids (SS) emission Ammonia (NH4-N) emission Total Kjeldahl nitrogen (TKN) emission Specific plant footprint Operating skill |
[kWh/m3] [g/m3] [g/m3] [g/m3] [g/m3] [g/m3] [m2/m3 day] Operator grade |
Societal |
Public participation Performance reports & training |
Qualitative measure [h/year] |
Most of these indicators are obtained directly from linked technical sheets and required for assessing and ranking the impacts of the alternative technologies.
This module handles fundamental data, such as effluent water quality standards, raw wastewater and effluent parameters, and core engineering tasks like unit sizing and modelling for CAD, printing, and dashboard summaries.
For statistical data collection a traditional multi-stage approach with non-probability and purposive sampling [35] was used. It involved 100 randomly selected households/persons for survey and in-depth interviews. The interviews also provided a good opportunity to share information about the impending project.
The correct establishment of design water quality parameters was critically important. Table 2 shows the characteristics of influent wastewater adapted for design together with statutory effluent limits, and the minimum degree of required treatment.
Influent and effluent parameters for design
Parameter |
Influent |
Effluent limit |
Removal required |
BOD5 [mg/L] |
100 |
20 |
80% |
COD [mg/L) |
140 |
N/A |
N/A |
NH4-N [mg/L] |
12 |
N/A |
N/A |
NO3-N [mg/L] |
1 |
N/A |
N/A |
TKN [mg/L] |
25 |
15 |
40% |
TP [mg/L] |
3 |
N/A |
N/A |
SS [mg/L] |
80 |
30 |
63% |
Oil & grease [mg/L] |
3 |
5 |
N/A |
pH |
7‒8 |
6‒9 |
N/A |
The design values were partly based on data analysis obtained from similar plants, and mainly on 10 hour wastewater samples. Spot sampling was carried out a day per week over a 6 week period at two pumping stations in dry weather conditions. Duplicate samples were collected 10 hours apart during daytime, i.e. 4 samples per day. The analysed parameters did not change much over the collection period. BOD5 values were in the range of 60 to 80 mg/L, COD between 100 and 120 mg/L, NH4-N between 10 and 12 mg/L, TKN between 18 and 20 mg/L while SS did not exceed 80 mg/L. However, tests results show that in the rainy season BOD5 values may be lower, in the range 30 to 60 mg/L, while COD below 100 mg/L. The samples were stored on ice and analysed in laboratory following the pertinent Standard Methods [36]. In addition, extended BOD values over 14 days with and without nitrification inhibition and oxygen uptake rates (OUR) were measured with Hanna HI5421 DO/BOD meter. These results enabled the derivation of kinetic rates, and comparing several design procedures in process calculations. The influent values for design incorporate an average safety factor of 1.2 due to uncertainties in current and future data. Regarding the absent parameters in Table 2, Thailand currently has a set of fragmented, sparse, and inconsistent legislation regarding wastewater management [37]. Water quality is monitored in recipients but untreated discharges are rampant.
For planning and preliminary design cost estimations USEPA’s methodology [38] [39] provides useful guidance that can be implemented in spreadsheets. A modernised and updated version of the CapdetWorks is available from Hydromantis Inc. This application offers an interactive user interface that simplifies the construction and evaluation of multiple treatment plant layouts. It can be used for assessing large-scale activated sludge plant [40] or small plants (Figure 3) versions but international users must provide local costing data to ensure reasonably accurate estimates.
The annual O&M costs include energy consumption, labour, maintenance repair and replacement (1%‒2% of equipment capital costs), and chemical costs. Options for solids disposal should be carefully analysed. Screenings and grit represent only a small proportion of the total and often can be disposed at sanitary landfills economically. However, sewage sludge disposal poses a greater challenge, and thus local storage and treatment, or utilization in agriculture should be carefully examined.
Aerated pond treatment system in CapdetWorks
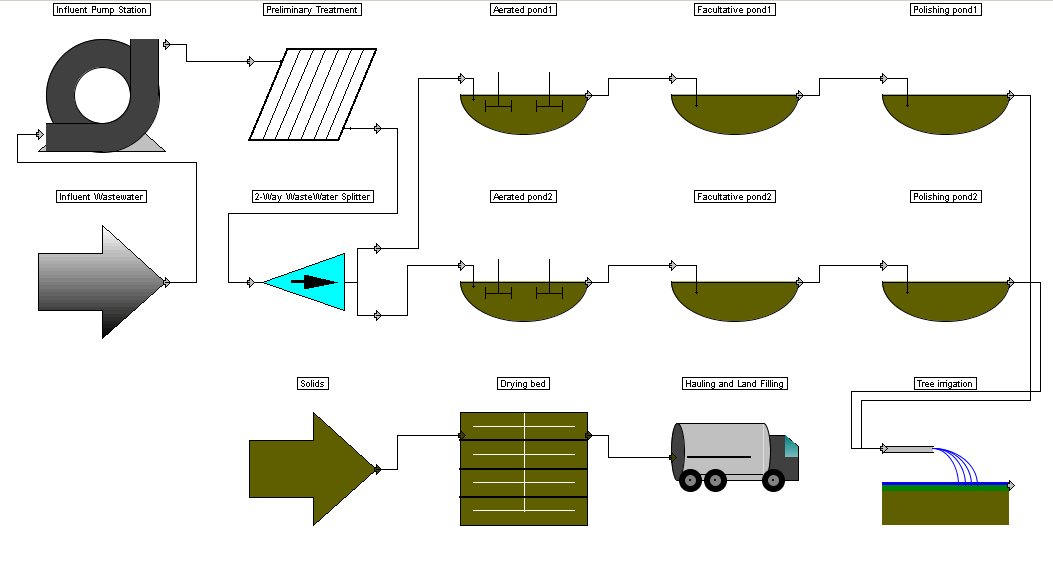
Kasemsawat et al. found [41] that the main reasons behind inadequate community involvement are limited awareness and lack of initiatives, which can be remedied by community education. Therefore, this module focuses on producing community educational slides about environmental issues, progress updates, and survey/feedback materials to keep residents informed and motivate their active participation. In the initial stage it is helpful to engage in community outreach, and organise a workshop to introduce the project to learn the perception of the residents. The main aim of the module was to achieve active participatory involvement of residents in the formulation of the plans and approval of the development. Local communities are not mere spectators but drivers of development, and thus their ability to effectively manage affairs and risks should be supported by transparency, data and knowledge sharing, and cooperation with local administrative organizations and academic institutions. The local culture is characterised by avoidance of confrontations and willingness to make compromises. These traits are helpful in the reconciliation of the differing interest of stakeholders, including the ecosystem.
This section presents the major results specific to the present project and discusses selected findings of wider interest and applicability.
The results shown in Table 3 are pollutant emissions for peak load and non-ideal conditions that can be regarded a worse-case scenario for normal operating conditions, i.e. excluding major plant failure events.
Summary of water pollutant inventory b64{PHhyZWYgcmVmLXR5cGU9ImJpYnIiIHJpZD0iYmlia2cvZGF5Ij5ba2cvZGF5XTwveHJlZj4=} for 1500 m3/day plant load
Parameter |
Input |
Output Stabilization ponds |
Output Contact oxidation |
Output Aerated lagoons |
BOD5 |
150 |
15 |
8 |
8 |
SS |
120 |
12 |
12 |
12 |
NH4-N |
18 |
7 |
2 |
2 |
TKN |
38 |
15 |
9 |
11 |
The final similar values (using the statutory method of unfiltered samples) reflect the fact that final effluent BOD is controlled by algal concentrations (i.e. BOD load in discharge) in polishing ponds. Filtered effluent samples reveal that BCO systems have slightly better performance than aerated ponds in terms of dissolved BOD removal. The comparable nitrogen removal efficiencies show that denitrification is limited due to low organic carbon content in the raw sewage. It can be seen that the project substantially reduces the current aquatic pollutant burden on the ecosystem, and the differences among the alternatives are relatively minor.
Stabilization pond, aerated lagoon, and biological contact oxidation systems including auxiliary facilities (control/guard room, lime store, repair shop, transformer station, fencing, lightning, and temporary grit storage) were selected as alternative treatment technologies. The net available (fenced) land area is 5,440 m2 and is owned by the Municipality. The specific capital costs were calculated for the nominal peak design inflow of 1,500 m3/day. The biological contact oxidation system had the highest cost of about 1,048 USD/m3 day, or 90.31 USD/capita. The aerated pond system had a slightly smaller cost of 940 USD/m3 day, while the least costly was the stabilization ponds system at about 741 USD/m3 day. Since each alternative system can safely meet the current statutory pollutant discharge requirements, it could be inferred that these cost data would likely rank the order of preference for governmental funding.
The O&M cost included labour, chemical, repair & replacement of equipment, basic laboratory services and energy costs. Stabilization ponds rely on natural treatment processes, and thus have the lowest O&M cost of 0.05 USD/m3 treated wastewater. Aerated lagoons were more expensive and operate at 0.116 USD/m3 due to the energy cost for aeration, machinery maintenance requirements, and higher operating skills/labour costs. The more advanced biological contact oxidation has the highest O&M costs, about 0.147 USD/m3. In this case the energy cost of aeration is about 25% smaller than of aerated ponds (due to more efficient fine bubble aeration system), but substantial extra cost was created by the pumped recirculation illustrated in Figure 4.
Line diagram of the BCO system
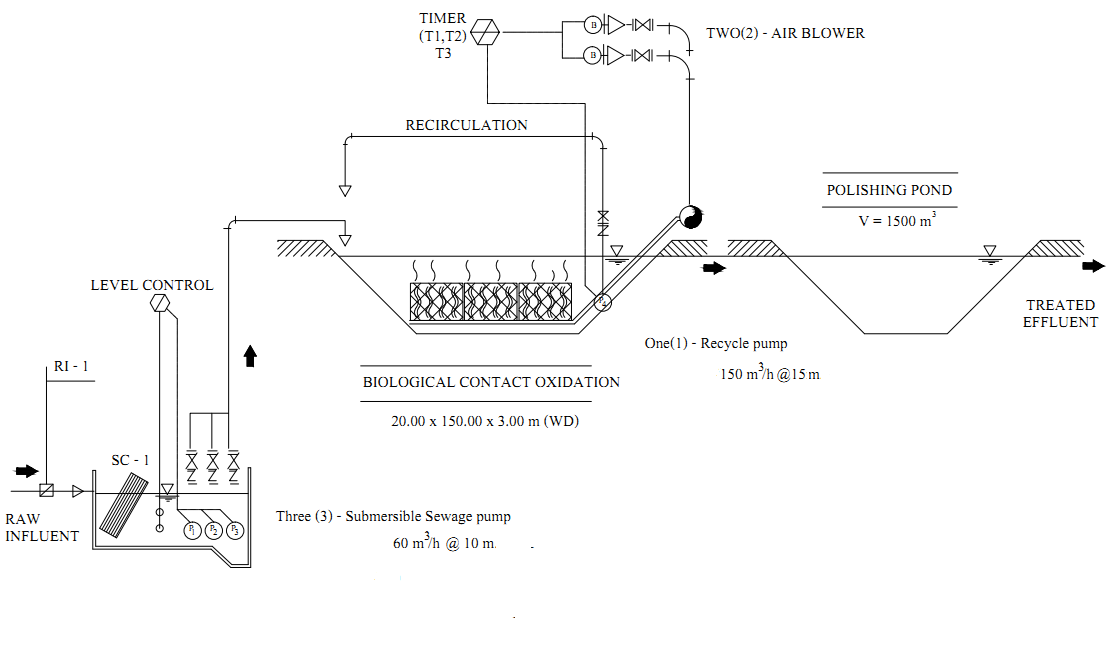
These findings also reveal that stricter effluent quality standards demanding nutrient removal would have only a relatively small effect on capital costs. However the O&M costs would increase substantially.
This is related to the critical cost element for community acceptance and willingness to pay, hence sustainability of the WWTP. In line with Government policy the Municipality had to fund the O&M costs by charging users according to the user pay principle [42]. A wastewater collection and treatment fee is based upon metered water supply, using a 0.80 volume conversion factor to estimate sewage discharge. Accordingly, for stabilization pond treatment users will be charged at 0.05 USD/m3 (1.50 THB/m3) rate, which is quite reasonable for a small system. For comparison, the wastewater charge introduced in Bangkok in 2017 is 25% higher, generally using activated sludge treatment technologies but benefiting from the effect of scale. Table 4 provides income data obtained by statistical survey in the serviced area during the initial phase of design, and confirms affordability.
Survey of household revenue
Total household revenue per month |
Count |
|
THB |
USD |
|
< 9,000 |
< 275 |
7 |
9,001‒15,000 |
276–450 |
25 |
15,001‒20,000 |
451–613 |
24 |
20,001‒30,000 |
614 – 919 |
12 |
30,001‒50,000 |
920‒1,532 |
19 |
50,001‒70,000 |
1,533‒2,146 |
7 |
70,001‒90,000 |
2,147‒2,759 |
1 |
90,001‒110,000 |
2,600‒3,372 |
0 |
110,001‒130,000 |
3,373‒3,985 |
3 |
130,001‒150,000 |
3,986‒4,598 |
1 |
> 150,000 |
> 4,599 |
1 |
Total |
100 |
Overall, the results show that adequate wastewater treatment can be implemented at low capital and operating costs using stabilization pond technology to provide tangible benefits and improvement to the environment and the community.
For the final preparative stage of design the Municipality organised an open community meeting (Figure 5).
Preparation of public meeting
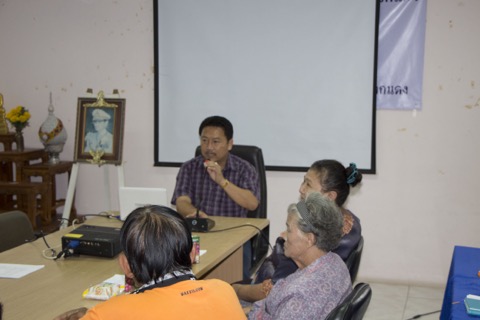
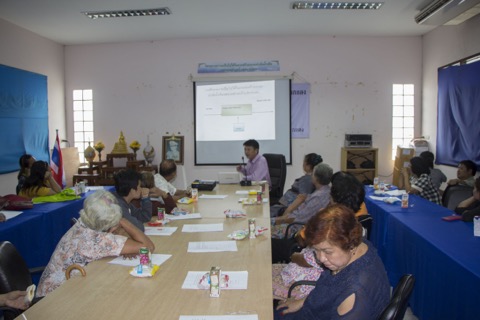
The introductory presentation covered project aims and outcomes, alternative solutions, budgets, implementation phases, and expected construction schedules. The subsequent discussion followed a forum format with questions asked and answered.
The residents were aware of, and readily accepted the user pay policy. The charging method (calculated after water use) and the modest charge was received favourably. The Municipality provides support/subsidy to those in financial need to have adequate water and wastewater services as dictated by social policy and public health interests. The charging method, however, needs to be refined to include users relying on bore well and rainwater supply, and therefore do not receive water bills used for sewage charges.
Concerns regarding plant operation, maintenance and required operator skills were also raised, with the view that simpler solutions should be preferred.
It was suggested to utilise the polishing ponds of the plant for “koi” fish (ornamental carp) breeding. This was not recommended since effective disinfection in polishing ponds requires deep sunlight penetration. Breeding small bait fish (minnow, etc.) is acceptable since these are not bottom-dwellers that risk making water murky and consume algae.
Possible odour emission, noise and mosquito nuisances also caused anxiety. This problem was alleviated by locating the plant away for populated areas and using a buffer zone for (Figure 6). Odour emission from primary treatment (screening), sludge processing, and sludge disposal will be minimised by using mobile industrial containers and applying sealing lime/sand layers on drying beds as standard operational procedure.
Nuisance reduction by plant location and buffer zone
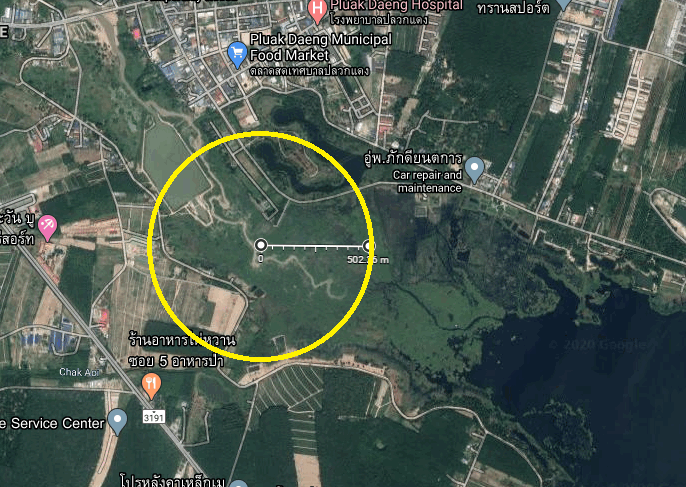
Voting prior to the closure of the meeting showed 96% approval rate for implementing the WWTP project. For the proposed plant variants, 71% preferred facultative pond, 15% aerated lagoon, 8% BCO systems, with 6% abstaining. Therefore, the meeting showed strong public support for implementing the project, and preferably the facultative pond treatment system variant.
The last section present some observations and lessons learned in the course of design. Sharing such information may be useful to readers involved with the planning, design, operation, and monitoring of sewage treatment works.
Boundary selection. The selection of system boundaries has a profound effect on LCA results. For example, the environmental costs vary significantly whether or not the impact of sludge management is allocated to on-site or external sinks like landfills. Similarly, the construction of sewer infrastructures usually has an environmental impact larger than both the construction and operation of the WWTP [26] but might be excluded from the scope of the study.
Energy use. Coupling wastewater collection and treatment systems, the highest portion (about 80%) of applied electric energy is required for pumping during wastewater conveyance [43]. Considering only treatment, for activated sludge treatment technology pumping absorbs approximately 50% of the total electrical energy, while about 10% and 40% is required for primary treatment, and aeration, respectively [44]. In the present study, energy input was the most substantial contributing factor to operational and maintenance costs of the aerated pond and BCO systems. Over 90% of energy use was attributed to electricity consumption, and the rest is fuel cost of service vehicles and machinery. Aerated ponds do not use recirculation, albeit this might be a useful means to increase treatment capacities for overloaded systems. In relevant studies Middlebrooks et al. found [45] [46] that land treatment and aerated lagoon treatment systems have 2.5‒3 times smaller energy cost than conventional activated sludge plants for comparable quality effluents. However, aeration of lagoon systems increased energy use by 3 times for 380 m3/day and 5 times for 18,900 m3/day plant capacities. These results corroborate the present finding, and explain the significantly higher O&M costs of aerated lagoon system vs. facultative ponds. Moreover, this finding implies that life cost analyses can be simplified to consider only the plant operational phase and electric energy use.
Process design. Biological wastewater treatment theory and practice has a vast literature. Process calculations do not pose challenges since these are thoroughly presented and discussed in many handbooks. For pond treatment design in particular there are excellent materials available [47] [48] [49]. Herein only some aspects are highlighted that have profound effects on the performance and costs of plants. Many older treatment plants using activated sludge treatment technology show poor performance in Thailand due to poor design that ignored the effects of sewage temperature on kinetic rates and oxygen transfer, and long detention times in the collection system [50]. Typical BOD5 values prior to discharge in Bangkok are only around 40 mg/L [51]. For a smaller(30,000 population) provincial centre a safe design value of 80 mg/L was recommended, or 60 mg/L for a plant located further away with an extra 5 hours detention time in the transfer pipeline [52]. In other words, the collection system provides a substantial degree of anaerobic pre-treatment with profound effects on wastewater composition. In this sense, the purpose of the WTTP in such cases is to provide post-treatment to low-strength anaerobic effluents that recently opened up interesting directions in research [53]. The same observation may lead to conclusions that with substantial anaerobic pre-treatment there is no need for consecutive treatment in anaerobic ponds. Such views are quite agreeable; however such ponds also provide efficient sludge treatment. Due to the slower but more complete degradation, the mass of residual sludge is 5‒8 times less than in aerobic treatment [54]. Therefore, properly sized anaerobic ponds can eliminate the need for further sludge treatment and disposal elsewhere, while also allowing potential sludge reuse as liquid fertilizer.
Nutrient removal. The rapid and inevitable removal of readily biodegradable substrates in the collection system leads to low C:N ratios to make biological nitrogen removal difficult. Pond treatment systems provide primarily secondary treatment for carbon removal but also reduce nutrient levels in effluents. Inorganic/mineralised nitrogen is removed by direct algal uptake of ammonium ion, and out-gassing of ammonia to provide up to 50%‒55% reduction rates in tropical conditions. Algal activity during daytime raises the pH (coincidentally providing effective disinfection) that shifts the ionic equilibrium towards the gaseous form. Phosphorous removal also involves two mechanisms, microbial uptake and chemical precipitation. Phosphorus uptake is limited since the P content of microorganisms is much lower than of N. For facultative ponds the phosphorus uptake is about 1 to 3 mg/L [55]. Thailand banned the use of detergents with phosphorous additives [56]. Typical P concentrations in sewage are low compared to developed countries [57] and far below the level given (only) for discharge from housing estates.
Operational robustness. The BCO system of the present study combines aerated and unaerated zones in a single reactor unit [58] for effective nutrient removal as illustrated in Figure 7. This reduces the reactor footprint but treatment efficiency is dependent upon recirculation. Equipment fault or power blackouts can have serious consequences and with sludge and biofilm degradation. Aerated lagoons are more resilient since operate as stabilization ponds in a "fallback mode" after a temporary process upset. The simplest alternative (stabilization ponds) is inherently robust because it utilises oxygen provided by natural diffusion and algal photosynthesis.
Aerobic and anoxic zones in biological contact oxidation units
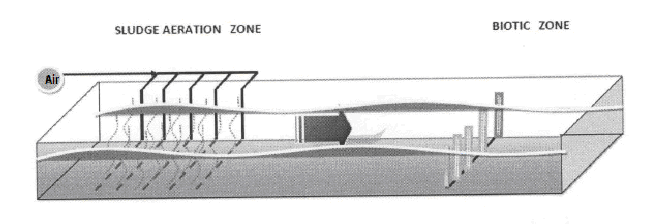
Plant expandability. It is prudent to assume that plant loads might increase as a consequence of unexpected population growth and/or industrial development. Besides, there is a possibility of stricter effluent quality standards in the future. Would such need arise, stabilization pond treatment can be simply augmented for carbon removal by installing surface aerators in the facultative ponds. Nutrient removal can be improved significantly by addition of slaked lime. Dosing at low rates (20‒80 g/m3, depending on water hardness) can achieve effective reduction of phosphorous, nitrogen, and algae levels [55]. Another possibility is the construction of wetland (as in the present case) or land-based treatment systems for post-treatment. For the latter, a good choice is reuse by irrigation.
Effluent reuse. Wastewater utilisation not only can reduce environmental impacts but also decrease the operating costs [59]. In this case the local climatic conditions would allow 6‒7 months effluent utilization in dry months by irrigation, effectively halving the annual pollutant load. Pond effluents should not be used for irrigation of alimentary crops [60] because despite efficient reduction of bacteria [61], helminth egg [62] and virus removals [63] are limited. Irrigation of prized tropical tree plantations such as teak [65] and mahogany [66] can generate significant profits. This opportunity was not in the current scope, and its feasibility shall be examined in a consecutive study.
Community engagement. Residents only can provide feedback in merit if sufficient and clear information about the planned project is given. The successful community meeting showed that it is possible to convey bottom-line results with technical terminology explained in glossaries. A sample report used for the comparison of alternative treatment solutions at the forum is shown in Table 5. Various features are compared to give qualitative (relative) ranks. Forum participants use their own weight/importance factors to score and identify the system variant preferred. Tariffs are quantitative criteria and treated separately. Users pay for O&M costs only, hence the units fees and mode, ranging from 0.05 USD/m3 to 0.147 USD/m3 for the different treatment systems. In addition, Government policy for small/disadvantaged communities also allows for a flat monthly rate of 1.59 USD (50 THB) per household. In fact, tariff seemed to be a less important issue for participants than other aspects, for example possible nuisances. The stabilization pond option has the highest land use and highest odour emission to affect the nearby community. It was recognised that the BCO system has the best technical performance but that also the highest costs and requiring skilled operation and maintenance.
Relative preference ranking of system features for community discussion; more stars indicate higher/better performances
Criteria |
Stabilization pond system |
Aerated lagoon system |
BCO system |
BOD5 removal |
* |
** |
*** |
Nitrogen removal |
* |
** |
*** |
Sludge formation |
** |
* |
*** |
Nuisance/odour |
* |
** |
*** |
GHG emission |
* |
*** |
** |
Land use |
* |
*** |
** |
Capital costs |
*** |
** |
* |
O&M costs |
*** |
** |
* |
Robustness |
*** |
** |
* |
Supervision |
*** |
** |
* |
Many Municipalities in Thailand face with new challenges to provide affordable and efficient wastewater treatment services for their residents. The solutions have to be planned, designed, implemented, and sustained locally using limited resources. The process inevitably affects the broader environment, and crosses various boundaries among organizations, areas of knowledge, and responsibilities that create additional constraints.
The presented case study explored the complex relationship between limited funding, anachronistic legislation, community expectations, and restricted technical possibilities to provide wastewater treatment with 1,500 m3/day capacity for a peri-urban community. Three alternative technologies were assessed for the treatment of communal wastewater to find the most agreeable and satisfactory solution. The version eventually accepted for implementation was the oxidation pond system, which is the simplest, least expensive and sustainable choice.
The integrated design management framework developed for this (and similar) projects proved to be an effective tool. It helped learning new skills and competences, facilitated inclusive thinking, and ensured the appreciation of possible effects both on local and wider environments over a longer term.
The authors gratefully thank Pluakdaeng Municipality and Suan Sunandha Rajabhat University for their support.
- Muga, H. E. and Mihelcic, J. R., Sustainability of Wastewater Treatment Technologies, J. Environ. Manage., Vol. 88, No. 3, pp 437-447, 2008, https://doi.org/10.1016/j.jenvman.2007.03.008
- United Nations Division for Sustainable Development, United Nations Conference on Environment and Development, Rio Declaration, Agenda 21, Forest Principles, Report, A/CONF.151/26/Rev.l (Vol. l), New York, USA, 1992.
- Leknoi, U., Analysis of the Critical Success Factors that Affect the Implementation of Local Agenda 21 in Thailand, Int. Bud. Stud., Vol. 8, No. 1, pp 82-102, 2017.
- Xavier, L. Y., Jacobi, P. R. and Turra A., Local Agenda 21: Planning for the Future, Changing Today, Environ. Sci. Policy, Vol. 101, pp 7-15, 2019, https://doi.org/10.1016/j.envsci.2019.07.006
- Murray, K., Sustainability Reporting - Beyond TBL?, Solutions, Vol. 10, No. 2, 2019.
- Department of the Environment and Heritage, Triple Bottom Line Reporting in Australia: A Guide to Reporting Against Environmental Indicators, Environment Australia, Canberra, Australia, 2003.
- Petrova, K., Sinclair, R. and Kwan, C-T., Risk Assurance for Triple-Bottom Line Reporting using SoDIS, Proceedings of the 2004 IT Governance Conference, Auckland, New Zealand, pp 59-68, 2004.
- Office of the National Economic and Social Development Board (NESDB), The Twelfth National Economic and Social Development Plan (2017-2021), Office of the Prime Minister, Bangkok, Thailand, 2017.
- Norese, M.F., Decision Aid in Public Administration: From Evidence-Based Decision Making to Organizational Learning, in: Multiple Criteria Decision Making: Applications in Management and Engineering, (Zopounidis, C. and Doumpos, M., eds.), pp 1-29, Springer International Publishing, Switzerland, 2017.
- Weistroffer, H. R. and Li Y., Multiple Criteria Decision Analysis Software, in: Multiple Criteria Decision Analysis: State of the Art Surveys, (Greco, S., Ehrogott, M. and Figueira, J., eds.), pp 1301-1338, Springer Science, New York, USA, 2016.
- Cossio, C., et al., Indicators for Sustainability Assessment of Small-scale Wastewater Treatment Plants in Low and Lower-middle Income Countries, Environ. Sustain. Ind., Vol. 6, 100028, 2020, https://doi.org/10.1016/j.indic.2020.100028
- Rammont, L. and Amin, A. T. M. N., Constraints in Using Economic Instruments in Developing Countries: Some Evidence from Thailand's Experience in Wastewater Management, Habitat Int., Vol. 34, No. 1, pp 28-37, 2010, https://doi.org/10.1016/j.habitatint.2009.05.003
- Olukanni, O. D., Hydraulic Modeling and Optimization of Waste Stabilization Pond Design for Developing Nations, PhD Thesis, Civil Eng. Dept., Covenant University, Ota, Nigeria, 2011.
- Office of the Chief of Engineers (Army), Computer-Assisted Procedure for the Design and Evaluation of Wastewater Treatment Systems (CAPDET) - Programmer's Reference Manual, USEPA, Washington D.C., USA, 1982.
- Li, X.-W., Computer-Aided Design for Stabilization Ponds, Water Sci. Technol., Vol. 26, No. 9-11, pp 2425-2428, 1992, https://doi.org/10.2166/wst.1992.0753
- Wondimu, P.A., Hailemichael, E., Hosseini, A., Lohne, J., Torp, O. and Lædre, O., Success Factors for Early Contractor Involvement (ECI) in Public Infrastructure Projects, Ener. Procedia, Vol. 96, pp 845-854, 2016, https://doi.org/10.1016/j.egypro.2016.09.146
- Piao, W., Kim, Y., Kim, H., Kim, M. and Kim, C., Life Cycle Assessment and Economic Efficiency Analysis of Integrated Management of Wastewater Treatment Plants, J. Clean. Prod., Vol. 113, pp 325-337, 2016, https://doi.org/10.1016/j.jclepro.2015.11.012
- Corominas, L., Foley, J., Guest, J. S., Hospido, A., Larsen, H. F., Morera, S., and Shaw, A., Life cycle Assessment Applied to Wastewater Treatment: State of the Art, Water Res., Vol. 47, No. 15, pp 5480-5492, 2013, https://doi.org/10.1016/j.watres.2013.06.049
- Kalbar, P.P., Karmakar, S. and Asolekar, S.R., Life Cycle-Based Decision Support Tool for Selection of Wastewater Treatment Alternatives, J .Clean. Prod., Vol. 117, pp 64-72, 2016, https://doi.org/10.1016/j.jclepro.2016.01.036
- Castillo, A., Cheali, P., Gómez, V., Comas, J., Poch, M. and Sin, G., An Integrated Knowledge-based and Optimization Tool for the Sustainable Selection of Wastewater Treatment Process Concepts, Environ. Modell. Software, Vol. 84, pp 177-192, 2016, https://doi.org/10.1016/j.envsoft.2016.06.019
- Economopoulou, M.A. and Economopoulos, A. P., Expert System for Municipal Wastewater Management with Emphasis in Reuse, Water Supp., Vol. 3, No. 4, pp 79-88, 2003, https://doi.org/10.2166/ws.2003.0048
- Ullah, A., Hussain, S., Wasim, A. and Jahanzaib, M., Development of a Decision Support System for the Selection of Wastewater Treatment Technologies, Sci. Total. Environ., Vol. 731, 139158, 2020, https://doi.org/10.1016/j.scitotenv.2020.139158
- Horne, A., Szemis, J. M., Kaur, S., Webb, J. A., Stewardson, M. J., Costa, A. and Boland, N., Optimization Tools for Environmental Water Decisions: A Review of Strengths, Weaknesses, and Opportunities to Improve Adoption, Environ. Modell. Software, Vol. 84: pp 326-338, 2016, https://doi.org/10.1016/j.envsoft.2016.06.028
- Hurlimann, A. and Dolnicar, S., When Public Opposition Defeats Alternative Water Projects – The Case of Toowoomba Australia, Water Res., Vol. 44, No. 1, pp 287-297, 2010, https://doi.org/10.1016/j.watres.2009.09.020
- Tsoukias, A., Montibeller, G., Lucertini, G. and Belton, V., Policy Analytics: An Agenda for Research and Practice, Euro J. Decis. Proc., Vol. 1., pp 115-134, 2013, https://doi.org/10.1007/s40070-013-0008-3
- Metcalf and Eddy Inc., Tchobanoglous, G., Burton, F. L., Tsuchihashi, R. and Stensel, H. D., Wastewater Engineering: Treatment and Resource Recovery, McGraw-Hill Professional, Boston, USA, 2013.
- von Sperling, M., Waste Stabilisation Ponds, Biological Wastewater Treatment, Vol. 3., IWA Publishing, London, UK, 2007.
- Reed, S. C., Crites, R. W. and Middlebrooks, E. J., Natural Systems for Waste Management and Treatment, McGraw-Hill, New York, USA, 1995.
- Rozkošný, M., Kriška, M., Šalek, J., Bodík, I. and Istenič, D., Natural Technologies of Wastewater Treatment, Global Water Partnership Central and Eastern Europe, 2014.
- Lombard-Latune, R., Pelus, L., Fina, N., L'Etang, F., Le Guennec, B., and Molle, P., Resilience and Reliability of Compact Vertical-Flow Treatment Wetlands Designed for Tropical Climates, Sci. Total Environ., Vol. 642, pp 208-215, 2018, https://doi.org/10.1016/j.scitotenv.2018.06.036
- Garfí, M., Pedescoll, A., Bécares, E., Hijosa-Valsero, M., Sidrach-Cardona, R. and García, J., Effect of Climatic Conditions, Season and Wastewater Quality on Contaminant Removal Efficiency of Two Experimental Constructed Wetlands in Different Regions of Spain, Sci. Total Environ., Vol. 437, pp 61-67, 2012, https://doi.org/10.1016/j.scitotenv.2012.07.087
- Pena, L., Oliveira, M., Fragoso, R. and Duarte, E., Potential of Duckweed for Swine Wastewater Nutrient Removal and Biomass Valorisation through Anaerobic Co-Digestion, JSDEWES, Vol. 5, No. 2, pp 127-138, 2017, https://dx.doi.org/10.13044/j.sdewes.d5.0137
- Frankin, R. J., Full-Scale Experiences with Anaerobic Treatment of Industrial Wastewater, Water Sci. Technol., Vol. 44, No. 8, pp 1-6, 2001, https://doi.org/10.2166/wst.2001.0451
- Recio-Garrido, D., Kleiner, Y., Colombo, A. and Tartakovsky, B., Dynamic Model of a Municipal Wastewater Stabilization Pond in the Arctic, Water Res., Vol. 144, pp 444-453, 2018, https://doi.org/10.1016/j.watres.2018.07.052
- Yamane, T., Statistics: An Introductory Analysis, Harper and Row, New York, USA, 1967.
- American Public Health Association (APHA), Standard Methods for the Examination of Water and Wastewater, Washington D.C., USA, 2005.
- Yoochatchaval, W., Regulations and Standards on Decentralized Domestic Wastewater Treatment in Thailand, The 5th Workshop on Decentralized Domestic Wastewater Treatment in Asia, Yangoon, Myanmar, December 12, 2017.
- Tinkham, L. S. and Green, J., CAPDET (Computer Assisted Procedures for the Design and Evaluation of Wastewater Treatment Systems), USEPA, Washington D.C., USA, 1981.
- Harris, W. R., Jr. Cullinane, M. J. and Sun, T. P., Process Design and Cost Estimating Algorithms for the Computer Assisted Procedure for Design and Evaluation of Wastewater Treatment Systems (CAPDET), USEPA, Washington D.C., USA, 1982.
- Jafarinejad, S., Cost Estimation and Economical Evaluation of Three Configurations of Activated Sludge Process for a Wastewater Treatment Plant (WWTP) using Simulation, Appl. Water Sci., Vol. 7, No. 5, pp 2513-2521, 2017, http://doi.org/10.1007/s13201-016-0446-8
- Kaseamsawat, S., Choo–in, S., Utaraskul, T., and Chuangyham, A., Factors Influencing Water Quality of Kwae-Om Canal, Samut Songkram Province, Procedia - Soc. Behav. Sci., Vol. 197, pp 916-921, 2015, http://doi.org/10.1016/j.sbspro.2015.07.273
- Simachaya, W., Wastewater Tariffs in Thailand, Ocean Coast. Manage., Vol. 52, No. 7, pp 378-382, 2009, http://doi.org/10.1016/j.ocecoaman.2009.04.012
- Risch, E., Gutierrez, O., Roux, P., Boutin, C. and Corominas, L., Life Cycle Assessment of Urban Wastewater Systems: Quantifying the Relative Contribution of Sewer Systems, Water Res., Vol. 77, pp 35-48, 2015, http://doi.org/10.1016/j.watres.2015.03.006
- Singh, P., Carliell-Marquet, C. and Kansal, A., Energy Pattern Analysis of a Wastewater Treatment Plant, Appl. Water Sci., Vol. 2, 2012, pp 221-226, http://doi.org/10.1007/s13201-012-0040-7
- Middlebrooks, E. J., Middlebrooks, C. H. and Reed, S. C., Energy Requirements for Small Wastewater Treatment Systems, J. Water Pollut. Cont. Fed., Vol. 53, No. 7, pp 1172-1198, 1981.
- Middlebrooks, E. J., Upgrading Pond Effluents: An Overview, Water Sci. Technol., Vol. 31, No. 12, pp 353-368, 1995, http://doi.org/10.2166/wst.1995.0504
- Shilton, A., Pond Treatment Technology, Vol. 5. IWA Publishing, London, UK, 2006.
- von Sperling, M. and Chernicharo, A. D. L. C., Biological Wastewater Treatment in Warm Climate Regions, IWA Publishing, London, UK, 2005.
- Mara, D., Waste Stabilization Ponds: Past, Present and Future, Desalin. Water Treat., Vol. 4, No. 1-3, pp 85-88, 2009, http://doi.org/10.5004/dwt.2009.359
- Jinjaruk, T., Chunkao, K., Pongput, K., Choeihom, C., Pattamapitoon, T., Wararam, W., Thaipakdee, S., Srichomphu, M. and Maskulrath, P., HDPE Pipeline Length for Conditioning Anaerobic Process to Decrease BOD in Municipal Wastewater, EnvironmentAsia, Vol. 11, No. 1, pp 31-44, 2018, http://doi.org/10.14456/ea.2018.3
- Prateep Na Talang, R., Sirivithayapakorn, S. and Polruang, S., et al., Environmental Impacts and Cost-Effectiveness of Thailand’s Centralized Municipal Wastewater Treatment Plants with Different Nutrient Removal Processes, J. Clean Prod., Vol. 256, 120433, 2020, http://doi.org/10.1016/j.jclepro.2020.120433
- Jinjaruk, T., Maskulrath, P., Choeihom, C. and Chunkao, K., The Appropriate Biochemical Oxygen Demand Concentration for Designing Domestic Wastewater Treatment Plant, EnvironmentAsia, Vol. 12, No. 1, pp 162-168, 2019, http://doi.org/10.14456/ea.2019.18
- Yudachev, V. and Lew, B., Trends and perspectives of anaerobic technologies in the face of stringent disposal standards, in: Post Treatments of Anaerobically Treated Effluents, (Tyagi, V. K., Khan, A. A., Jern, N. W., Khursheed, A. and Kazmi, A. A., eds.), pp 133-149, IWA Publishing, London, UK, 2019.
- Foladori, P., Andreottola, G. and Ziglio, G., Sludge Reduction Technologies in Wastewater Treatment Plants, IWA Publishing, London, UK, 2010.
- Nurdogan, Y. and Oswald, W. J., Enhanced Nutrient Removal in High-Rate Ponds, Water Sci. Technol., Vol. 31, No. 12, pp 33-43, 1995, http://doi.org/10.1016/0273-1223(95)00490-E
- Rieck, H. P., Builders: The Backbone of Powdered Detergents, in: Powdered Detergents, (Showell, M. S., ed.), pp 43–108, Marcel Dekker, New York, USA, 1988.
- Tsuzuki, Y., Koottatep, T., Jiawkok, S. and Saengpeng, S., Municipal Wastewater Characteristics in Thailand and Effects of Soft Intervention Measures in Households on Pollutant Discharge Reduction, Water Sci. Technol., Vol. 62, No. 2., pp 231-244, 2010, http://doi.org/10.2166/wst.2010.164
- Li, L., Xie, S., Zhang, H. and Wen, D., Field Experiment on Biological Contact Oxidation Process to Treat Polluted River Water in the Dianchi Lake Watershed, Front. Environ. Sci. En., Vol. 3, No. 1, pp 38-47, 2009, http://doi.org/10.1007/s11783-009-0007-6
- Hanjra, M. A., Blackwell, J., Carr, G., Zhang, F. and Jackson, T. M., Wastewater Irrigation and Environmental Health: Implications for Water Governance and Public Policy, Int. J. Hyg. Envir. Heal., Vol. 215, No. 3, pp 255-269, 2012, http://doi.org/10.1016/j.ijheh.2011.10.003
- Avila, C., Garfí, M. and García, J., Three-Stage Hybrid Constructed Wetland System for Wastewater Treatment and Reuse in Warm Climate Regions, Ecol. Eng., Vol. 61, Part A, pp 43-49, 2013, http://doi.org/10.1016/j.ecoleng.2013.09.048
- von Sperling, M., Modelling of Coliform Removal in 186 Facultative and Maturation Ponds Around the World, Water Res., Vol. 39, No. 20, pp 5261-5273, 2005, http://doi.org/10.1016/j.watres.2005.10.016
- Verbyla, E. M., Iriarte, M. M., Mercado Guzmán, A., Coronado, O., Almanza, M., and Mihelcic, J. R., Pathogens and Fecal Indicators in Waste Stabilization Pond Systems with Direct Reuse for Irrigation: Fate and Transport in Water, Soil and Crops, Sci. Total Environ., Vol. 551-552, pp 429-437, 2016, http://doi.org/10.1016/j.scitotenv.2016.01.159
- Verbyla, E. M. and Mihelcic, J. R., A Review of Virus Removal in Wastewater Treatment Pond Systems, Water Res., Vol. 71, pp 107-124, 2015, http://doi.org/10.1016/j.watres.2014.12.031
- Sharma, A. and Ashwath, N., Land Disposal of Municipal Effluents: Importance of Choosing Agroforestry Systems, Desalination, Vol. 187, No. 1, pp 361-374, 2006, http://doi.org/10.1016/j.desal.2005.04.094
- Gogate, M. G., Farooqui, U. M. and Joshi, V. S., Sewage Water as Potential for the Tree Growth - A Study on Teak (Tectona Grandis) Plantation, Indian Forest., Vol. 121, No. 6, pp 472-481, 1995.
- Ali, H. M., El-Mahrouk, E. L. S. M., Hassan, F. A. and El-Tarawy, M. A., Usage of Sewage Effluent in Irrigation of Some Woody Tree Seedlings. Part 3: Swietenia Mahagoni (L.) Jacq., Saudi J. Biol. Sci., Vol. 18, No. 2, pp 201-207, 2011, http://doi.org/10.1016/j.sjbs.2010.08.001